Fig. 13.1
DWI (a) and FLAIR (b) of an acute stroke patient <3 h after symptom onset. DWI shows a hyperintensity in the left MCA territory, whereas FLAIR is without pathological findings. This is a typical patient randomized for wake up stroke in the WAKE UP trial. The FLAIR/DWI mismatch indicates an early time window <6 h
DWI is superior to CT in the diagnosis of acute ischemic stroke [15, 16]. A blinded comparison of MRI (including GRE and DWI) to CT in a broad spectrum of patients with suspected stroke provided conclusive evidence for the superior sensitivity of DWI for diagnosis of acute ischemic stroke (Fig. 13.2) [17]. The sensitivity, specificity and accuracy were 83 %, 97 %, 89 % for MRI and 17 %, 98 %, 54 % for CT, respectively for all patients. In the under 3-h subgroup, the sensitivity, specificity and accuracy were 73 %, 92 %, 81 % for MRI and 12 %, 100 %, 49 % for CT, respectively. False negative DWI were associated with mild strokes (NIHSS < 4), brainstem strokes, and early strokes (<3 h). Other studies have reported higher sensitivities for DWI and CT but these studies lacked a cohort of non-stroke controls and had samples with more severe deficits than an unselected sample presenting to emergency departments.
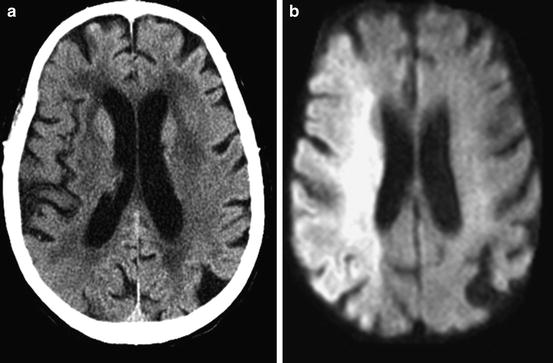
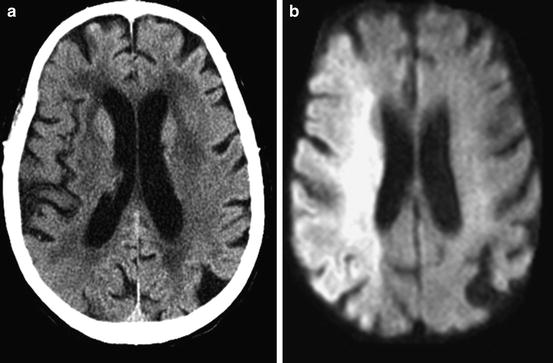
Fig. 13.2
CT (a) and DWI (b) images from a patient presenting at 2 h after onset of left hemiparesis and hemisensory loss. Ischemia within right middle cerebral artery territory is more evident on DWI image
Compared to CT, DWI is particularly useful in identifying small cortical, subcortical, or posterior fossa lesions, particularly in the acute phase. In addition, numerous studies showed that initial diffusion lesion volumes correlate well with final infarct volumes as well as neurological and functional outcomes in acute stroke patients, suggesting that diffusion MR can provide important early prognostic information [18–21].
Diffusion imaging also provides new insights into the underlying pathophysiology of transient ischemic attacks. Aggregate data from seven observational studies reported in abstract or manuscript format demonstrated that almost one-half of patients with clinical TIA syndromes have a DWI abnormality [22–28]. Although the majority of these studies suggest that the likelihood of a positive DWI increases with longer symptom duration, this relationship is not absolute. While these lesions may resolve in some cases, the vast majority of patients have imaging evidence of permanent ischemic injury [22, 29]. Based on these findings, the term “acute ischemic cerebrovascular syndrome (AICS)” may be used to describe a spectrum of clinical presentations that share a similar underlying pathophysiology: cerebral ischemia [30–32].
