Chapter 13 The knee
The knee, the intermediate joint of the lower limb, is formed by two joints, the knee joint (often referred to as femorotibial or tibiofemoral joint) and the patellofemoral, with the first being the weight-bearing component and the second serving to reduce friction of the quadriceps tendon on the femoral condyles and acting as an ‘anatomic pulley for the quadriceps muscle’ (Levangie & Norkin 2005). Kapandji (1987) expresses the paradoxical ‘mutually exclusive’ requirements of the knee joint as having to provide ‘great stability in complete extension, when the knee is subjected to severe stresses resulting from body weight and the length of the lever arms involved’ as well as great mobility, essential when running or gait on uneven ground, which is achieved only with a certain degree of flexion. ‘The knee resolves this problem by highly ingenious mechanical devices but the poor degree of interlocking of the surfaces – essential for great mobility – renders it liable to sprains and dislocations.’
The knee is not well protected by fat or muscle mass, making it relatively susceptible to trauma. Additionally, it is often subjected to maximal stress (being located at the intersection of two long levers) and is ‘probably the most vulnerable of all structures of the body to soft tissue injury with attendant pain and impairment’ (Cailliet 1996). The knee is unstable during flexion, making its ligaments and menisci most susceptible to injury; however, fractures of the articular surfaces and ruptures of the ligaments are more likely during extension injuries (Kapandji 1987). Due to its easily palpable contours and features, coupled with potential use of arthroscopic examination, if needed, the diagnostic process for the knee is fortunately far easier than for many other joints of the body (Hoppenfeld 1976).
In writing this chapter, we have included many quotes from the skilled writings of Pamela Levangie and Cynthia Norkin (2005) (and their contributing authors), who have described this complex joint and its complicated movements in their book, Joint structure and function: a comprehensive analysis. Their dedication to clarity and accuracy of information is particularly obvious in such a difficult subject as the knee joint.
The knee joint is discussed first in this chapter, with the patellofemoral joint, whose function is distinctly different, following it. The discussions in this chapter start from the inside with the bony surfaces and then progress outwardly, through the menisci, ligaments, joint capsule and, finally, the muscular elements. The proximal tibiofibular joint, which relates functionally to the ankle joint (Levangie & Norkin 2005), is not enclosed within the joint capsule of the knee and is therefore not discussed in this chapter. Details regarding the tibiofibular joints are to be found in Chapter 14 with the ankle and foot complex.
Regarding terminology of the region of the knee, the common use of both tibiofemoral joint and femorotibial joint appears in textbooks as well as journal articles. Gray’s anatomy (2005) uses only tibiofemoral joint, however, Gray’s Anatomy for Students (Drake et al 2010) does not. Terminologia anatomica (FCAT 1998), an international standard on human anatomical terminology developed by the Federative Committee on Anatomical Terminology (FCAT) and the International Federation of Associations of Anatomists (IFAA), suggests that the appropriate name is the knee joint. We have adopted that term throughout this chapter except in direct quotes from other authors, which show terms used by them.
The knee joint
The knee joint, the largest and most complicated joint in the body, is a special type of hinge joint. While hinge joints normally allow one degree of movement, this trochoginglymus joint allows flexion/extension of the joint, produced by a combination of rolling and gliding, and, when in a flexed position, also allows a small degree of rotation (Platzer 2004). Because it must perform its movement while also bearing most of the body’s weight (at times well over five-sixths of the entire weight of the body), it seems as though stability of this joint should be a primary feature when, in fact, the joint design itself engenders relative instability. The following summations by no means explain the detailed architecture of the knee joint but they are intended to give a simplistic yet encompassing view of the knee on which basic assessment skills may be built. Readers interested in a deeper understanding of the mechanics of this joint (and others) are referred to Volume 2 of three volumes titled The physiology of the joints, which were beautifully mastered and illustrated by I. A. Kapandji.
Regarding the relationship of the femur and tibia, Gray’s anatomy (2005) notes:
The incongruence of the convex femoral condyles and the concave tibial condyles is significant, so much so that interposed menisci are needed to achieve a degree of stability. However, it is the ligamentous and muscular components – not joint congruency – that primarily support this joint (Cailliet 1996). Because an understanding of the bony and cartilaginous features is complicated, yet essential, they are deserving of detailed discussion.
