Chapter 14 The leg and foot
Some of the terminology regarding movements of the foot is not universally agreed upon and clarifications are listed in Box 14.1.
Box 14.1 Semantics: clarifying terminology
In considering the terminology used to describe the foot’s position and movements, differences in nomenclature are commonly found, which creates confusion for most readers. This type of confusion is not confined to the lower extremity, nor is it a new situation. Within the medical professions, unclear terminology may result in serious consequences (Greathouse et al 2004). In 1998, the Federative Committee on Anatomical Terminology (FCAT) and the International Federation of Associations of Anatomists (IFAA) updated a previously recognized authoritative work (Nomina Anatomica) and published it under a new title, Terminologia Anatomica. This text contains parallel listing of Latin terms and English equivalents that designate the structures of the human body. Having been developed by an international committee of anatomists, is intended for worldwide use as the definitive nomenclature text (FCAT 1998).
The following points are offered to help clarify the terms adopted with in this NMT text.
• Regarding the lower extremity, the portion that lies between the hip and knee joints is the thigh, while the portion that lies between the knee and ankle joints is the leg. ‘Lower leg’ is that portion of the leg that lies near the ankle.
• What was previously known as peroneal is now fibular, e.g. peroneus longus is fibularis longus and peroneal nerve is fibular nerve. This is more descriptive of the region and it helps to prevent confusion with similar terms, such as the perineum (perineal) or the peritoneum (peritoneal).
• Standard anatomical position describes the foot divided into the tarsus (seven bones), metatarsus (five) and phalanges (14 + two sesamoids) (Gray’s anatomy 2005). However, regarding functionality, the foot can be better divided into three functional segments: the hindfoot (calcaneus and talus), the midfoot (navicular, cuboid and three cuneiforms) and the forefoot (five metatarsals, 14 phalanges and two sesamoids).
• Dorsal and plantar surfaces replace the terms anterior and posterior respectively, while proximal and distal are used in their normal manner.
• ‘Crural’ pertains to the leg.
• Flexion of a joint approximates the joint surfaces so as to create a more acute angle. (The reader might reflect on whether this descriptive ‘rule’ is used consistently, for example in relation to normal cervical and lumbar curves where the creation of more acute joint angles occurs when these areas are extended, rather than flexed [i.e. backward bending should really be called ‘flexion of the lumbar spine’!], which might add confusion rather than clarity to texts.) In regard to the foot, moving the dorsal surface of the foot toward the tibia constitutes flexion of the ankle joint. Therefore, movement in the opposite direction constitutes extension of the joint. However, with the foot, these movements are usually termed dorsiflexion and plantarflexion, respectively. While some authors feel the use of the term plantarflexion is inappropriate (Kapandji 1987), it does clarify a movement that might otherwise be even more unclear. Some of the confusion surrounding the use of flexion and extension regarding the ankle is due to the fact that the toe extensors assist in creating ankle flexion while the toe flexors assist in ankle extension. The terms dorsi- and plantarflexion help in this dilemma and are therefore used in this text to define flexion and extension of the ankle, respectively.
• Supination and pronation are often used synonymously with inversion and eversion of the foot. However, one set of terms often relates to movement about a longitudinal axis while the other set defines a simultaneous triplanar movement about the longitudinal, horizontal and vertical axis. It is not surprising that these are confused since definitive texts have no universal alignment regarding their usage. Regarding this terminology issue, Levangie & Norkin (2001) explain:
• In the subsequent edition of their text, Levangie & Norkin (2005) suggest ‘…some of these terminology differences are not really as problematic as they might initially seem.’ We suggest that, regardless of the term employed to describe it, it is most important to realize that the movement that turns the sole of foot toward the mid-line and elevates its medial aspect (whether called supination or inversion) is a triaxial movement involving rotation about a vertical, longitudinal and horizontal axis.
• Regarding this particular terminology debate, Gray’s anatomy (2005) points out that ‘Pronation and supination are usually better terms than eversion and inversion, as the latter rarely occur in isolation and the former describe the “compound” motion that usually occurs’.
• For simplicity in this text, the term supination is used to describe the lifting of the medial border of the foot and pronation to describe the lifting of the lateral border of the foot. Inversion and eversion are used infrequently in this text (e.g., in direct quotes) to describe the same movements.
The leg
The tibia, the second longest bone in the body and its companion, the fibula, are vertically oriented and articulate at both their upper and lower ends (Figs 14.1, 14.2).
