Chapter 12 The hip
Mennell (1964) describes the hip joint as ‘probably the most nearly perfect joint in the body …close to being a perfect ball-and-socket joint’. In erect bilateral stance each hip joint carries approximately one-third of the body’s weight (with the remaining one-third being found in the lower extremities), sufficient force to produce an actual bending between the femoral neck and the shaft of the femur (Lee 2004) (see also Box 12.1). One-legged standing, as well as the compounded force of hopping or landing on one leg, exaggerate these forces dramatically. It is the trabecular systems of the pelvis and femur which in particular resist this bending, shearing force (Levangie & Norkin 2005). Because of its vital role in locomotion and the interaction between the trunk and the lower extremities (especially the lumbo-pelvic-hip region), an optimal combination of osseous, joint, muscular and ligamentous function, as well as integral postural alignment, is required to maintain the hip joint in good working order.
Box 12.1 Compressive forces of the hip joint
Levangie & Norkin (2005) provide a thorough discussion of weight distribution in both bilateral and unilateral stance. They point to factors other than weight (such as torque created by the distance of the joint from the center of gravity of the body) to highlight that compression considerations are more complex than solely weight distribution. Regarding bilateral stance, they note that total hip joint compression, through each hip, should be one-third of body weight. However, they go on to report:
Bergmann and colleagues [1997] showed in several subjects with an instrumented pressure sensitive hip prosthesis that the joint compression across each hip in bilateral stance was 80% to 100% of body weight, rather than one-third (33%) of body weight, as commonly proposed. When they added a symmetrically distributed load to the subject’s trunk, the hip joint forces both hip joints increased by the full weight of the load, rather than by half of the superimposed load, as might be expected. Although the mechanics of someone standing who has a prosthetic hip may not fully represent normal hip joint forces, the findings of Bergmann and colleagues call into question the simplistic view of hip joint forces in bilateral stance.
In unilateral stance, the muscular contractions necessary for torque and countertorque add a tremendous muscular compressive force, much greater than the weight compressive force on the hip joint (Levangie & Norkin 2005).
Lee (2004) summarizes:
Dysfunction therefore requires investigation of all these elements.
Influences are multidirectional, not just downward, from the trunk to the hip. For example, in discussing ‘other’ causes of low back pain, Waddell (1998) makes the pertinent observation:
If considering broadly, as Waddell suggests, it becomes evident that the pain could also be due to hip imbalances or from more distal structures of the lower extremity. As Greenman (1996) points out: ‘Dysfunction of the lower extremity [including the hip] alters the functional capacity of the rest of the body, particularly the pelvic girdle’. This is one reason why, when assessment is performed, it is useful to carry out the process from proximal to distal, with any indication of pelvic dysfunction (for example) demanding assessment of the structures distal to it, including the hip joint, knee joint and foot complex.
• The polyaxial articulation at the ball-and-socket hip joint is made up of the head of the femur, ‘the longest and heaviest bone in the body’ (Kuchera & Goodridge 1997), and its articulation with the cup-shaped acetabulum of the innominate bone. (See Fig. 11.1 B and 11.2 B for details of the acetabulum and Figs. 13.1 A and B for details of the femur)
• The articular surfaces are curved to accommodate each other reciprocally. Gray’s anatomy (2005) suggests that evidence favors there being spheroid and slightly ovoid surfaces, which become almost spherical with advancing age.
• Apart from a rough area where the ligament of the head attaches, the femoral head is covered by articular cartilage that, anteriorly, extends laterally over part of the femoral neck.
• The acetabular articular surface is moon shaped (lunate), so forming an incomplete ring, which is broadest above (where body weight is carried when upright) and narrowest in the pubic region.
• The acetabular fossa contains fibroelastic fat, mainly covered by synovial membrane. A fibrocartilaginous acetabular labrum increases acetabular depth.
• Ligaments include the iliofemoral, ischiofemoral, pubofemoral and the ligament of the head of the femur.
• The neck of the femur inclines toward the acetabulum, from the shaft of the femur, at angles that range from less than 120° (coxa varus) to over 135° (coxa valgus), with normal lying between these extremes (i.e. 120–135°) (Fig. 12.1).