The femur
The femur is the longest and strongest bone in the human body, its strength evident by its weight and its power obvious by its muscular forces (Gray’s anatomy 2005). It is composed of:
• a head at the proximal end – projected by its short neck to meet the acetabulum (see Chapter 12)
• a shaft that is almost cylindrical. It displays three surfaces (anterior, lateral and medial) and their associated borders, bows forward and has a degree of torsion around its vertical anatomical axis. This anatomical axis courses downward and medially at an oblique angle to meet the vertically oriented tibia, providing the knee joint with a normal valgus angle of 5–10°
• double condyles on the distal end are separated by an intracondylar notch or fossa, with the medial condyle extending further distally as well as being longer than the lateral condyle.
Because weight-bearing forces follow a mechanical rather than anatomical axis, the angulation of the femur assists in placing the femoral condyles under the head of the femur so that, in a normally positioned leg, the weight-bearing line passes through the center of the knee joint (between the condylar tubercles) and then through the center of the talus. Levangie & Norkin (2005) note:
• an anterior surface that is smooth and gently convex
• a lateral surface, which has as a posterior boundary the linea aspera, that displays itself as a crest with lateral and medial edges, diverging proximally (to form the gluteal tuberosity) and distally toward the condyles, to form the medial and lateral supracondylar lines
• a medial surface, which has the linea aspera as its posterior border.
• The femoral shaft lies within a muscular envelope. The following attachments are shown in Figure 13.1.
• Vastus intermedius (VI) attaches anteriorly and laterally on its upper three quarters.
• Articularis genus attaches on the anterior surface just distal to the end of the VI.
• The most distal anterior surface is covered by a suprapatellar bursa.
• The medial surface, devoid of attachments, is covered by vastus medialis.
• At the proximal anterior surface can be seen a small attachment of vastus lateralis and vastus medialis.
• At the greater trochanter the gluteus minimus and medius, piriformis and the remaining deep hip rotators attach.
• At the lesser trochanter, the iliopsoas is the only attachment, with iliacus extending down the shaft a short distance.
• Gluteus maximus attaches posteriorly to the gluteal tuberosity, which is continuous with
• the linea aspera, which provides attachments for the adductor group, vastus medialis, vastus lateralis and short head of biceps femoris as well as the intermuscular septa.
• Distally, on the posterior and lateral aspects of the femur, the gastrocnemius, plantaris and popliteus attach as well as the adductor magnus, which attaches to the adductor tubercle.
Femoral condyles
The distal end of the femur is constructed for the transmission of weight to the tibia (Fig. 13.2), with two formidable condyles. These condyles are convex in both a frontal and sagittal plane and are bordered through their length by a saddle-shaped groove, which unites them anteriorly (as the patellar groove or surface) and which separates them posteriorly (as the intercondylar notch or fossa). Anteriorly, these condyles merge with the shaft, united by, and continuous with, the patellar surface (as described with the patellofemoral joint on p. 462). They do not articulate with the proximal end of the fibula (Fig. 13.3), which articulates only with the lateral proximal tibia.
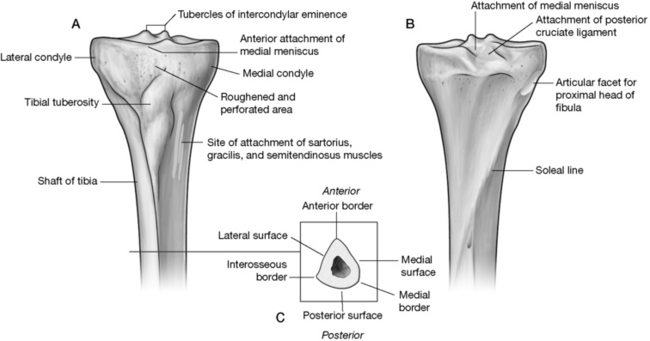
Figure 13.2 The proximal tibia. A: Anterior view; B: Posterior view; C: Crossection
(Reproduced, with permission, from Gray’s anatomy for students, 2nd edn, 2010, Churchill Livingstone)
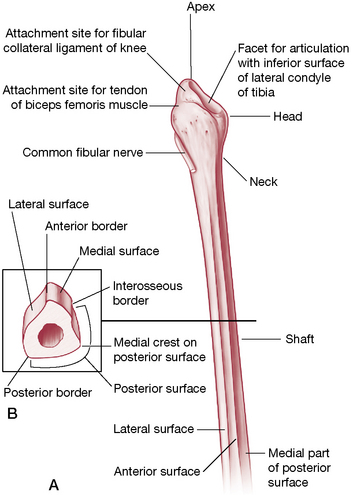
Figure 13.3 The proximal fibula. Note facet that articulates with the lateral tibia.
