Introduction
For many decades there has been an ongoing discussion about the effect that seizures have on the brain, and, although there is a rapidly expanding body of knowledge on this topic, the answers may be only partially clearer. In this chapter we review some of these data and try to draw some conclusions from as-yet-incomplete information on the overall effect of seizures on the brain. As we will show, this issue is quite complex. The clinical answer we are looking for is how repeated seizures affect the patient with epilepsy. Most of the laboratory reports over the years examining that issue have studied the effects that induced seizures have on normal brains, and a number of interesting observations have been made, many of which are outlined in this chapter. Many of the observations from these models have been equated with epilepsy or the process of becoming epileptic. However, with the availability of an increasing number of animal models of epilepsy that have spontaneous seizures and structural changes that have significant similarities to human epilepsy, we are finding that the changes associated with epilepsy are not necessarily the same as the changes that have resulted from repeated seizures in normal brains.
These observations complicate the basic question of what is the effect of seizures on the brain, and now emphasize the need to differentiate the cause from the consequence of epilepsy. To make the interpretation of the new findings more difficult, some suggest that some of the changes found in these models could represent an attempt of the system to return everything to normal, a sort of counterbalance to the changes that are interpreted as epileptogenic or pro-seizure. For this reason it is important to understand the circuitry of the seizures and place the many changes that are found throughout the circuit in the context of the overall effect these changes have on circuit function. Our understanding of the changes and the circuitry of seizure initiation and spread is not yet to the point at which we can determine the effect of the observed changes in promoting or potentially inhibiting the generation and spread of seizure activity, but we will try to place the findings into context wherever possible.
In the course of this chapter we look at three different types of seizures that either have distinctly different consequences or clinical relevance. Although we try to make the distinctions clear wherever we discuss the different models, we highlight the distinctions among the different groups here. The first group includes intermittent seizures that are induced in normal brains. Perhaps the most common model for the study of this issue is kindling, which usually involves the focal induction of a seizure through an electrical stimulation via an implanted electrode. The number of seizures can be controlled, so that the cumulative effect of the seizures can be quantified in light of an exact number of seizures. The second group comprises the models that have epilepsy. Because we do not cover absence or other generalized epilepsies, most of the models that we discuss have some form of pathologic change that contribute to the epileptic condition. For this reason it will be difficult to differentiate which changes contribute to the epilepsy from those that may be the consequence. The third group is status epilepticus. There is no question that these prolonged seizures cause brain damage and chronic changes, including, in many cases, epilepsy. Status epilepticus is not really the focus of this chapter; rather, it is used as a tool for creating animals with epilepsy, so in this chapter it is discussed primarily in that light. In the course of this chapter we review the data that are available, including the effect of repeated seizures to increase the likelihood of having another seizure, the potential result of seizures on brain structure and on behavioral function, the potential of seizures to alter neurogenesis, and the consequences of seizures on receptors and channels and, as a secondary consequence, on the pharmacology. We also review the current state of knowledge on synaptic reorganization associated with seizures and epilepsy. As will become clear, not all seizures are created equal, nor do they affect the brain equally.
Seizures Beget Seizures?
There has been a long-standing concept that the occurrence of a seizure prepares the way for the next, so that each subsequent seizure induces changes that further enhance the odds of having another.55 To some this hypothesized process represents epileptogenesis. But what is the evidence for seizures truly begetting seizures as opposed to each subsequent recurrence of a seizure representing a symptom of an independent underlying process that is causing the seizure? In this scenario the recurrences are simply a symptom of the abnormalities that are the true source for the seizures. Although the varying natural histories of different epilepsy syndromes support the concept that seizures do not necessarily beget seizures, there are experimental data on both sides of the discussion. How do the findings apply to human epilepsy?