• Dislocations occur more easily in a coxa valgus hip, particularly when the femur is adducted. It will be more stable, however, in abduction of the femur (wide-based stance).
• The hip joint displays a precise convex surface (femoral head) articulating symmetrically with the concave one (acetabulum) to provide an ‘outstanding example of a congruous joint’ (Cailliet 1996).
Capsule, ligaments and membranes
The hip’s fibrous capsule
• A collar around the neck of the femur is formed internally by circular fibers, known as the zona orbicularis, which merge with the pubofemoral and ischiofemoral ligaments.
• Longitudinal fibers lie externally, particularly anterosuperiorly, where they are reinforced by the iliofemoral ligament.
• The capsule also receives support from the pubofemoral and ischiofemoral ligaments.
• The capsule is covered by a bursa that separates it from psoas major and iliacus.
• Toe-out stance directs the head of the femur forward (out of the socket). The iliofemoral ligament would be too far forward to prevent subluxation and support would then need to be derived from the iliopsoas tendon.
Iliofemoral ligament
This powerful triangular structure (commonly referred to as the Y-shaped ligament) lies anterior to the capsule with which it blends. It is a major stabilizing force for the anterior joint. The apex of the triangle attaches between the anterior inferior iliac spine and the acetabular rim, while the base of the triangle attaches to the intertrochanteric line. The ligament has been noted (Gray’s anatomy 2005) as having a less powerful central segment (greater iliofemoral ligament) lying between denser lateral and medial iliofemoral ligaments, both of which attach to the intertrochanteric line, at the superolateral and the inferomedial ends respectively (Fig. 12.2).
Ischiofemoral ligament
• A central part runs from the ischium, posteroinferiorly to the acetabulum.
• ‘The superior ischiofemoral ligament spirals superolaterally behind the femoral neck, some fibers blending with the zona orbicularis, to attach to the greater trochanter deep to the iliofemoral ligament.
• Lateral and medial inferior ischiofemoral ligaments embrace the posterior circumference of the femoral neck.’ (Gray’s anatomy 2005)
Ligamentum teres (Fig. 12.3)
This ligament, also called the ‘ligament of the head of the femur’, is a ‘triangular flat band, its apex is attached anterosuperiorly in the pit on the femoral head; its base is principally attached on both sides of the acetabular notch, between which it blends with the transverse ligament’ (Gray’s anatomy 2005). The ligament is encased by a synovial membrane, ensuring that it does not communicate with the synovial cavity of the hip joint. The ligamentum teres becomes taut when the thigh is adducted and partially flexed. It releases on abduction.
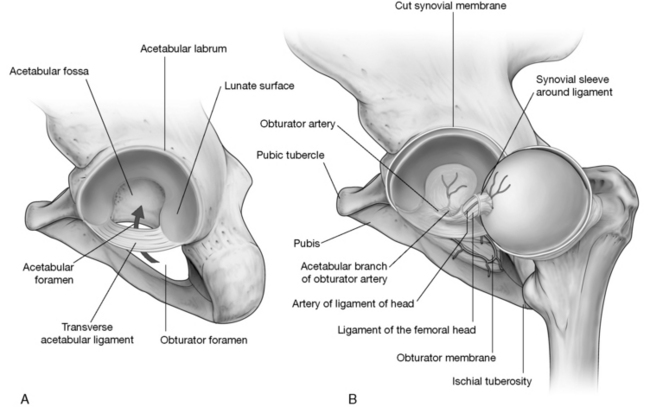
Figure 12.3 A: Acetabulum of hip joint. B: Ligamentum teres provides vascular supply to the head of the femur.
(Reproduced, with permission, from Gray’s anatomy for students, 2nd edn, 2010, Churchill Livingstone)
Kapandji (1987) notes: The ligamentum teres plays only a trivial mechanical role though it is extremely strong (breaking force equivalent to 45 kg weight [about 100 lbs]). However, it contributes to the vascular supply of the femoral head. (Kapandji’s emphasis). His illustration depicts the artery of the ligamentum teres coursing through the ligament to supply the proximal end of the femoral head. This secondary blood supply is important to both the child and the adult for different reasons. In the developing child, blood from the retinacular vessels cannot travel through the avascular cartilaginous epiphysis so the head of the femur is supplied through the artery of the ligamentum teres. For the adult this secondary supply might become especially important in preventing avascular necrosis of the femoral head if the primary supply from the retinacular vessels were injured in femoral neck fractures.