(Reproduced, with permission, from Gray’s anatomy for students, 2nd edn, 2010, Churchill Livingstone)
The medial and lateral femoral condyles, which diverge distally and posteriorly, can be compared. They offer the following features (Platzer 2004) (Figs 13.4A, 13.5, 13.6). Both femoral condyles are covered with articular cartilage.
• The medial condyle is uniform in width while the lateral condyle is narrower in the back than in the front.
• The medial condyle extends more distally, which counters the oblique position of the femoral shaft, placing the condyles ‘in the same horizontal plane despite their different sizes’ (Platzer 2004).
• The two condyles are almost equally (and only slightly) curved in a transverse plane about the sagittal axis.
• In the sagittal plane, the curvature increases posteriorly, resulting in a decreased radius posteriorly, placing the mid-points of the curve on a spiral line and resulting in ‘not one but innumerable transverse axes, which permits the typical flexion of the knee joint that consists of sliding and rolling motion’ (Platzer 2004).
• An additional vertical curvature on the medial condyle (as seen from below) allows for a rotational feature during flexion.
• The articulating surface of the lateral femoral condyle (excluding the patellar surface) is shorter than the articular surface of the medial femoral condyle.
• Proximal to the medial condyle lies the medial epicondyle, which receives the tibial (medial) collateral ligament and, on its upper edge, the adductor magnus attaches to its adductor tubercle.
• The fibular (lateral) collateral ligament attaches to the lateral epicondyle (above the lateral condyle) and the lateral head of gastrocnemius attaches posterosuperior to this.
Intercondylar fossa
• On the medial surface of the lateral condyle, which makes up the lateral wall of the fossa, is the smooth proximal attachment site for the anterior cruciate ligament.
• On the lateral surface of the medial condyle, which makes up the fossa’s medial wall, is the smooth proximal attachment site for the posterior cruciate ligament.
• The popliteal surface of the femur is a triangular surface delimited by the medial and lateral supracondylar lines and, distally, by the intercondylar line (upper edge of intercondylar fossa).
Regarding the popliteal region:
• the medial head of gastrocnemius attaches a little above the medial condyle
• various arteries lie close by, including the popliteal artery, which arches above the condyle as it branches from the superior medial genicular artery
• plantaris attaches to the distal part of the lateral supracondylar line, separating the lateral genicular artery from bone
• the medial supracondylar line provides the attachment for vastus medialis and the tendon of adductor magnus. The line is crossed by femoral vessels, which enter the popliteal fossa from the adductor canal
• this triangular area is the upper half of the diamond-shaped ‘popliteal fossa’, a region that requires caution during palpation due to the course of relatively exposed neurovascular structures
The proximal tibia
The vertically oriented tibia (Fig. 13.2) lies medial to, and is stronger than, the accompanying fibula. (Fig. 13.3) The tibia’s proximal end, the tibial plateau, provides a surface for articulation with the femur, thereby allowing transmission of the body’s weight as well as ground reaction forces (13.4A). When both forces are transmitted strongly, as in jumping from an elevated position, the knee joint and its internal elements are at increased risk for injury. Additionally, when the angulation of the femur and tibia is other than normal (genu valgum, genu varum), significant changes take place in the weight-bearing pressures on the menisci and cartilage (see Box 13.1).
Box 13.1 Weight-bearing forces and knee joint alignment (Platzer 2004)
The mechanical axis of the lower limb lies on a straight line drawn through the center of three joints: the hip, the knee and the ankle (Fig. 13.7A). This mechanical axis coincides with the anatomical axis of the tibia, but the anatomical axis of the femur is diagonally inclined, which forms a 6° acute angle with the mechanical axis and gives the knee joint a normally slightly valgus position.