Diagnosis: Hemodynamic Compromise
Perfusion MR imaging (PI) allows the measurement of capillary perfusion with the dynamic susceptibility contrast-enhanced (DSC) technique. A paramagnetic contrast agent is injected as an intravenous bolus and the signal change within the brain is tracked by ultrafast MR sequences [33, 34]. The vascular hemodynamic parameters that are calculated from the MR-derived contrast-bolus-over-time-curve include mean transit time, cerebral blood volume, relative cerebral blood flow, and time to peak measures. The contrast bolus passage causes a signal loss that increases with the perfused cerebral blood volume (CBV). In ischemic brain tissue with reduced perfusion or zero perfusion, less (or no) contrast agent is present and the T2*-WI signal remains hyperintense.
It is not yet clear which PI parameter gives the optimum measure of critical hypoperfusion, nor which provides the best differentiation between infarct core and penumbra or penumbra and benign oligemia. Many researchers believe that, in clinical practice, a mean transit time (MTT) or time to peak (TTP) measures provides the best prognostic information [35, 36]. Calculation of (semi-)quantitative cerebral blood flow (CBF) requires knowledge of the arterial input function, which in clinical practice is estimated from a major artery such as the MCA or ICA. However, quantitative CBF perfusion measures have not rigorously been validated against gold standards. Another technique, not used in daily clinical practice, is arterial spin labeling which is technically more demanding and more time consuming, however may approximate real CBF values more closely [37, 38].
Automated assessments have been facilitated with various software packages one of them being the Stanford RAPID solution, not yet commercially available [39, 40]. A widespread investigator independent software solution for calculating DWI lesion sizes and PI parameter maps and respective volumes offhand remains to be implemented.
Diagnosis: Hemorrhage
While hemorrhage imaging still is and always has been a domain of CT, MRI can track components of blood hyperacutely. The appearance of intracerebral hemorrhage (ICH) depends on several factors such as the MRI sequence, field strength, and the oxygen saturation of hemoglobin and its degradation. Other factors are protein concentration, hydration, form and size of red blood cells, hematocrit, clot retraction and clot structure. In the very first minutes after symptom onset, the center of the lesion appears heterogeneous on all images (T2-WI, T2*WI, T1-WI), due to a local predominance of oxyhemoglobin. On GRE or SWI, the periphery of the hematoma appears as a hypointense rim (Fig. 13.3). Over time, this hypointensity expands into the core of the hematoma due to a progressive centripetal increase in concentration of deoxyhemoglobin. There is often a surrounding rim, which appears hyperintense on T2-WI and T2*-WI but hypointense on T1-WI and represents perifocal vasogenic edema. The sensitivity and specificity of ICH detection with MRI compared to CT was equally good with 100 %, MRI is superior for the diagnosis of subacute and chronic ICH [41–43].
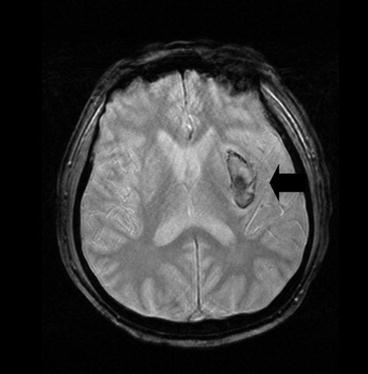
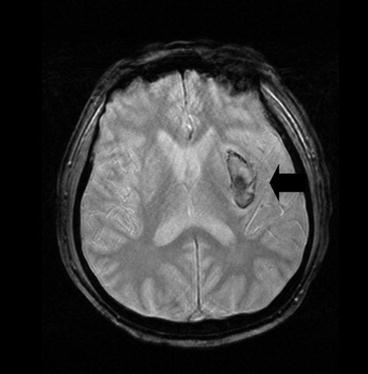
Fig. 13.3
GRE appearance of hyperacute hemorrhage in left basal ganglia image <3 h from symptom onset. Rim of hematoma appears hypointense while core of hematoma has mixed signal intensities
The role of microbleeds (Fig. 13.4), small hemorrhagic lesions seen in chronic hypertensive disease and in cerebral amyloid angiopathy as well as vasculitis and other instances has not been firmly established. While the general risk for first time or recurrent ICH increases with the number of microbleeds, their relevance for making therapeutic decisions in acute stroke remains unknown [44]. In clinical practice, presence of microbleeds should not deter from thrombolytic therapy.