Stability
The hip joint’s design provides for excellent stability, unlike that of the shoulder, which is designed more for mobility. When comparing the two joints, it is obvious that the articular surface of the humeral head is greater than that of the glenoid cavity, with the capsule (of the shoulder) offering little restraint. In contrast, in the hip there is a closer fit of the head of the femur to the acetabulum with the labrum providing an encompassing attachment to hold it in place, thereby qualifying the hip as a true ball-and-socket joint (Kapandji 1987), with powerful ligaments providing stabilizing support anteriorly and muscles dominating the support posteriorly.
In the erect position, stability of the hip is also assisted by the interaction of ground forces and gravity. The femoral head is pressed upward by ground forces matching the weight of the body applied by the overhanging ‘roof’ of the acetabulum (Kapandji 1987). Atmospheric pressure as well as appropriate position of the femoral head will also assist in maintaining apposition of the articular surfaces.
The hip ligaments are under moderate tension when the body is in an erect posture and become more taut as the leg moves into extension. Anteriorly, significant stability derives from the ligamentous support, as Gray’s anatomy (2005) explains: The thick capsule is reinforced by three ligaments, of which the iliofemoral is the strongest, and is progressively tightened when the femur extends to the line of the trunk. The pubofemoral and ischiofemoral ligaments also tighten and, as the joint approaches close-packing, resistance to an extending torque rapidly increases. This also implies that the iliofemoral ligament prevents excessive posterior tilt of the pelvis, which constitutes extension of the hip joint.
Despite the considerable power of some of these ligamentous structures (Kuchera & Goodridge (1997) state that the iliofemoral ligament is the most powerful ligament in the body), it is the enormous muscles of the area, including gluteus maximus and the hamstrings, which dominate in providing stability for the posterior portion of the hip joint. As Kuchera & Goodridge (1997) explain:
Angles
Angle of inclination
The angle formed by the shaft and the neck of the femur is termed the angle of inclination (collodiaphysial angle). This angle in the normal adult averages about 125° (though less in women than men) and is greater in the newborn (150°) and less in the elderly (120°) (see Fig. 12.1).
A pathologically decreased angle of inclination (producing coxa vara) affects the strength and stability of the head and neck of the femur (Platzer 2004), as do the trabeculae (Fig. 12.4). The smaller the angle of inclination, the greater the shearing force on the natural ‘zone of weakness’ of the neck of the femur. This decreased angle of inclination will also affect the positioning of the knee and knee mechanics as the weight-bearing line will then run through the medial femoral condyle and produce genu varum of the knee (bow legs).
A pathological increase in the angle of inclination (producing coxa valga) will likewise affect both hip and knee function as can pelvic dysfunction (Box. 12.2). The hip will have a greater tendency to dislocate while the altered weight bearing on the knee joint will be placed primarily on the lateral condyle and result in genu valgum (knock knees) (Platzer 2004). These effects on weight bearing on the knee joint will also produce abnormal loading on the meniscus, often resulting in deterioration of the knee joint.
Box 12.2 Motions of the pelvis at the hip joint
Levangie & Norkin (2005) discuss three movements of the hip on the femur and note that regardless of which segment is moving (femur or pelvis), the range of motion of the joint remains the same.
Lateral pelvic tilt (see Fig. 12.6)
In the normal pelvis, the ASISs are in horizontal alignment. When they are not horizontally aligned, lateral tilt of the pelvis has occurred. One side of the hip is ‘hiked’ or ‘dropped’ in relation to the other whether in unilateral or bilateral stance (Fig. 12.6). These movements, involving abduction and adduction of the femur, are functionally critical in gait, where weak abductors can create a Trendelenburg sign (see pp. 400 and 426). A key element in assessing lateral tilt is to follow the crest of the non-fixed leg. Levangie & Norkin note that ‘osteokinematic descriptions reference the motion of the end of the lever farthest from the joint axis’.