In genu valgum (knock knees), the weight-bearing line is displaced laterally and courses through the lateral femoral condyle and head of the fibula, overstretching the medial collateral ligament and placing excessive stress on the lateral meniscus and the cartilaginous joint surfaces of the lateral tibial and fibular condyles (Fig. 13.7B).
In genu varus (bow legs), the weight-bearing line is displaced medially and courses through the medial femoral condyle or medial to it, overstretching the lateral collateral ligament and placing excessive stress on the medial meniscus and the cartilaginous joint surfaces of the medial tibial and fibular condyles (Fig. 13.7C).
Tibial plateaus (superior articular facets) (Figs 13.8, 13.9)
• During knee flexion the intercondylar eminence slides in the intercondylar groove of the femur as well as becoming a fulcrum around which rotation can take place when the knee is flexed. The complexity of these concepts is well illustrated and described by Kapandji (1987) as a mechanical model.
• At full extension, the eminence becomes lodged in the intercondylar notch of the femur and the tibia then rotates about it in the final stage of extension (Levangie & Norkin 2005). This ‘screw home’ locking mechanism results in an automatic (terminal) rotation of the knee joint that brings the joint into a close-packed position and ‘locks’ it in extension. It must then be ‘unlocked’ before flexion can occur or else damage may result.
• In front of the intercondylar eminence lies the anterior intercondylar area to which the anterior cruciate ligament attaches and, anterior to this, the anterior horn of the medial meniscus attaches. The anterior horn of the lateral meniscus attaches lateral to the anterior cruciate ligament (see Fig. 13.9).
• Behind the eminence lies the attachment of the posterior cruciate ligament in the posterior intercondylar area. Between the attachments of the two cruciate ligaments lie the attachments of the posterior horns of both menisci (see Fig. 13.9).
• The articulating surface of the lateral condyle is half the size of that of the medial condyle and its articular cartilage is one-third as thick as that of the medial condyle.
• The articular surfaces of the tibial plateau are concave centrally but flatten peripherally. The menisci rest, one on each condyle, on the flattened portion of the surface and increase the concavity of each tibial condyle (Gray’s anatomy 2005) (see description of the menisci below).
• From a frontal perspective, both tibial condyles are concave yet shallow; however, the two condyles differ in anteroposterior profile. The medial condyle is concave while the lateral condyle is convex, adding to the instability of the joint, as the lateral femoral condyle must ride up and over this slope during joint movements (Fig. 13.10).
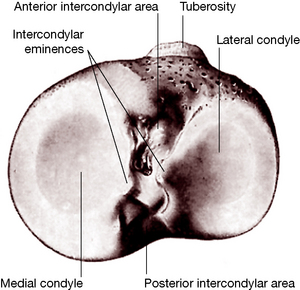
Figure 13.8 The proximal articular surface of the right tibia
(reproduced with permission from Gray’s anatomy 1995).
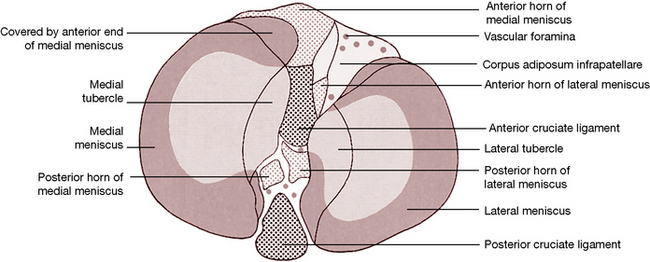
Figure 13.9 Surface features of the proximal aspect of the right tibia
(reproduced with permission from Gray’s anatomy 1995).
Kapandji (1987) notes:
The anterior and posterior cruciate ligaments are discussed further below.
Menisci (Fig. 13.11)
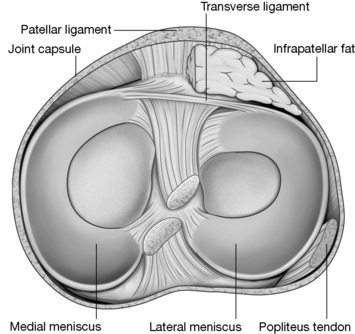
Figure 13.11 Superior aspect of the right tibia showing the menisci and the tibial attachments of the cruciate ligaments.
