INTRODUCTION
The proportion of the world’s population over the age of 60 years doubled in the last century and will increase two-to threefold in the first century of this millennium1. This population has a higher prevalence of chronic illnesses including cardiovascular diseases, cancers, osteoporosis, diabetes, Parkinson’s disease, dementia and many other conditions. An increasing body of research is adding further prescribing indications for diseases that occur in the elderly population. This, along with the increasing size of the population, means that the numbers of prescriptions for elderly patients are increasing. Depending on the age group between 60% and 80% of elderly people are taking medication and between 20% and 30% of these patients are taking at least three drugs2. It is also estimated that although those over 75 years account for 14% of the population, they receive 33% of medication prescribed3.
An appreciation of the physiology of the ageing process and how it affects an individual’s response to, and handling of, drugs (and drug combinations) is essential to ensure safe prescribing for this vulnerable population4. The ageing process can be described as the consequences of the sum of local effects at the cellular and molecular level. A feature of cellular ageing is the disruption of regulatory processes between cells and organs with consequent failure to maintain homeostasis. There is also time-related loss of functional units (the smallest structures still capable of performing the specific physiological activity characteristic to the organ involved) such as nephrons and neurons5. These age-related physiological changes affect all organ systems in the body, they significantly affect how the ageing body handles and responds to pharmacological agents and hence need to be considered when prescribing. Chronic illness in older age and related organ dysfunction can compound these ageing-associated changes.
In this chapter we describe the age-associated changes in physiology and consequent changes in pharmacokinetics and pharmacody-namics and their implications for prescribing in elderly patients.
PHARMACOKINETICS AND THE AGEING PROCESS
Pharmacokinetics is the description of how the body handles drugs after administration. It incorporates the liberation, absorption, distribution, metabolism and excretion of drugs and their metabolites. These processes may be affected by the physiological changes associated with ageing resulting in changes in the pharmacokinetics of drugs.
Absorption
Following administration, a number of factors affect a drug’s entry into the circulation. These include properties such as particle size, molecular weight, charge, solubility and pKa (the pH at which 50% remains in an un-ionized, lipid-soluble state). Most drugs are weak acids or bases and are present in solution as both ionized and nonionized species. Non-ionized drugs are lipid soluble and diffuse easily across the cell membrane. Following liberation some absorption may take place in the stomach, depending on the pKa of the drug and the pH of the stomach. Gastric acid secretion decreases with normal ageing, confounded by the widespread use of proton pump inhibitors and, to a lesser extent, H2 receptor antagonists, as well as eradication of Helicobacter pylori5. Drugs requiring an acidic environment to become ionized will be affected most (e.g. iron compounds). However, for most drugs the large surface area of the small bowel makes it the main site of drug absorption. With ageing, although many drugs show no change in absorption, there is slightly reduced small bowel absorption of some substances (including iron, calcium and sugar). There is slower colonic transit time with age, and an associated decrease in peristalsis, largely due a loss of neurons involved in control of the gastrointestinal tract1. Passive intestinal permeability is probably unchanged in old age for most substances. However, active transport of other agents such as vitamin B12 is impaired. The effects of these age-related changes would influence primarily those drugs with low permeability and low solubility, e.g. cephalexin. Agents such as benzodiazepines and lithium are readily absorbed. Some agents such as neuroleptics can be esterified with long-chain fatty acids to yield depot formulations that are only slowly absorbed from the site of peripheral injection, e.g. flupentixol where the elimination half-life (t1/2z) can be prolonged from 6 hours to 36 hours by use of an intramuscular depot preparation.
Distribution
The volume of distribution (V), also known as apparent volume of distribution, is a pharmacological term used to quantify the distribution of a medication between plasma and the rest of the body after oral or parenteral dosing. It is defined as the volume in which the amount of drug would need to be uniformly distributed to produce a given plasma concentration. Put another way it refers to the fluid volume that would be required to contain all of the drug in the body at the same concentration as that in the blood or plasma. A drug with a high V (e.g. morphine -300 litres) implies extensive distribution outside the blood or plasma to other tissues such as fat or muscle. The V is dependent on lipophilicity and the ability of the drug to bind to plasma proteins such as albumin (acidic drugs) and a1-acid glycoprotein (basic drugs) thus holding drug in the blood compartment and reducing V6,7.