Pelvic rotation
This motion of the entire pelvis around a vertical axis is best seen from above (see Fig. 3.10). This movement is most common during the single limb support in the gait cycle, although it may be seen in bilateral stance. These motions involve medial and lateral rotation of the femur and are described regarding the movement of the side of the pelvis contralateral to the fixed limb. It should be noted that the terms ‘forward rotation’ or ‘backward rotation’ of the pelvis describe this motion around the vertical axis and should be distinguished from anterior and posterior movements of the individual innominate (or pelvis) around a horizontal axis as these describe the condition noted above as anterior or posterior tilt.
The angle of torsion (also called the angle of anteversion) of the femur expresses the relationship between an axis through the femoral condyles and the axis of the head and neck of the femur (Fig. 12.5). This angle can be observed when looking down the length of the femur from above the femoral head toward the knee. With the femoral condyles lying appropriately in the frontal plane, the axis through the head and neck of the femur normally forms an angle of 10–15° with the frontal plane (although it may vary from 7° to 30°). Levangie & Norkin (2005) point out that a pathological increase of this angle (anteversion), or decrease of the angle (retroversion) as well as abnormal angles of inclination, can:
Movement potential
The potential motions of the hip joint include flexion-extension, adduction-abduction, medial and lateral rotation (which are accompanied by glide or spin), as well as circumduction (which is a compound movement resulting from a combination of these six). Gray’s anatomy (2005) implies that there are no accessory movements available, except for very slight separation when strong traction is applied.
Gray’s anatomy (2005) further suggests that ‘circumduction, [can be] conveniently considered as rotations around three orthogonal axes’. It continues:
In discussion of accessory movements available at the hip later in this chapter (pp. 404–405), it will be seen that there is no general agreement on this topic.
When the knee is in neutral (not bent), active hip flexion to 90–100° from the vertical is possible; however, active extension of the hip beyond the vertical is limited to between 10° and 20°. These movements are enhanced by modifications of the spine and pelvis and/or by flexion of the knee and associated medial or lateral rotation of the hip. Gray’s anatomy (2005) offers an example:
Lee (2004) has graphically detailed the multiple ligamentous involvements in all hip movements. For example:
Muscles producing movement (Gray’s anatomy 2005)
• Flexion of the hip with the knee extended is generally about 90° and with the knee flexed reaches about 120°. Hip flexion is produced primarily by psoas major and iliacus, assisted by rectus femoris, sartorius, pectineus, and tensor fasciae latae. The adductors, particularly adductor longus and gracilis, also take part, particularly in early flexion from a fully extended position. The anterior fibers of gluteus medius and minimus also offer weak assistance.
• Extension range is usually considered to be about 10–20° and is primarily produced by gluteus maximus and the hamstrings and assisted by posterior fibers of gluteus medius and minimus and adductor magnus.
• Abduction to about 45–50° is produced by gluteus medius and minimus, assisted by tensor fasciae latae (especially when the hip is flexed), upper fibers of gluteus maximus, sartorius, piriformis (especially when hip is flexed to 90°) and possibly other hip rotators when the hip is flexed.
• Adduction of 20–30° from neutral is performed by adductors longus, brevis and magnus, assisted by pectineus, gracilis, gluteus maximus, the hamstrings, quadratus femoris and obturators internus and externus.
• Medial rotation (with the hip joint in flexion to 90°) offers about 30–35° and is primarily produced by tensor fasciae latae and the anterior fibers of gluteus minimus and medius, although no muscle has this action as its primary function (Levangie & Norkin 2005). Gray’s anatomy (2005) informs us that: ‘Electromyographic data suggests that the adductors usually assist in medial rather than lateral rotation but this is, of course, dependent on the primary position [of the femur]’. Travell & Simons (1992) note that piriformis appears to rotate the thigh medially when the hip is fully flexed.
• Lateral rotation of 50–60° is produced by gluteus maximus, posterior fibers of gluteus medius and minimus, piriformis, obturator externus and internus, gemelli superior and inferior, quadratus femoris, portions of adductor magnus and, in some positions, sartorius.