The proximal tibia (described on p. 450) has (on the inferior surface of its lateral projection) a fibular facet, which faces distally and posterolaterally to receive the fibular head. This articulation comprises the proximal tibiofibular joint. While this joint does not provide a high degree of movement, dysfunctions within this joint are an important consideration when the ankle is being assessed due to the potential impact this may have on distal tibiofibular joint function, and, therefore, movements of the ankle joint.
The triangular shaft of the tibia has a medial, lateral and posterior surface, the borders of which are fairly sharply defined. The medial surface is immediately recognizable as the ‘shin’, while the interosseous border is the attachment site for the interosseous membrane. When compared to the proximal end, the distal end of the tibia, where its medial malleolus projects inferomedially, is noted to be rotated laterally (tibial torsion) about 30°, this being significantly more in Africans (Eckhoff et al 1994). The distal surface, which articulates with the talus, is concave sagittally, convex transversely and is continuous with the malleolar articular surface. The medial malleolus lies anterior and proximal to the lateral malleolus. Various ligaments (described below) and the joint capsule attach to it.
The fibula has a proximal head, a long, thin shaft and a distal projection, the lateral malleolus. The head offers a round facet, which articulates with the tibia. Like the tibia, the fibula has three borders and surfaces, the details of both being well described by Gray’s anatomy (2005). Its distal end articulates with the lateral talar surface and is joined to the tibia as a syndesmosis (fibrous union that does not directly contact) to form the ankle mortise.
The proximal tibiofibular joint
The proximal tibiofibular joint is a synovial joint that is not directly connected with the knee joint. When the knee is flexed, this joint plays a part in rotation of the leg, allowing small degrees of supplementary abduction and adduction of the fibula (Lewit 1985) or, as Kuchera & Goodridge (1997) term it, ‘anterolateral and posteromedial glide of the fibular head’.
Greenman (1996) notes that the behavior of the fibular head is strongly influenced by the biceps femoris muscle, which attaches to it, suggesting that any dysfunction of the tibiofibular joint calls for assessment of this muscle, for length, strength and localized dysfunction (trigger points). Schiowitz (1991) notes that: ‘When evaluating or treating a fibular head dysfunction, the [practitioner] should completely examine the distal articulation as well, at the ankle joint’. Kuchera & Goodridge (1997) point out that functional movement of the distal tibiofibular joint is imperative as it allows the ankle mortise to accommodate the increased width of the posterior portion of the talus during dorsiflexion.
Proximal tibiofibular joint play
Lewit (1985) reports joint play at the proximal tibiofibular joint as involving an anteroposterior glide as well as some rotation potential of the fibular head on the tibia.
• When the tibia and ankle are externally rotated, the proximal fibular head elevates and glides (translates) anteriorly, to accommodate this movement (Kuchera & Goodridge 1997) (Fig. 14.3).
• Similarly, when the tibia and ankle are internally rotated, the proximal fibular head depresses and glides (translates) posteriorly, to accommodate this movement.
The proximal tibiofibular joint’s role in ankle sprains
Details regarding ankle sprains are discussed on p. 512. In addition to those considerations, Kuchera & Goodridge (1997) suggest that in cases of recurrent ankle sprain, examination for fibular head dysfunction should be carried out, ‘because with trauma the physiologic, reciprocal motion [between the distal and proximal tibiofibular articulations] may not occur’.
Greenman (1996), discussing the problem of recurrent ankle sprain, suggests they ‘are difficult to treat’ and that ‘structural diagnostic findings in this population consistently show dysfunction at the proximal tibiofibular joint and dorsiflexion restrictions of the talus at the talotibial articulation’. Greenman further observes that common findings include loss of subtalar joint play, pronation of the cuboid, and weakness of the lateral compartment muscles and tibialis anterior.
• In cases involving a pronation sprain of the ankle joint, ‘the distal talofibular joint glides posteriorly and the head of the fibula glides anteriorly’.
• In cases of supination ankle sprain, ‘the distal fibula is often found to be anterior and the fibular head is posterior’ (Kuchera & Goodridge 1997).
Testing joint play and mobilizing the proximal tibiofibular joint
• The patient is supine with hip and knee flexed so that the sole of the foot is flat on the table.
• The practitioner sits so that her buttock rests on the patient’s toes, stabilizing the foot to the table.
• The head of the fibula is grasped between thumb and index finger of one hand as the other hand holds the tibia firmly, inferior to the patella.
• Care should be taken to avoid excessive pressure on the posterior aspect of the fibula head as well as its neck, as the common fibular nerve (relatively exposed) wraps around it and is vulnerable to compression (Kuchera & Goodridge 1997) (see Fig. 14.2C).