An initial phase of distribution reflects cardiac output and regional blood flow, to well-perfused organs (heart, liver, kidney and brain). A second phase, involving a larger fraction of the body mass, to muscle, skin, viscera and fat can take several minutes to hours to reach steady state. Drugs accumulating in a given tissue, such as fat, may serve as a reservoir that prolongs drug action in that same tissue or at a distant site7.
With ageing there is a decrease in lean body weight, muscle mass and body water and an increase in body fat per kg of body weight. As a result, lipid-soluble drugs such as benzodiazepines, morphine, neuroleptics and amitriptyline have an increased V due to the higher proportion of body fat. With ageing the higher V for such lipidsoluble drugs, (along with reduced clearance) will result in prolonged elimination half-life and hence drug effects. For water-soluble drugs, V will fall hence higher concentrations will be reached after initial administration. Loading doses of water-soluble drugs should, therefore, be reduced in elderly patients unless the drug also binds to muscle such as digoxin when the muscle mass would be a relevant consideration.
Protein Binding
Many drugs are protein bound to a varying degree. Bound drugs are inactive. An unbound drug is free to mediate its effect. The binding is usually reversible. Most acidic drugs (diazepam, phenytoin, warfarin) bind to albumin. Basic drugs such as lidocaine and tricyclic antidepressants bind to a1-acid glycoprotein. With healthy ageing there is no substantial change in plasma proteins, however, intercurrent illness can result in a drop in albumin and an increase in a1-acid glycoprotein (an acute phase protein)5. Chronic disease tends to accelerate the age-related decline in serum albumin. This can produce clinically significant increases in the free fraction of very heavily protein-bound drugs such as ibuprofen (99.5% bound)5. Other highly protein-bound drugs include benzodiazepines (>90%) and many antipsychotics (>90%). Lithium, however, is not protein bound and is excreted unchanged rather than being metabolized.
Clearance – Hepatic
Hepatic metabolism of drugs is dependent on the ability of the liver to extract drugs from the blood passing through the organ. Lipophilic drugs are metabolized into more hydrophilic compounds to facilitate elimination, particularly through the urine. However, in some cases metabolites are biologically active or even toxic. Thus risperidone is metabolized to an active metabolite (9-OH risperidone) which has a t1/2z of 22 hours versus the parent drug t1/2z of 4 hours. Similarly, diazepam is metabolized to an active metabolite (desmethyl diazepam) and amitriptyline is metabolized to nortriptyline. Both active metabolites have a longer t1/2z than the respective parent drugs. In the liver, phase I metabolism introduces a functional group onto the parent compound, generally resulting in loss of pharmacological activity. Phase I reactions are located primarily on the endoplasmic reticulum and are performed largely through the cytochrome P450 system of enzymes. This family of enzymes oxidize, hydroxylate or hydrolyse the drug substrate6,7. Several studies have shown an age-related decline in the clearance of drugs by phase I metabolism, probably reflecting a reduced hepatic mass (and, for high clearance drugs, blood flow), as enzyme activity is preserved5. Induction or increased synthesis of the cytochrome P450 enzymes induced by drugs, such as phenytoin, isoniazid, glucocorticoids and alcohol, may decrease the bioavailability of parent drug compounds.
Inhibition of drug biotransformation enzymes, commonly by depletion of necessary co-factors, results in elevated levels of parent drugs. This can lead to increased pharmacological effects and an increased incidence of drug-induced toxicity. Inhibition of different isoforms of cytochrome P450 enzymes can be seen with erythromycin and ketoconazole (CYP450 3A4); SSRIs (CYP450 2D6). For two of these enzymes, CYP4502D6 and CYP4502C19, genetic polymorphisms exist that lead to poorly functioning enzymes causing individuals to be poor metabolizers. Thus when prescribing drugs metabolized by these enzymes where the therapeutic window is narrow, prescribing should be on the basis the patient is a poor metabolizer. For example when prescribing haloperidol starting doses should be those appropriate to poor CYP2D6 metabolizers.
Many pharmacokinetic drug interactions occur at the level of hepatic metabolism. For example the inhibition of clozapine and chlorpromazine metabolism by cimetidine.