Muscular imbalances in which postural muscles shorten and phasic muscles become inhibited, and possibly lengthen, may play a major part in the evolution of hip dysfunction, encouraging aberrant movement patterns and resulting in adaptational – and ultimately degenerative – changes in the hip, pelvic and spinal joints (Janda 1986). The issues and concepts associated with varying responses to overuse or misuse, by different categories of muscles, are discussed fully in Volume 1, Chapter 5, and are summarized in the Essential Information chapter at the start of this book.
Relations
The muscles and other structures associated with the joint capsule are as follows.
• Anteriorly, the lateral fibers of pectineus separate its most medial part from the femoral vein.
• The tendon of psoas major, with iliacus lateral to it, descends across the capsule with the femoral artery lying anterior to the tendon.
• The femoral nerve lies in a furrow between the tendon and iliacus muscle.
• The straight head of rectus femoris crosses the joint laterally together with a deep layer of the iliotibial tract, which merges with the capsule beneath the lateral border of rectus femoris.
• The head of rectus femoris contacts the capsule superomedially and gluteus minimus covers it laterally.
• Inferiorly, the lateral fibers of pectineus lie alongside the capsule, while obturator externus is located posteriorly. The tendon of obturator externus covers the lower capsule posteriorly, dividing it from quadratus femoris.
• Superior to this the obturator internus tendon and the gemelli pass close to the joint, separating it from the sciatic nerve.
• The nerve supply for quadratus femoris lies beneath the obturator internus tendon.
• Superior to this piriformis crosses the posterior surface of the joint.
Vessels and nerve supply to joint
Articular arteries are branches from the obturator, medial circumflex femoral, and superior and inferior gluteal arteries. Nerves are from the femoral or its muscular branches, the obturator, accessory obturator, the nerve to quadratus femoris and the superior gluteal nerves. (Gray’s anatomy 2005)
Assessment of the hip joint
Is the hip joint behaving normally?
Symptoms of hip joint dysfunction usually include:
• pain aggravated by walking (especially on hard surfaces)
• pain when standing for anything but a short period
• relief of pain when lying down unless lying on the painful side
• painful distribution includes low back and the hip, into the groin and toward the knee.
• a positive Patrick’s (F-AB-ER-E) sign (see p. 407)
• tenderness at the head of the femur when palpated in the groin
• restriction on internal rotation
Lewit (1985) reports that limitation of adduction at the hip might be due to spasm initiated by trigger point activity located at an attachment at the pes anserinus (knee pain may also be reported).
Arthritic hip changes are discussed later in this chapter and discussion of hip joint reconstruction surgery is to be found in Box 12.7.
Box 12.7 Total hip replacement
Gray’s anatomy (1995) states: ‘Total hip replacement has, over the last 25 years, become one of the most successful surgical operations, with over 35 000 performed annually in the United Kingdom alone’. Hip replacement is now so widespread that it is important for practitioners to be aware of the variations in surgical procedure and what is and what is not appropriate in terms of adjunctive therapeutic intervention.
Those most likely to undergo replacement are patients with osteoarthritis, rheumatoid arthritis, psoriatic arthritis, ankylosing spondylitis, avascular necrosis, trauma and tumors affecting the hip. Symptoms preceding the operation are likely to have included pain (mainly over trochanter area, groin and anterior thigh), stiffness, deformity, limb shortening and, therefore, a consequent limp. See the discussion regarding possible trigger point input to arthritic hip pain on p. 411.
Depending on the type of procedure used, surgical access to the joint can involve:
• a lateral approach in which there is dissection of tensor fasciae latae (Charnley method)
• a posterior approach involving division of the short external rotator muscles (piriformis and the gemelli)
• an anterolateral approach involving separation of the junction between gluteus medius and tensor fasciae latae. The operation scar is a useful clue as to which approach has been used and, therefore, which muscles have been most traumatized.