(Reproduced, with permission, from Gray’s anatomy for students, 2nd edn, 2010, Churchill Livingstone)
• the entire meniscus in the infant
• only the outer third of the meniscus, while the middle and inner thirds remain avascular, in the adult
Levangie & Norkin (2005) comment on the effects of this decreasing blood supply.
The nerve supply to the menisci is substantial; free nerve endings supply nociceptive information while mechanoreception is offered by Ruffini corpuscles, Pacinian corpuscles and Golgi tendon organs (Levangie & Norkin 2005), indicating that the menisci are a source of information about joint position, direction of movement and velocity of movement as well as information about tissue deformation.
Dysfunctional joint mechanics, ligamentous injuries and arthritic changes, as examples, can severely disrupt the proprioceptive function of the knees (Koralewicz & Engh 2000) (see Box 13.6).
Box 13.6 Proprioception and the arthritic knee
Koralewicz & Engh (2000) compared proprioception in arthritic and age-matched normal knees. They note that proprioception: ‘the ability to sense joint position and joint motion – is affected by factors such as age, muscle fatigue, and osteoarthritis’. The purpose of their study was to determine whether there was a difference in proprioception between arthritic knees and nonarthritic, age-matched, normal knees. Additionally they sought to evaluate whether, when proprioception is reduced in an arthritic knee, it also was reduced in the opposite knee irrespective of the presence of arthritis. One hundred and seventeen patients who were scheduled for total knee arthroplasty due to severe arthritis (mean age 67.9 years) were compared with a control group of 40 patients who were recruited from a hospital-based cardiac rehabilitation program and did not have knee arthritis (mean age 68.3 years).
• middle-aged and elderly persons with advanced knee arthritis were significantly less sensitive to the detection of passive motion of the knee than middle-aged and elderly persons without knee arthritis.
• the ability to detect passive motion was reduced in both knees when arthritis was present in only one knee.
The collagen fibers of each meniscus are arranged in two directions.
• The medial two-thirds comprise radially organized collagen bundles, lined by thinner collagen bundles parallel to the surface. This suggests a biomechanical compression coping function.
• The peripheral third comprises larger circumferentially arranged bundles, suggesting biomechanical tension coping functions.
• The peripheral circumferential fibers are strongly anchored to the intercondylar bone, preventing outward displacement of the menisci.
The wedge-shaped menisci each provide three surfaces: the superior surface (1) which articulates with the femur, the peripheral surface (2) with its overall cylindrical shape, which is in contact with and adherent to the deep surface of the joint capsule, and the inferior surface, which rests on the tibial condyle (Fig. 13.12).
Kapandji (1987) describes the attachments of the menisci and notes that the meniscal attachments between the femoral and tibial surfaces are important from the functional point of view.
• They attach to the deep surface of the capsule; the medial meniscus firmly attaches while the lateral meniscus has very loose connections.
• Each horn anchors to the tibial condyle in the anterior and posterior intercondylar fossae, respectively.
• Lateral meniscus: the anterior horn (4) attaches just in front of the lateral intercondylar tubercle while the posterior horn (5) attaches just posterior to the same tubercle.
• Medial meniscus: the anterior horn (6) inserts in the anteromedial angle of the anterior intercondylar fossa while the posterior horn (7) attaches in the posteromedial angle of the posterior intercondylar fossa.
• The transverse ligament of the knee (8) links the two anterior horns and (rarely) may attach to the tibial plateau (de Abreu et al 2007). It is found in approximately 73.5% of knee joints (de Abreu et al 2007), being absent in the remainder (de Abreu et al 2007, Gray’s anatomy 2005, Platzer 2004).
• The meniscopatellar fibers (9) run from the lateral edges of the patella (P) to the lateral borders of each meniscus.
• The medial (tibial) collateral ligament (MCL) is attached to the internal border of the medial meniscus.
• The lateral (fibular) collateral ligament (LCL) is separated from its corresponding meniscus by the tendon of the popliteus (Pop), which itself attaches (10) to the posterior border of the lateral meniscus (LM).
• The semimembranosus tendon (11) attaches by fibrous expansion to the posterior edge of the medial meniscus (MM).