Before we enter into a discussion of the many observations related to the topic we should make clear what is meant by epileptogenesis because it has taken on several connotations in the epilepsy literature. A commonly used definition is that epileptogenesis is the process by which seizures gradually lengthen and behaviorally intensify with each recurrence. A common example is the kindling model, in which each repeated stimulated seizure results in a lengthening of the seizure duration and the behavioral intensity until a plateau is reached.50,109 The other definition for epileptogenesis is the process that leads to the first spontaneous seizure after the appearance of a potentially seizure-causing abnormality, such as a traumatic lesion, a malformation, or a tumor. Whether it’s through the interruption of critical neuronal pathways, the loss of particular neuronal populations, or the secretion of a proexcitatory substance (sometimes casually referred to as epileptogenic goo),
a series of structural, pharmacologic, and physiologic changes evolve during a clinically silent time known as the latent period to the point of having a seizure.49 Although both definitions have clinical relevance, understanding the mechanisms behind the changes that take place in a brain that ultimately lead to the first spontaneous seizure will likely provide us a greater understanding of the basis for the different epilepsy syndromes.
a series of structural, pharmacologic, and physiologic changes evolve during a clinically silent time known as the latent period to the point of having a seizure.49 Although both definitions have clinical relevance, understanding the mechanisms behind the changes that take place in a brain that ultimately lead to the first spontaneous seizure will likely provide us a greater understanding of the basis for the different epilepsy syndromes.
One can find human syndromes that either support or refute the idea that seizures beget seizures because there are a number of syndromes that remit spontaneously, regardless of the number of seizures experienced, and there are others that clearly have a worsening with regard to frequency and clinical severity. Benign epilepsy of centrotemporal spikes or rolandic epilepsy is well known to remit by the teenage years independent of the number of seizures experienced.88b On the other hand, seizures associated with the mesial temporal lobe or limbic epilepsy syndrome sometimes start with barely perceptible subjective events and gradually progress to longer seizures with loss of consciousness that become refractory to therapy.49 There is enough variation from individual to individual even within one of these syndromes to support the interpretation that the natural history of each epilepsy syndrome is the natural history of the underlying cause because each syndrome has a common course unique to it. Although one cannot completely exclude the possibility that in some patients with particular syndromes the seizures do cause seizure-enhancing changes in the critical circuits, it does not appear to be a universal phenomenon.
The kindling model is most frequently cited as evidence for seizures begetting seizures. Clearly with each repeated stimulated seizure there is a more intense seizure, and the seizure increases in duration.50,109 This observation has been made in many forms of human epilepsy as well as in some of the animal models of chronic limbic epilepsy.17,31 There are a number of additional reports that with enough stimulated seizures the kindled animals will have spontaneous seizures. This observation has been made in rats, cats, and baboons (Papio sp.).130,146,164 Some investigators have indicated that this susceptibility to developing spontaneous seizures also varies with the age of the animal at the time of kindling.58,147 However, this observation is not universal across all investigators. There are some primate species that do not fully kindle in the sense that they never show convulsions and they have never been seen to have spontaneous seizures in the course of kindling stimulation. Genetics clearly plays a role because the baboons that have spontaneous seizures observed following kindling come from lines that are known to develop epilepsy, whereas the macaques in which spontaneous seizures are not associated with kindling have not been associated with a predisposition to epilepsy.165 In rats, the issue is complicated by strain susceptibility, variations in the site of kindling stimulation, and many variable stimulation protocols. The number of stimulations required before spontaneous seizures are observed varies from about 150 to 300 or more.130,136 Others (by personal report, including one of the authors) have rarely seen spontaneous seizures, even after 1,000 or more stimulations. Although it is clear that in some labs and with some stimulation protocols, spontaneous seizures can be observed following a period of extensive kindling, it is not a universal phenomenon. The real question is whether kindling to spontaneous seizures has significant implications for human epilepsy, and here the answer is unknown. It is clear that in some patients with certain types of epilepsy the seizures can progress, but this progression happens after the onset of spontaneous seizures with an underlying associated pathology. The situation of recurrent stimulated seizures in people with an otherwise normal brain is seen only with patients that undergo electroconvulsive therapy for psychiatric disorders. Among these patients epilepsy as a consequence of the convulsive therapy is extremely rare, if it occurs at all. There is a report of a patient with 1,200 induced convulsions with no evidence for progressive brain injury or the development of epilepsy.90 There are reports of patients who do have seizures following the therapy, but the observation is clouded by the concomitant use of medications that can lower seizure threshold.91