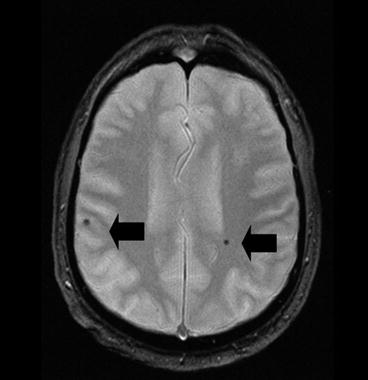
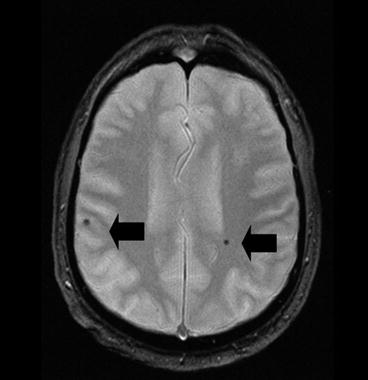
Fig. 13.4
Example of bilateral microbleeds (black arrows) on gradient echo imaging
Diagnosis: Vascular Pathology
Three types of MRA are in clinical use. Time-of-flight (TOF) MRA depends on the movement of blood into the imaging field. The magnetization of protons in stationary tissue is saturated by repeated low flip angle radiofrequency pulses, whereas protons in the vessels flowing into the tissue remain unsaturated and appear relatively bright. The data are then postprocessed using a maximum intensity projection (MIP) algorithm for angiographic reconstruction. In practice, inspection of the source images is often necessary to evaluate subtle or ambiguous findings. Three dimensional rather than two dimensional TOF MRA is the most common implementation of MRA in clinical practice, since it gives superior spatial resolution and is less prone to signal loss from turbulent flow at sites of stenoses, although two dimensional TOF may be more sensitive to slower flow. Phase contrast MRA is another technique for generating angiographic-style images based on the velocity and direction of flow. Phase contrast MRA is based on the principle that moving spins develop a phase shift relative to stationary spins in the presence of a pair of opposing magnetic field gradients. There is superior background suppression with phase contrast MRA, but acquisition times tend to be longer and more prone to artifacts [45].
The most promising new approach is contrast-enhanced MRA of the carotid arteries, in which a more rapid MR acquisition is timed to a bolus injection of contrast over a larger field of view; carotid MRA with contrast compares favorably to conventional angiography for the diagnosis of carotid stenosis. The accuracy of contrast enhanced MRA for detecting vascular disease in vertebral artery origins and the aortic arch is being investigated [45].
MRA rivals conventional angiography for the detection of arterial stenoses and occlusions, although there is a tendency of MRA to overestimate the degree of stenosis because turbulent flow causes proton dephasing; also calcifications at the site of the stenosis may cause interference, and the smaller intracranial vessels are not well visualized. A normal screening MRA is reliable in excluding hemodynamically significant stenoses. False positive results can arise when the degree of carotid stenosis is overestimated or when the carotid artery is kinked or changes direction abruptly, distally in the carotid artery as it enters the carotid canal due to susceptibility artifact between vessel and bone, and in the presence of surgical clips. Sensitivity and specificity for carotid occlusion has been found to be 100 % in most studies. In the intracranial vasculature, MRA is useful in identifying acute proximal large vessel occlusions and stenoses, but is not currently reliable in identifying distal or branch occlusions [45].
MR Imaging for Stroke in Summary
A recent TTA report of the American Academy of Neurology [46] stated the following: DWI is established as useful and should be considered more useful than noncontrast CT for the diagnosis of acute ischemic stroke within 12 h of symptom onset. DWI should be performed for the most accurate diagnosis of acute ischemic stroke (Level A); however, the sensitivity of DWI for the diagnosis of ischemic stroke in a general sample of patients with possible acute stroke is not perfect. On the basis of Class II and III evidence, baseline DWI volumes probably predict baseline stroke severity in anterior territory stroke (Level B) but possibly do not in vertebrobasilar artery territory stroke (Level C). Baseline DWI lesion volumes probably predict (final) infarct volumes (Level B) and possibly predict early and late clinical outcome measures (Level C). Baseline PI volumes predict to a lesser degree the baseline stroke severity compared with DWI (Level C). There is insufficient evidence to support or refute the value of PI in diagnosing acute ischemic stroke (Level U).