• The menisco-femoral ligament (fibers from the posterior cruciate ligament) are inserted into the posterior horn of the lateral meniscus (12). A few fibers of the anterior cruciate ligament insert into the anterior horn of the medial meniscus as well.
The ability to resist both the compression and tension forces is especially important in the knee joint, as described by Levangie & Norkin (2005).
Gray’s anatomy (1995) reports that menisci ‘spread load by increasing the congruity of the articulation, give stability by their physical presence and as providers of proprioceptive feedback, probably assist lubrication, and may cushion extremes of flexion and extension.’ In adults, the peripheral zone is vascularized; however, the inner regions, where most tears occur, are avascular. Hence, peripheral tears have repair possibility, whereas with central tears resection may be the best choice.
Fibrous capsule and synovial membrane
• The posterior, vertical fibers attach proximally to the posterior margins of the femoral condyles and inter-condylar fossa; distally to the posterior margins of the tibial condyles and intercondylar area; proximally on each side with gastrocnemius attachments, strengthened centrally by the oblique popliteal ligament (derived from the tendon of semimembranosus), which thickens it.
• Medial capsular fibers attach to the femoral and tibial condyles where the capsule blends with the medial (tibial) collateral ligament.
• Lateral capsular fibers attach to the femur above popliteus and follow its tendon to the tibial condyle and fibular head. It is interrupted where popliteus emerges. A prolongation of the iliotibial tract fills in between the oblique popliteal and lateral (fibular) collateral ligament and partially covers the latter.
• Anteriorly, the capsule blends with expansions from the vasti medialis and lateralis, which attach to the patellar margins and patellar ligament, from where fibers extend posteriorly to the collateral ligaments and tibial condyles. Medial and lateral patellar retinacula are formed with the lateral being augmented by the iliotibial tract. An absence of capsule proximal to the patella allows for continuity of the suprapatellar bursa with the joint.
• The capsule attaches internally to the meniscal rims, which affords them a connection to the tibia by short coronary ligaments.
Regarding the synovial lining, Levangie & Norkin (2005) note: The synovial lining of the joint capsule is quite complex and is among the most extensive and involved in the body’. They describe the ensheathing, infolding synovial lining in detail, noting its adherence to the fibrous layer of the capsule except posteriorly where ‘the synovium breaks away from the inner wall and invaginates anteriorly between the femoral condyles’. It adheres to the sides and anterior portion of the anterior and posterior cruciate ligaments, resulting in these ligaments, while contained within the knee joint capsule, being excluded from the synovial sleeve.
Gray’s anatomy (2005) notes that:
Kapandji (1987) offers a detailed description of the variable plicae (recesses, pleats) of the synovial lining as well as the infrapatellar pad, a considerable pad of adipose tissue located between the patella and the anterior inter-condylar fossa. Regarding the plicae, Levangie & Norkin (2005) state:
Bursae
There are many bursae in the region of the knee, some of which are continuous with the joint capsule. (Fig. 13.13)
The most important include the following:
• subcutaneous prepatellar bursa between the lower patella and skin allows movement of the skin over the patella during flexion and extension
• infrapatellar bursa between the tibia and patellar ligament reduces friction between these two surfaces
• subcutaneous infrapatellar bursa between the distal part of the tibial tuberosity and skin may become irritated by kneeling or by direct trauma
• suprapatellar bursa between the femur and quadriceps femoris is continuous with the joint capsule.
• between the lateral collateral ligament and the tendon of biceps femoris
• between the lateral collateral ligament and the tendon of popliteus
• between the tendon of popliteus and the lateral femoral condyle, usually an extension from the joint.
• between the medial head of gastrocnemius and fibrous capsule
• between the medial collateral ligament and the tendons of sartorius, gracilis and semitendinosus
• various bursae deep to the medial collateral ligament between the capsule, femur, medial meniscus, tibia or tendon of semimembranosus
• between the tendon of semimembranosus and the medial tibial condyle.