So, can seizures beget seizures? The data suggest that in certain strains or species of animals the repeated induction of seizures using a particular stimulus protocol at particular ages can result in spontaneous seizures. Is this phenomenon universal? No. More important, is this phenomenon relevant for human epilepsy? It is not possible to provide a blanket statement for all epilepsies in all individuals, but certainly there is little to no evidence suggesting that repeated exogenously induced seizures lead to chronic epilepsy. Can seizures in an individual worsen over time? There is certainly good evidence that they can, but that is more of a kindling phenomenon in which the seizure duration increases and the recruitment of brain regions expands once the spontaneous seizures have started. Alternatively, it could be a symptom of a worsening of the underlying condition that is causing the epilepsy. In some of the animal models of limbic epilepsy there is good evidence for a worsening over time until a plateau is achieved, after which a relatively stable pattern is maintained.17,51 However, in some animals, as in some people, the seizures remain fairly stable for prolonged periods of time. In most cases the seizures are “begotten” by an underlying pathology, and although some can clearly progress over time with repeated seizures, other syndromes can spontaneously go into remission, an observation that suggests that the evolution of the disorder depends on the underlying etiology and less on the recurrence of seizures.
As a final observation regarding seizures and epileptogenesis, there has long been a desire to prevent the development of epilepsy or to cut the disease’s natural history short by means of some early intervention, and the search for an effective prophylaxis has been going on for years. The most common approaches have been to treat patients with antiepileptic drugs following an event, such as head trauma, that has a significant risk for subsequent epilepsy. To date, none of these approaches has been effective in reducing the subsequent risk of epilepsy, although immediate postinjury treatment does block the acute seizures in the first week.157 In animal work these attempts have been divided along two lines: (a) attempts to slow or prevent the kindling process by pretreatment before stimulation and (b) attempts to prevent the development of epilepsy following status epilepticus. The former approach, the inhibition of kindling, has been most frequently studied, and there are a number of compounds with varying, and in some cases unknown, mechanisms of action that have slowed the process (which means increased the number of stimulations necessary to achieve fully kindled seizures).108,140,149 It may be, however, that the treatments were successful at suppressing seizures but not really altering the kindling process, because as soon as the pretreatment was stopped, the animals were often at the same stage as the untreated controls. Although there are some reports that drugs such as levetiracetam, when used prophylactically during the kindling process, result in a permanent reduction in seizure duration, this phenomenon has not been regularly seen.92 Ultimately one is left with the conclusion that treatment in kindling may slow the process slightly but is unable to prevent it.
With the more recent appearance of the post–status epilepticus limbic epilepsy models (kainic acid, pilocarpine, limbic electrical stimulation), there has been an opportunity to examine possible treatments for preventing the development of chronic epilepsy. A major problem for these studies in rats has been the maintenance of potentially effective levels throughout the treatment phase, which could last several weeks or more. In
the studies examining the prevention of kindling, the animals can be given the drug at a standard time before the stimulation, which for these studies is usually once daily. Maintaining therapeutic levels over a 24-hour period is not an issue in the kindling studies. Following status epilepticus, however, the latent period before the first seizure is usually several weeks to a month, and for most drugs, maintaining levels during that time is extremely difficult. There have been a few studies using antimetabolites that have reported some success in preventing anatomic features of chronic epilepsy without preventing the epilepsy.89,168 The only studies that have been successful in preventing the development of epilepsy have been those that used treatments during the early stages of status epilepticus, usually during the first several hours, and the likely reason for success is that the intervention came early enough to stop the seizures and prevent the damage that the status epilepticus causes if left untreated.131 Inasmuch as it is the damage that is thought to lead to the epilepsy, these interventions are likely successful because they prevent the cause rather than preventing the epilepsy once the cause has happened. Prophylaxis against the development of epilepsy following a potentially epileptogenic injury remains to be discovered.