Adding sequences such as GRE or SWI for detection of hemorrhage, FLAIR for detection of old vascular lesion load (SAE) and determination of age of an ischemic lesion and MRA for vessel status completes a stroke MRI protocol feasible for acute stroke management. With regard to development and implementation of imaging for acute stroke, two consensus statements have been published, which we recommend to the interested reader [47, 48].
Selection: Identifying the Ischemic Penumbra and Patients at Increased Risk from Therapies
The target for most therapeutic interventions for focal cerebral ischemia should be ischemic tissue that can respond to treatment and is not irreversibly injured. The characterization of potentially reversible loss of function versus irreversible tissue damage is based on the concept of the ischemic penumbra [49]. Until recently only positron emission tomography (PET) and single-photon emission computed tomography (SPECT) imaging could approximately define ischemia and penumbra thresholds. However, it is not feasible to routinely perform these imaging studies in the acute stroke clinical setting.
MRI techniques including PI and DWI added another dimension to diagnostic imaging of the ischemic penumbra [50–53]. In a simplified approach, still valid today more than 10 years after introduction into clinical use, it has been hypothesized that DWI, in the absence of reperfusion, more or less reflects the irreversibly damaged infarct core and PI the complete area of hypoperfusion [54, 55]. The volume difference between these two regions is termed perfusion/diffusion mismatch (Fig. 13.5), i.e. PI minus DWI-volume (sometimes also as ratio: PI/DWI volume). The perfusion/diffusion mismatch volume could measure the ischemic penumbra, i.e. tissue at risk of infarction: the natural history of early diffusion abnormalities in untreated patients is to grow over time into the area of the initial perfusion abnormality. In addition, DWI lesion volume growth can be interrupted with early recanalization and reperfusion [36, 54, 56].
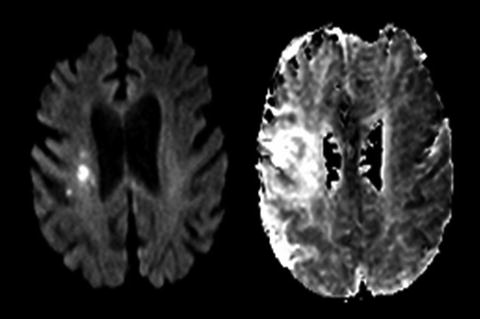
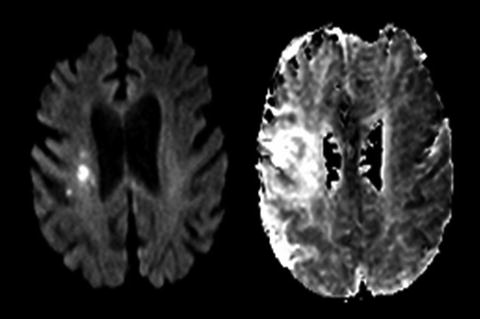
Fig. 13.5
Example of diffusion-perfusion mismatch in a patient imaging with MRI at 4 h after symptom onset. DWI image (left) shows small region of periventricular hyperintensity. Mean transit time perfusion map (right) shows much larger perfusion deficit
Overall, this simple model of diffusion perfusion mismatch appears to be acceptably accurate in many acute stroke patients and the findings of stroke MRI are consistent with our pathophysiological understanding [36, 53]. Using the mismatch concept, it may become possible to categorize patients into two major groups: those that may profit from a specific penumbral salvage therapy and those in whom little ischemic tissue remains to benefit. Recently a third group, the malignant profile identified by extensive ischemic changes on baseline DWI-lesion has been introduced. These patients appear to be at a high risk for fatal secondary hemorrhage after recanalizing therapies [57–60].