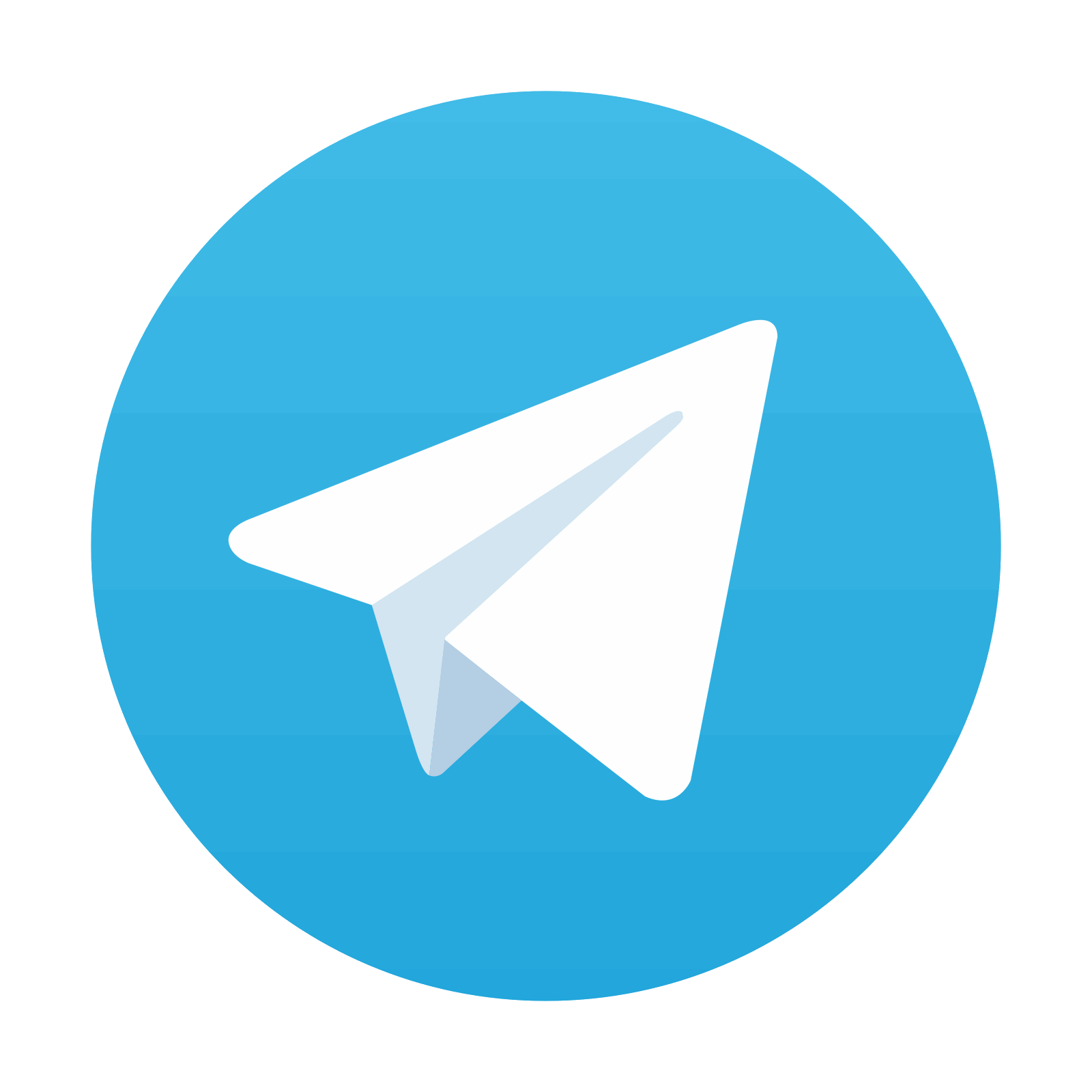
Stay updated, free articles. Join our Telegram channel

Full access? Get Clinical Tree
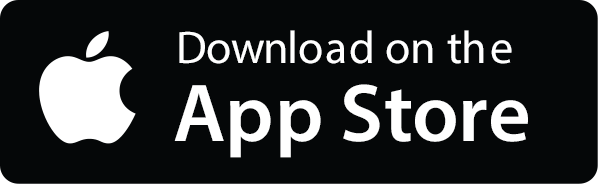
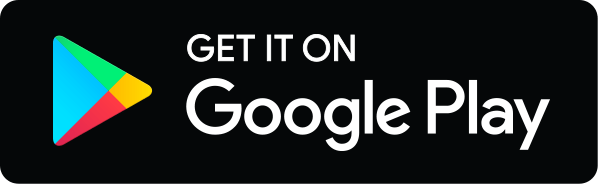