the studies examining the prevention of kindling, the animals can be given the drug at a standard time before the stimulation, which for these studies is usually once daily. Maintaining therapeutic levels over a 24-hour period is not an issue in the kindling studies. Following status epilepticus, however, the latent period before the first seizure is usually several weeks to a month, and for most drugs, maintaining levels during that time is extremely difficult. There have been a few studies using antimetabolites that have reported some success in preventing anatomic features of chronic epilepsy without preventing the epilepsy.89,168 The only studies that have been successful in preventing the development of epilepsy have been those that used treatments during the early stages of status epilepticus, usually during the first several hours, and the likely reason for success is that the intervention came early enough to stop the seizures and prevent the damage that the status epilepticus causes if left untreated.131 Inasmuch as it is the damage that is thought to lead to the epilepsy, these interventions are likely successful because they prevent the cause rather than preventing the epilepsy once the cause has happened. Prophylaxis against the development of epilepsy following a potentially epileptogenic injury remains to be discovered.
Seizures and Brain Structure
Perhaps one of the greatest concerns in epilepsy is the question of whether repeated seizures damage the brain. The answers are confused by the mixing of different models, methods of documentation, and types of seizures. Brain damage, as evidenced by neuronal loss, gliosis, and focal atrophy, has been associated with epilepsy for as long as people have been studying the issue. As noted, the issue that is of greatest concern is which of these changes preceded the onset of the seizures and which appeared afterward, that is, what is the cause of epilepsy and what is the consequence. But there are other questions as well. Do seizures alter the structure of neurons in a way that alters their physiology? What effect do seizures have on glial cells? Do the type of seizure and the temporal patterning of the repeated seizures have different effects on the anatomy? What is the potential consequence of seizure-induced changes? Are the changes harmful or are they a protective reaction to the seizures? In this and several subsequent sections dealing with genes, channels, and neurogenesis we address these issues as best we can, given the nature of the data.
There is no question that some seizures affect the structure of the brain. There is almost universal agreement on this point. The issues are which seizures and what do they do to the brain. Absence seizures, which are brief, have a slow discharge pattern and use intrinsic brain rhythms, and they are generally considered not to have a significant effect on brain structure. At the other extreme, status epilepticus, and usually the status epilepticus associated with a progression of electroencephalographic (EEG) frequencies from continuous high-frequency activity to periodic epileptiform discharges, is unquestionably associated with significant neuronal loss and gliosis. The controversy lies in whether occasional seizures that have a tonic–clonic pattern on EEG lasting for up to several minutes at a time induce neuronal loss. Furthermore, if damage does occur, does it ever plateau after a subset of vulnerable neurons is lost or does it continue to progress with each successive seizure? Clinical information is difficult to interpret in large part because it is rare to know what the structure of the brain was like before the onset of the seizures because much of the available tissue only becomes available after it has been removed surgically because of intractable epilepsy. There are a number of reports showing that patients who have more frequent or more intense seizures have more severe pathology/cell loss. Although this observation has often been interpreted to mean that more seizures or more intense seizures cause the greatest damage, this interpretation is based on the assumption that all individuals start from the same point and progress differently depending on the nature of the seizure disorder. An equally valid and more conservative interpretation is that the more severe pathology results in a worse seizure disorder. However, it is an issue that really cannot be resolved by clinical studies unless we begin collecting data well before the onset of epilepsy and watch for progression before and after the seizures begin. To study the issue under a controlled setting we have to use animal models of epilepsy.