The two-zone PI/DWI-mismatch model, however, has two major limitations. The visually identified PI lesion includes not only at-risk tissue, but also regions of benign oligemia (tissue with impaired blood flow, but not in danger of infarcting). At present, the optimum PI algorithm to differentiate oligemia from ischemia has not been established. In addition, DWI abnormalities are potentially reversible, especially with early reperfusion (Fig. 13.6) [61]. Therefore, in some patients, the penumbra includes a portion of the DWI abnormality. These findings have led to a modified model of the MRI defined penumbra (Fig. 13.7) [31]. Despite these limitations, the mismatch model may provide an approximation of the penumbra that is clinically useful and this model has the advantage of simplicity.
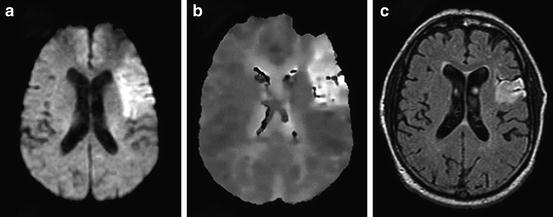
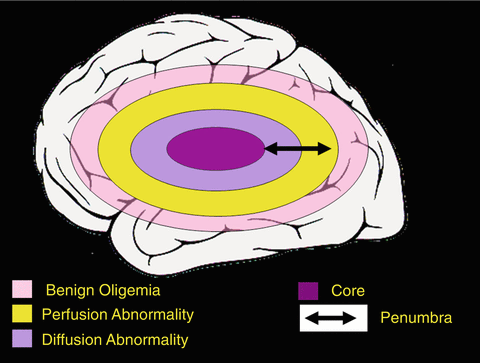
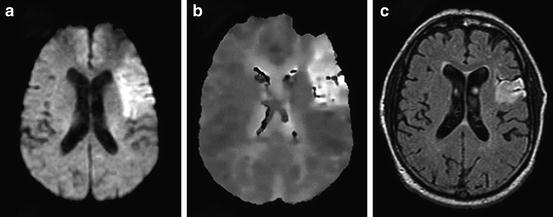
Fig. 13.6
A 74-year-old man with a history of hypertension, diabetes, and new onset atrial fibrillation presented with non-fluent aphasia and right hemiparesis (NIHSS = 7). He was treated with IV alteplase starting 2 h and 42 min after symptom onset. (a) Pretreatment DWI obtained 2 h 5 min after symptom onset is displayed, and (b) the concurrent PWI. (c) Follow-up FLAIR image obtained 108 days after stroke onset. The initial DWI lesion volume was 54.1 cc, and the follow-up FLAIR lesion volume 23.5 cc (reversal of 57 % of acute ischemic lesion). The patient recovered with occasional word finding difficulty and mRS = 1
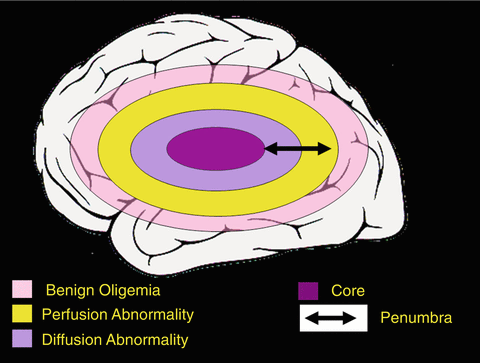
Fig. 13.7
Modified view of ischemic penumbra as defined by MR imaging. This graph actual in 2003 as well as today illustrates that the DWI lesion may depict infarct core and to a lesser extent penumbra, whereas the PI lesion consists of penumbra and oligemic tissue not likely to die. For PI the issue remains unresolved, where the exact threshold between tissue destined to die and not critically hypoperfused brain tissue lies
Over the last decade there have been major efforts to more precisely define the threshold of perfusion delay on time dependent PI sequences (TTP) that ideally matches penumbral tissue, in vain. It has become clear that the longer the chosen delay for a given cutoff, the more likely a tissue destined to die is imaged. On the other hand, this comes at the cost of specificity. The final verdict in terms of the perfect perfusion algorithm remains to be spoken, although in the past as well as currently a TTP or Tmax (TTP-based) perfusion map with a threshold of 4–6 s delay appears to be the best compromise [62, 63] also validated by comparison to H2O [15] PET studies [64].