Regarding the bursae that communicate with the joint capsule, Levangie & Norkin (2005) note:
The three bursae that are connected to the synovial lining of the joint capsule allow the lubricating synovial fluid to move from recess to recess during flexion and extension of the knee. In extension, the posterior capsule and ligaments are taut and the gastrocnemius and subpopliteal bursae are compressed. This shifts the synovial fluid anteriorly (Rauschining 1980). [Fig. 13.14A] In flexion, the suprapatellar bursa is compressed anteriorly and the fluid is forced posteriorly. [Fig. 13.14B] When the joint is in the semiflexed position, the synovial fluid is under the least amount of pressure. Clinically, when there is an excess of fluid within the joint cavity, as a result of injury or disease (termed joint effusion), the semiflexed knee position helps to relieve tension in the capsule and, therefore, minimizes discomfort.
Ligaments of the knee joint
The health of the ligaments and capsule of the knee joint is critical to knee stability and in maintaining integrity, and also in knee joint mobility (Levangie & Norkin 2005). The various ligaments play critical roles in preventing excessive knee extension, controlling varus and valgus stresses at the knee, preventing excessive anterior and posterior displacement as well as medial and lateral rotation of the tibia beneath the femur and modulating various combinations of displacement and rotation, collectively known as rotatory stabilization (Levangie & Norkin 2005). The most important of the knee ligaments include:
• patellar ligament (ligamentum (tendo) patellae) (discussed later)
• anterior and posterior cruciate ligaments
• medial (tibial) and lateral (fibular) collateral ligaments
• arcuate popliteal and posterior oblique ligaments