There are several questions to answer in examining the issue of repeated seizures and neuronal loss. First, does it happen at all? Second, following the induction of an epileptogenic lesion, do the repeated spontaneous seizures cause additional loss? Two models have been used to study these questions. First, the kindling model allows us to start with a naive brain and induce seizures with an implanted electrode. We can control the number and frequency of seizures as well as the age of the animal before examining brain structure for change. The status epilepticus models result in a relatively stereotyped, but still somewhat variable, pattern of neuronal loss and gliosis. These rats then develop chronic epilepsy over a period of some weeks. The pattern of neuronal loss approximates mesial temporal sclerosis, and this model allows us to determine whether recurrent seizures on a background of preexisting pathology cause additional neuronal loss. These models of post–status epilepticus limbic epilepsy are frequently compared to their human paradigm, and there are many points of similarity. One issue is not, and that is the origin of the pathology in the limbic structures. Although patients with limbic epilepsy frequently have an episode of a prolonged seizure (usually febrile) well before the onset of the seizures that are recognized as part of the symptoms of the chronic syndrome that first appear, the majority do not have a well-identified initial (and often considered precipitating) event.12,33 Although the prolonged seizures can clearly induce neuronal loss, for those patients without a well-defined first event, the origin of the abnormality is less clear. It is possible that the precipitating event was unrecognized but still caused damage. There is some evidence to suggest that the abnormalities were present at birth because there has been frequent association with cortical dysplasias, and there is increasing evidence that genetic factors may also play a role as well.25,87 It is also possible that the changes are the result of repeated seizures. Although the final answers are not in yet and the issue has supporters on both sides of the argument, there are a number of experimental results that are pointing in the direction of minimal loss as a result of repeated seizures.
The presence of neuronal loss is not controversial in limbic epilepsy. The questions are how it got there, and, more important, if is it progressive. It has been noted that there is considerable variability in the distribution of the pathology across patients.102 As noted earlier, there have been a number of reports, especially quantitative magnetic resonance imaging (MRI) studies comparing volume loss and seizure severity, and the investigators have reported a relation. Some studies, however, have concluded that repeated seizures are not the cause of hippocampal atrophy seen on MRI scans.23,24 A recent histologic study examined the relationship between the duration of the epilepsy (up to 30 years) and the severity of neuronal loss.106 The authors found that there was a slight decrease in neuronal density over the years and that this loss was significant (see later discussion regarding the interpretation of density changes), but that there was considerable loss even among patients with a relatively short disease duration. The difference between control hippocampal densities and the neuronal
density in epileptic patients was much greater than that between epileptic patients with relatively short duration and those with the longest duration. This observation suggests that most of the neuronal loss occurs either before the onset of the epilepsy or very shortly thereafter.
density in epileptic patients was much greater than that between epileptic patients with relatively short duration and those with the longest duration. This observation suggests that most of the neuronal loss occurs either before the onset of the epilepsy or very shortly thereafter.
There has been another interesting observation with regard to seizures and brain damage. There are reports that animals with prior seizures, whether kindled or electroconvulsive, have less neuronal loss when subsequently subjected to insults that usually result in significant brain damage. This has been reported for animals that had been subjected to status epilepticus, ischemia, or adrenalectomy.74,79,104 The duration of the protective effect is likely transient, first coming into effect about 24 hours after the “protective” seizure and lasting at least 1 or 2 weeks. The mechanism is unclear but almost certainly involves the upregulation of factors that interfere with the apoptotic cascade.