From Theory to Practice
In stroke centers organized for immediate access to MRI acquisition and interpretation, MRI is often used instead of CT as the neuroimaging screen prior to standard intravenous thrombolytic therapy, with times-to-treatment within published benchmarks. The potential advantages include greater diagnostic accuracy for ischemic and non-ischemic pathology, facilitating thrombolysis in patients with uncertain clinical diagnoses, but definite AICS by MRI criteria, and excluding patients with normal CT but confirmed non-ischemic pathology by MRI. MRI may also serve to screen patients for possible intra-arterial thrombolysis [3, 65], although a recent trial could not show any use or predictive value of stroke MRI patterns in patients selected for thrombectomy with the MERCI device [66]. MR RESCUE, however, suffered from very low recanalization rates, a late time window, a small number of patients and several other flaws. It appears unlikely that the effect of stroke MRI was assessed. In opposite, MR RESCUE illustrated the lack of use of thrombectomy with the MERCI device.
After smaller earlier series [36, 54, 67, 68], a growing body of data is now available suggesting the utility of employing MRI to identify the ischemic penumbra and assessing response to therapies. Chalela and colleagues studied patients with MRI before and after standard intravenous alteplase therapy and found that perfusion defect reduction by more than 30 % within 2–4 h after treatment predicted good outcome (mRS 0–1) more reliably than age, pretreatment NIHSS or other imaging variables [69]. Parsons and colleagues reported a comparison of imaging and clinical outcomes in a group of 16 patients with diffusion-perfusion mismatch treated with intravenous tPA between 3 and 6 h from onset, compared to a group of 16 historical controls [56]. They found that the IV tPA treated patients had a substantially greater rate of recanalization, smaller infarct volume, greater penumbral salvage, and greater clinical improvement. Köhrmann and colleagues assessed 400 consecutive patients treated with rt-PA, 400 of whom were treated CT-based within 3 h, or MRI based within or beyond 3 h after symptom onset [70]. MRI-selected patients had lower rates of hemorrhage and mortality but identical rates of favorable outcomes suggesting that time from symptom onset was less relevant in patients with a penumbral pattern on stroke MRI, even if treated late. In a larger approach the TAKE 5 Collaborative pooled their institutional rt-PA registries [71], and described their results of 1,210 rt-PA treated patients. Three groups (CT <3 h: N = 714; MRI <3 h: N = 316; MRI >3 h: N = 180) were analyzed. Favorable outcomes occurred in 35.4 %, 37.0 %, and 40 % (p = 0.51). The overall use of MRI significantly reduced symptomatic intracranial hemorrhage (OR = 0.520, 95%CI 0.270–0.999, p = 0.05). Beyond 3 h, the use of MRI significantly predicted a favorable outcome (OR = 1.467; 95%CI 1.017–2.117, p = 0.040). In this large, but non-randomized study, MRI-based thrombolysis appeared to be safer and potentially more efficacious than standard CT-based thrombolysis despite of significantly longer time windows and significantly higher baseline NIHSS scores.
In the meantime several abbreviated concepts for mismatch have been proposed, among them the clinical-DWI mismatch, the MRA-DWI mismatch and the FLAIR-DWI mismatch. All of these concepts together are part of the comprehensive evaluation of a potential thrombolysis patient and the clinician will introduce these concepts into his overall assessment. Using only a few of the aspects available with MRI could allow clinicians to reduce and facilitate the imaging protocol, especially by not performing perfusion MRI. DWI, FLAIR, GRE, and maybe MRA suffice to make a decision in many cases. For instance a severe clinical deficit with a small cortical lesion would represent a clinical-DWI mismatch, a small DWI lesion with a proximal M1 occlusion a DWI-MRA mismatch. However, these approaches may also miss additional and potentially useful information. The idea to use a FLAIR DWI mismatch in patients with unknown time of stroke onset as a substitute for an early time window on the other hand appears to be a promising concept, which will be put to test in two trials, an US-based phase IIa trial (MR WITNESS) and a phase III European trial (WAKE UP).