• transverse ligament (discussed with the menisci)
Cruciate ligaments (Fig. 13.15)
The anterior and posterior cruciate ligaments are very powerful structures that cross each other (hence their name) as they run anteriorly and posteriorly from their tibial attachments to their femoral attachments. Although located centrally within the joint capsule, they lie outside the synovial membrane, which invaginates around them to their anterior surface. These two ligaments are considered to be significantly responsible for ensuring stability of the knee and, when damaged, contribute to considerable impairment and disability. Cailliet (1992) interestingly notes, however, that ‘…there are several reported cases of congenital absence of cruciate ligaments with apparent normal knee function (Johansson & Aparisi 1982, Noble 1976, Tolo 1981), which brings into question why traumatic impairment of the ACL causes such disability’.
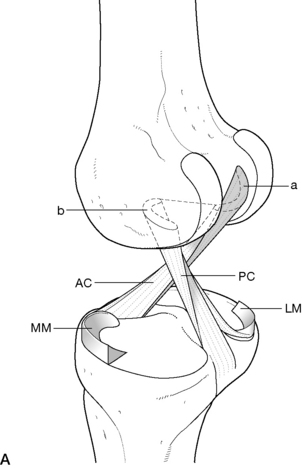
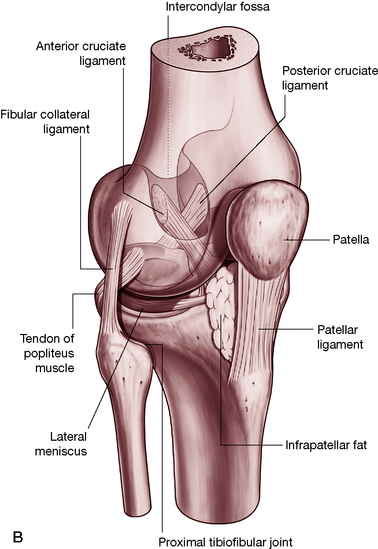
Figure 13.15 A: The cruciate ligaments. a: Attachment of anterior cruciate ligament (AC) b: Attachment of posterior cruciate ligament (PC); medial meniscus (MM); lateral meniscus (LM). (reproduced with permission from Kapandji 1987); B: The complexities of the knee joint are made ‘visible’ in this transparency graphic. (Reproduced, with permission, from Gray’s anatomy for students, 2nd edn, 2010, Churchill Livingstone)
Both cruciate ligaments are composed of type I collagen separated by type III collagen fibrils, as well as abundant fibroblasts. Levangie & Norkin (2005) note:
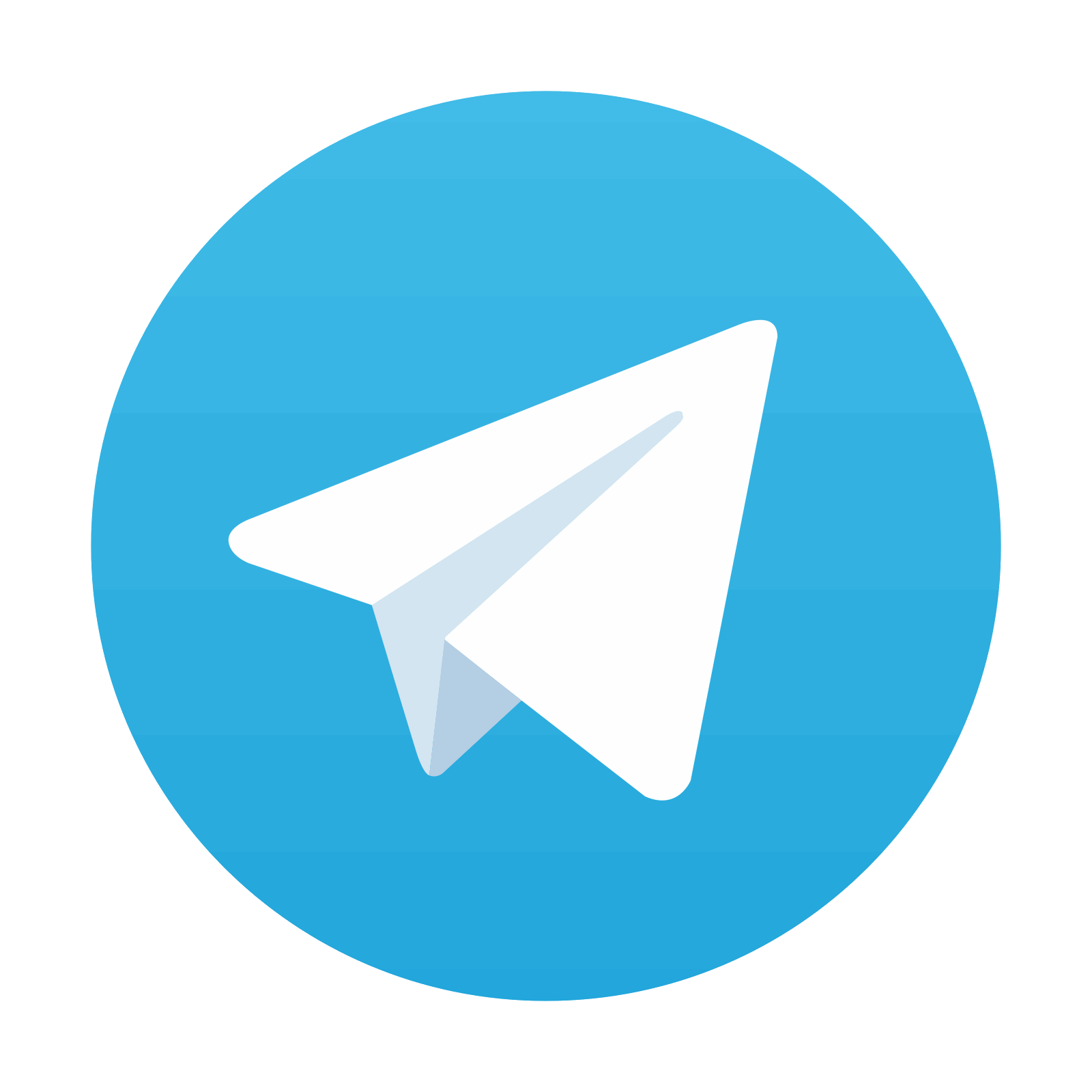
Stay updated, free articles. Join our Telegram channel

Full access? Get Clinical Tree
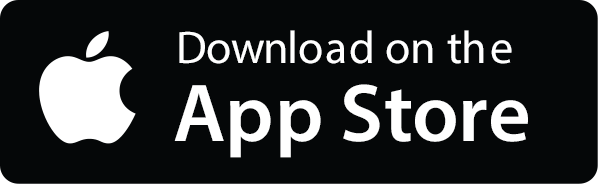
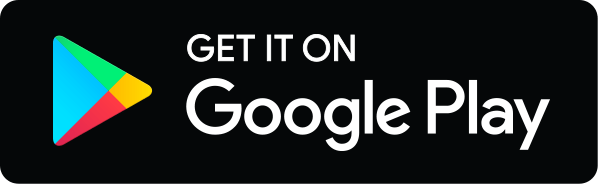