Kindling has the distinct advantage in examining the question of seizure-induced neuronal loss because intervals between seizures and the number of seizures will be controlled as desired by the experimenter. It delivers a focal electrical stimulation to the desired part of the brain, and the stimulations can follow any pattern. There have been a number of kindling-based studies, and they are generally in agreement that repeated seizures result in lower neuronal densities. There are, however, quite different opinions regarding the significance of the reduced neuronal densities and the greater reduced densities with increasing numbers of seizures. On one side of the discussion the conclusion is that the reduced density is evidence for progressive seizure-induced neuronal loss.32 The other side of the discussion holds that the reduced density is the result of seizure-induced changes in the neuropil (e.g., increased glial activation), and that once one corrects for the expanded neuropil, there is little or no change in the absolute numbers of neurons.3,18,19
One of the anatomic hallmarks of the limbic epilepsy syndrome is mossy fiber sprouting, in which the axons of the dentate gyrus granule cells that normally fill the dentate hilus and project to CA3 in the hippocampus are found in the supragranular layer of the dentate gyrus.154,156 It is seen in human limbic epilepsy as well as in the animal models of the disorder. It is also seen in kindling, with a number of reports of progression of the sprouting with successive seizures. However, the degree of sprouting seen following kindling is usually a fraction of what is seen in epileptic animals and people.105 The primary questions surrounding this phenomenon are why it occurs and what function it serves. The mechanisms underlying the process of sprouting are clearly unknown, and it is quite likely that the mechanisms associated with sprouting in kindling are quite different than those following status epilepticus. There is some evidence that in epilepsy the degree of sprouting is linked to the severity of neuronal loss in the hilus. A potential mechanism for the initiation of the sprouting is the loss of synapses and the creation of openings for new synapses. There is some evidence that neurotrophins may also be involved in bringing the aberrant axons into the molecular layer.64 In kindling, with a less severe level of sprouting, the presence or absence of hilar neuronal loss is under-debated, so the mechanisms for the process are less clear. However, in relative terms, the degree of sprouting is much less in kindled animals.
More important than knowing the mechanism underlying the process of sprouting is the physiologic or functional consequence of the new synapses, and there are a number of hypotheses and some data that address the issue. The issue centers on the question of whether the sprouting is part of an overall process in the granule cell that is net excitatory, net inhibitory, or part of a zero-sum process in which the final effect is that all the changes balance out to no change in overall function. What many of the studies show is that the overall response to perforant path stimulation is not profoundly different from the naive nonepileptic state.133 The data are somewhat conflicting in that there are varying shifts in the evoked responses in the granule cells, with some showing a slight increase in inhibition in paired stimulation and some a decrease. Overall, however, the highly epileptiform evoked responses that are seen in other brain regions are not seen in the dentate gyrus either following kindling or in the epileptic animals. However, there is evidence that these fibers form recurrent excitatory autosynapses. In addition, the granule cells appear to be more sensitive to the local application of glutamate and its analogs, with a heightened excitatory response.170 As discussed later, changes have been reported in the γ-aminobutyric acid (GABA)–mediated pharmacology, and, with regard to seizure-associated neurogenesis, there are multiple new dentate granule cells generated following status epilepticus. These new cells may contribute to the sprouting, although there are data that show that sprouting occurs even when the generation of new granule cells is prevented.126
Seizures and Behavior
On the clinical side of epilepsy, one of the major questions remains whether seizures have an effect on overall cognitive function or behavior. Epilepsy has long been associated with a number of problems in these areas. Although the overwhelming majority of patients with epilepsy have normal cognitive and behavioral profiles on formal testing, as a group they do not function quite as high as their nonepileptic counterparts. In seeking the reason behind this apparent overall lower performance, researchers have offered a number of plausible explanations, including medications, underlying preexisting abnormalities in the brain, depression that affects a high percentage of patients with uncontrolled seizures, and the consequences of the seizures themselves. In the clinical setting it is not really possible to separate all of the factors, but there is clear evidence that medications can play a role in some (but not necessarily all or even a majority of the patients), and the identified pathology often corresponds to particular neuropsychological deficits that a patient may have. However, these correlations are difficult to separate and also limit our ability to answer the question of whether the seizures themselves are playing a harmful role.
To determine whether seizures can have a neurobiologic effect on performance long term, we have to turn to animal models because we can use them to focus on the question of seizures alone, independent of the issues of precipitating pathology, affect, or medications. The two most frequently used animal models in behavioral studies have been the post–status epilepticus model with chronic limbic epilepsy and the kindling model. The post–status epilepticus model is associated with significant neuronal loss, and behavioral testing, performed when the animals are in the chronic phase with spontaneous limbic seizures, have consistently revealed that there are overt abnormalities in a number of behavioral areas. However, just as with patients with epilepsy, one is dealing with the confounding issues of underlying brain pathology, seizures, and, frequently, the potential confounding effect of a postictal state.