Clinical Trials
Surprisingly, the stroke MRI PI/DWI mismatch concept has been put to test in only a handful of randomized thrombolysis trials. The DIAS/DEDAS desmoteplase study program [72–74], the Australian EPITHET trial [75] and the DEFUSE 1 and 2 trials [59, 65] besides of MR RESCUE [66] are the relevant studies to date. The DIAS-2 trial combined two different imaging modalities (perfusion CT as well as stroke MRI) while the still ongoing DIAS 3 and 4 trials stepped back to a selection mode using vascular occlusion only, but not penumbral imaging. ECASS-4 EXTEND will also be a stroke MRI mismatch based trial with a harmonized protocol congruent with the ongoing EPITHET EXTEND AUSTRALASIA trial [76]. MR WITNESS and WAKE UP are new studies. The former will recruit patients based on PI/DWI-mismatch selection with a full stoke MRI protocol whereas the latter will use an abbreviated MRI protocol (FLAIR/DWI mismatch) in wake up stroke patients.
The DIAS program started with DIAS 1 and DEDAS, both of which showing safety and suggesting efficacy for PI/DWI mismatch based treatment of acute stroke [72, 73] a result which could be repeated in terms of safety but not efficacy in DIAS 2, which also allowed CT-perfusion based inclusion [74]. The latter result may well be due to the inclusion of 70 % of patients without significant occlusion [77]. When pooling patients with occlusions on vascular imaging from DIAS 1, DIAS 2 and DEDAS, desmoteplase was beneficial compared to placebo with a significant OR of 4.144 (95%CI 1.4–12.23, p = 0.01) but not in patients without occlusions (which makes sense) [78].
The DEFUSE and EPITHET studies both showed that reperfusion in patients with a target mismatch translated into a favorable response to treatment, whereas reperfusion would not help patients with no mismatch or cause damage in patients with a malignant profile (more sICH and mortality). The key difference between both trials was that in DEFUSE all patients were treated with IV rt-PA whereas in EPITHET patients were randomized to IV rt-PA or placebo in a 3–6 h time window. In both studies MRI findings were not used for decision making. A pooled analysis of both studies [39, 60] confirmed these results using an automated image analysis software (RAPID). DEFUSE 2 also used RAPID and included patients scheduled for IAT/device in acute stroke. They found the same results for this patient sample as was seen in DEFUSE 1 and EPITHET, i.e. target mismatch patients benefit from reperfusion whereas no mismatch patients and malignant profile patients do not [65]. Interestingly patients with a target mismatch showed a benefit even in very late time windows, a finding that has not been consistently shown in advanced imaging studies before. This finding may indicate that at later time points ischemic core progresses more slowly possibly due to good collateral flow.
In MR-Rescue the imaging profile did not modify treatment response nor clinical outcome in patients randomized between standard of care and (late) thrombectomy. It has to be mentioned however that several methodological problems may have influenced these negative findings and conclusions especially regarding the value of penumbral imaging should be drawn with caution.
EXTEND and ECASS 4 EXTEND are planned or ongoing clinical trials. The EXTEND studies randomize to placebo versus IV rt-PA in the 3–9 h or 4.5–9 h time window. ECASS 4 EXTEND uses the classical non-automated MRI mismatch only, whereas EXTEND AUSTRALIA allows both, RAPID analyzed stroke MRI or perfusion CT [79]. MRI criteria in EXTEND AUSTRALIA include a PI lesion defined by a Tmax delay of >6 s and PI/DWI mismatch of at least 20 %. A pooled analysis is planned.