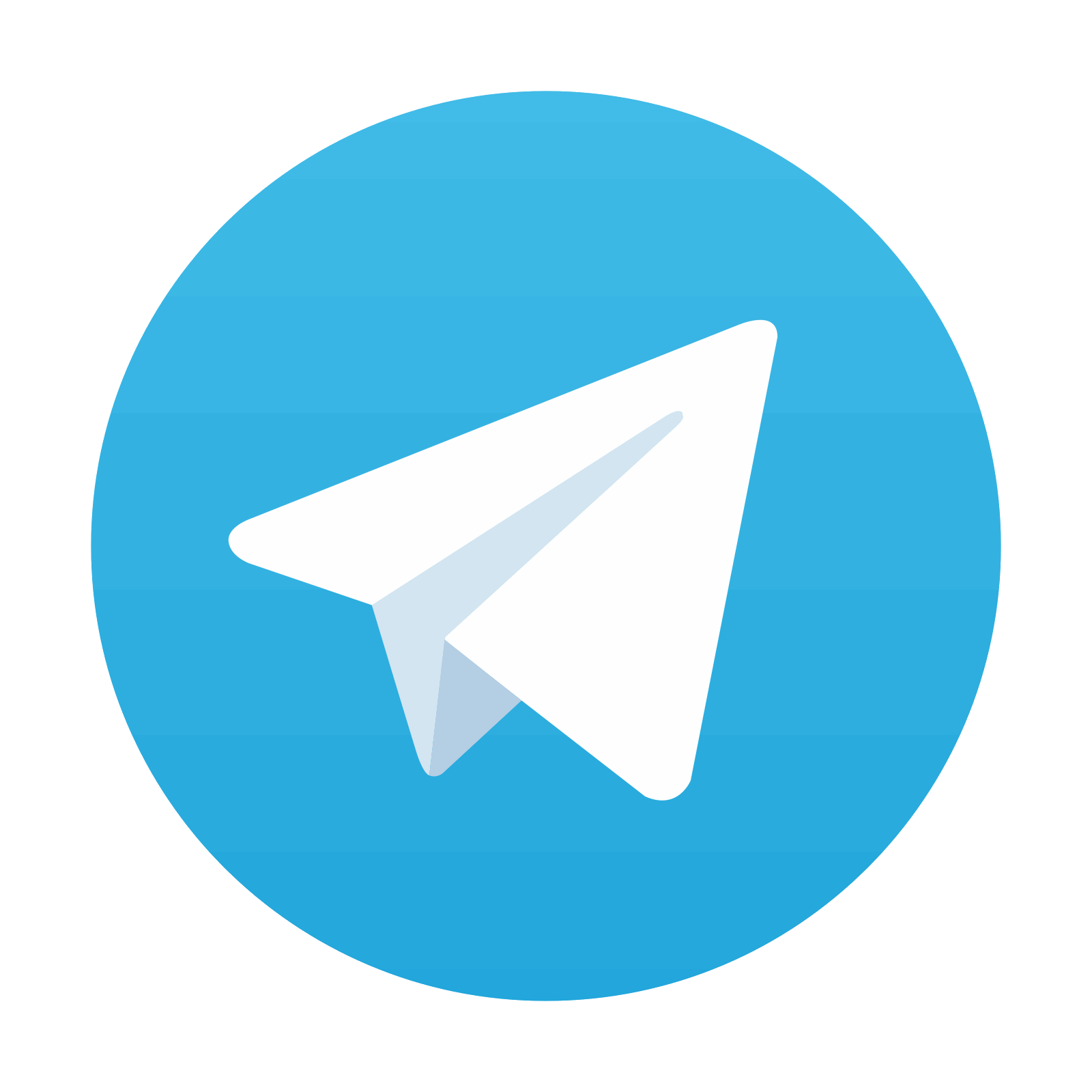
Stay updated, free articles. Join our Telegram channel

Full access? Get Clinical Tree
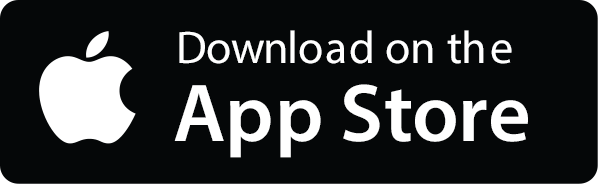
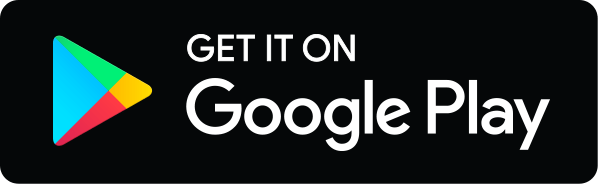