Table 1 Factors that modulate adult hippocampal neurogenesis | ||||||||||||||||||||||||||||||||||||||||||||||||||||||||||||||||||||||||||||||||||||
---|---|---|---|---|---|---|---|---|---|---|---|---|---|---|---|---|---|---|---|---|---|---|---|---|---|---|---|---|---|---|---|---|---|---|---|---|---|---|---|---|---|---|---|---|---|---|---|---|---|---|---|---|---|---|---|---|---|---|---|---|---|---|---|---|---|---|---|---|---|---|---|---|---|---|---|---|---|---|---|---|---|---|---|---|
|
It is not possible to isolate the potential effects of seizures from the neuropathology in this situation, and for this reason the kindling model may be more appropriate because the seizures can be induced in any desired pattern, at almost any age, and, although this is a controversial issue, without the overt pathology that is found in the limbic epilepsy model. Although not absolutely perfect, it may be considered a purer model for studying the effects of seizures on behavior and memory because there is much less, if any, associated neuropathology. As can be expected, the results from the kindling model are
complex. Although there is no question that kindling can have a negative impact on spatial memory,86 other studies suggest that the interactions may be more complex, with the overall effect depending on stimulation site, emotional state, and stimulation paradigm.2,11 It is also likely that intermittent brief seizures that occur during development have an impact, although investigators point out that there may be beneficial effects as well as negative ones.162 Overall the data suggest that seizures can have a negative effect, but that the effects may be relatively mild and are likely influenced by a number of factors in addition to the seizures themselves.