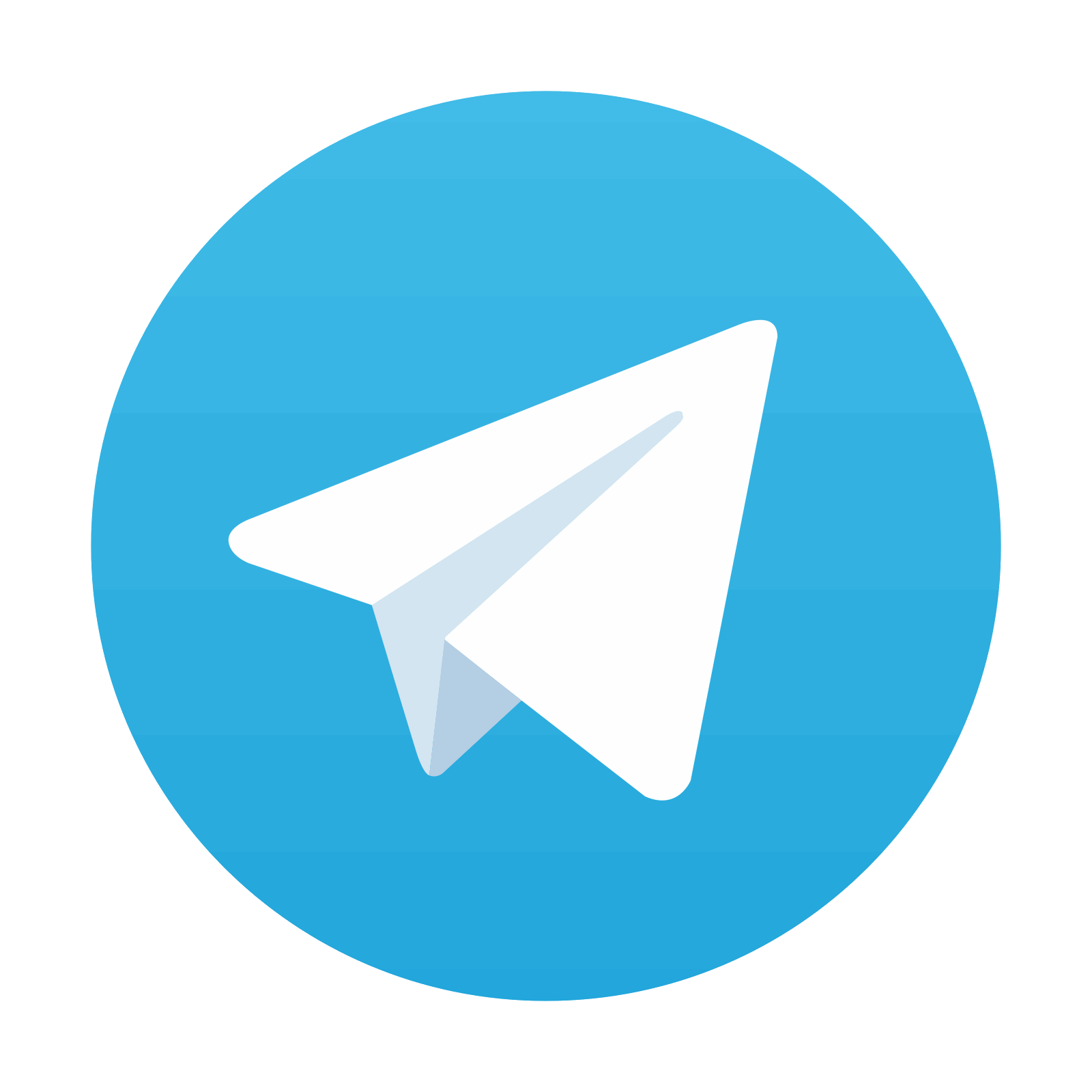
Stay updated, free articles. Join our Telegram channel

Full access? Get Clinical Tree
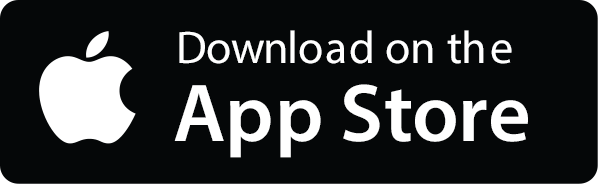
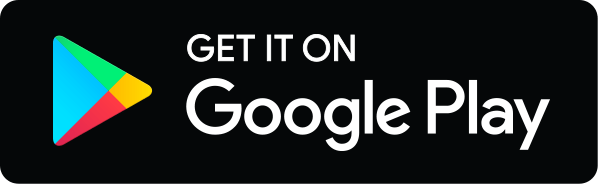