complex. Although there is no question that kindling can have a negative impact on spatial memory,86 other studies suggest that the interactions may be more complex, with the overall effect depending on stimulation site, emotional state, and stimulation paradigm.2,11 It is also likely that intermittent brief seizures that occur during development have an impact, although investigators point out that there may be beneficial effects as well as negative ones.162 Overall the data suggest that seizures can have a negative effect, but that the effects may be relatively mild and are likely influenced by a number of factors in addition to the seizures themselves.
Altered Neurogenesis in Experimental Temporal Lobe Epilepsy
The dogma that neuronal production ceases after birth no longer is accepted. Evidence accumulated over the last five decades indicates that neurogenesis persists in the adult mammalian hippocampal dentate gyrus and subventricular zone (SVZ)-olfactory bulb pathway.5,6,29,39,73,81,88a Neuronal birth continues throughout life in every mammalian species examined, including human and nonhuman primates.45,53,54,80a,128 In the dentate gyrus, neural stem–like cells reside in the subgranular zone (SGZ) at the border of the hilus and dentate granule cell (DGC) layer. These cells express glial fibrillary acidic protein (GFAP) and nestin42 and proliferate to generate clusters of neuroblasts that disperse and migrate a short distance into the DGC layer, where they differentiate into granule neurons.6,29,73,81 Adult-born DGCs send axonal projections to appropriate targets in hippocampal area CA359,103,152,161 and acquire electrophysiologic characteristics of mature DGCs.47,161,166
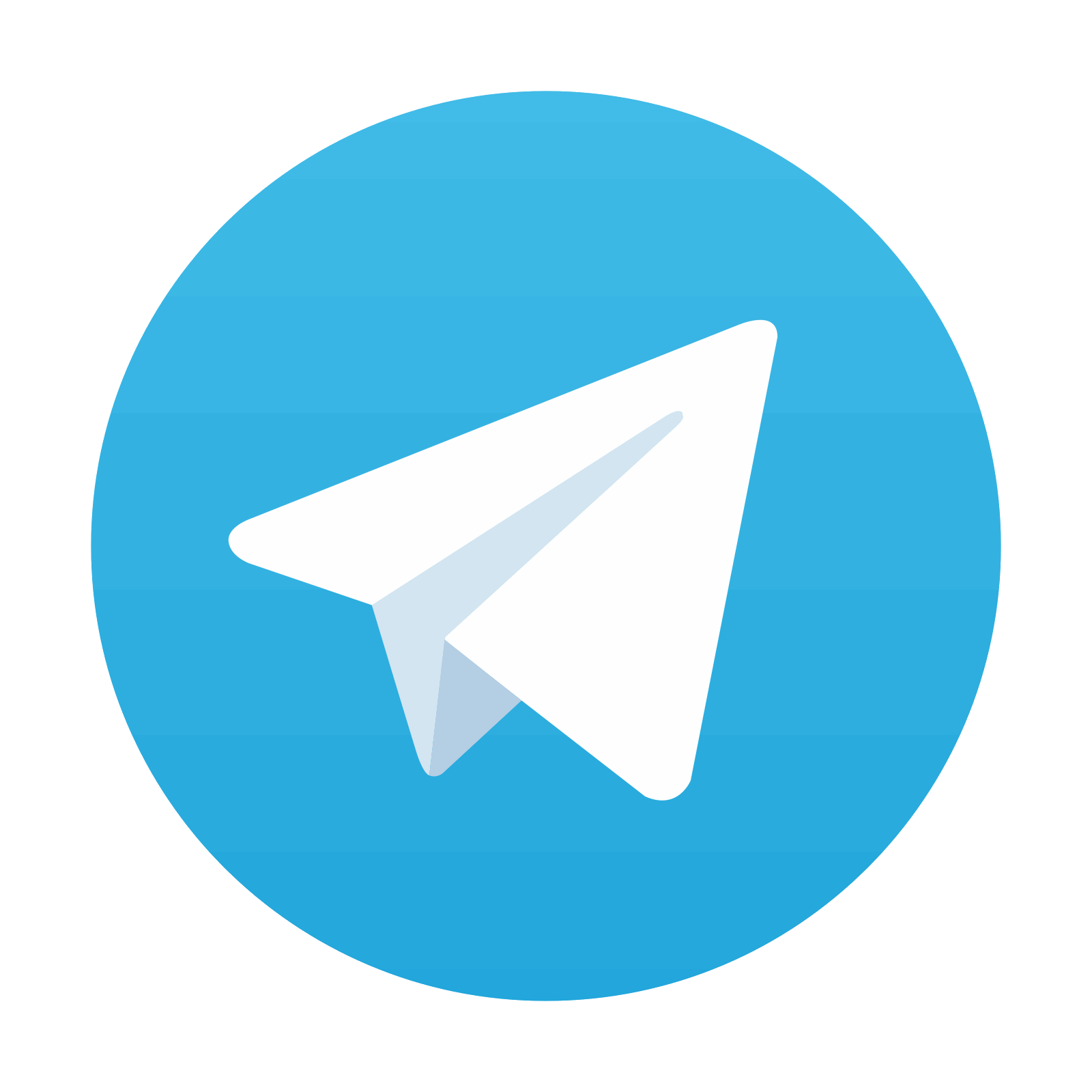
Stay updated, free articles. Join our Telegram channel

Full access? Get Clinical Tree
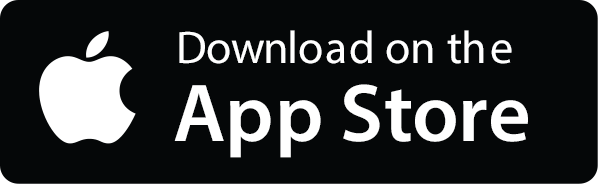
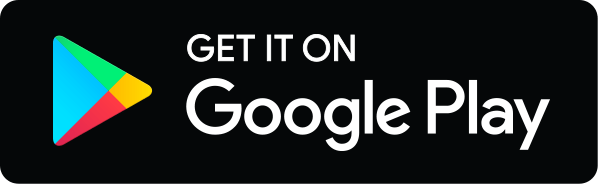