• The thumb resting on the anterior surface of the fibula should be reinforced by placing the thumb of the other hand over it.
• A movement that takes the fibular head firmly posteriorly and anteriorly, in a slightly curved manner (i.e. not quite a straight backward-and-forward movement, but more back and slightly curving inferiorly, followed by forward and slightly curving superiorly, at an angle of approximately 30° – see Fig. 14.3), determines whether there is freedom of joint glide in each direction.
• If restriction is noted in either direction, repetitive rhythmical springing of the fibula at the end of its range should restore normal joint play.
• It is worth noting that when the fibular head glides anteriorly there is automatic reciprocal movement posteriorly at the distal fibula (lateral malleolus), while posterior glide of the fibula head results in anterior movement of the distal fibula. Restrictions at the distal fibula are, therefore, likely to influence behavior proximally and vice versa.
• Petty (2006) utilizes a similar anteroposterior glide to that described above, but suggests a prone position for the posteroanterior assessment.
• The prone patient’s leg is supported proximal to the ankle on a cushion, so that the knee is in slight flexion.
• The practitioner’s thumbs are applied to the posterior aspect of the fibula head while avoiding the common fibular nerve, with fingers curved around the proximal leg to offer support for the hands as well as to stabilize the leg.
• On-and-off combined thumb pressure is applied to assess anterior glide potential of the head of the fibula.
Entrapment possibility
As observed above, care is required to avoid undue pressure on the posterior aspect of the fibula head due to neural structure proximity. Kuchera & Goodridge (1997) point out additionally that dysfunction that involves the fibular head being locked in a posteriorly translated direction ‘may cause symptoms related to entrapment neuropathy or compression of the common peroneal [fibular] nerve’ (see Box 14.9).
Box 14.9 Neural impingement and neurodynamic testing
Korr (1970, 1981) demonstrated that nerves transport vital biochemical substances throughout the body, constantly. The rate of axonal transport of such substances varies from 1 mm/day to several hundred mm/day, with ‘different cargoes being carried at different rates’. ‘The motor powers (for the waves of transportation) are provided by the axon itself’. Transportation is a two-way traffic, with retrograde transportation, ‘a fundamental means of communication between neurons and between neurons and non-neuronal cells’.
Korr (1981) believes this process to have an important role in maintenance of ‘the plasticity of the nervous system, serving to keep motor-neurons and muscle cells, or two synapsing neurons, mutually adapted to each other and responsive to each other’s changing circumstances’. The trophic influence of neural structures on the structural and functional characteristics of the soft tissues they supply can be shown to be vulnerable to disturbance. Korr (1981) explains:
Maitland (1986) suggested that assessment and treatment of ‘adverse mechanical tension’ (AMT) in the nervous system should be seen as a form of ‘mobilization’.
Chemical or inflammatory causes of neural tension can also occur, resulting in ‘interneural fibrosis’, which leads to reduced elasticity and increased ‘tension’, which would become obvious with tension testing of these structures (see the discussion of Morton’s neuroma on p. 532). The pathophysiological changes resulting from inflammation, or from chemical damage (i.e. toxicity) lead to internal mechanical restrictions of neural structures, which are quite different from externally applied mechanical causes, such as would be produced by a disc lesion, for example.
Petty (2006) suggests that in order to ‘ascertain the degree to which neural tissue is responsible for the production of the patient’s [ankle and foot] symptoms’ the tests that should be carried out are passive neck flexion, straight leg raising, passive knee flexion and slump. These tests are described below, with the exception of passive neck flexion, which is self-explanatory.
• A positive tension test is one in which the patient’s symptoms are reproduced by the test procedure and where these symptoms can be altered by variations in what are termed ‘sensitizing maneuvers’, which are used to ‘add weight to’, and confirm, the initial finding of AMT.
• Adding dorsiflexion during SLR is an example of a sensitizing maneuver.
• Precise symptom reproduction may not be possible, but the test is still possibly relevant if other abnormal symptoms are produced during the test and its accompanying sensitizing procedures. Comparison with the test findings on an opposite limb, for example, may indicate an abnormality worth exploring.
• Altered range of movement is another indicator of abnormality, whether this is noted during the initial test position or during sensitizing additions.
Variations of passive motion of the nervous system during examination and treatment
1. An increase in tension can be produced in the interneural component, where tension is being applied from both ends, so to speak, as in the ‘slump’ test (Fig. 14.45).
2. Increased tension can be produced in the extraneural component, which then produces the maximum movement of the nerve in relation to its mechanical interface (such as in straight leg raising) with the likelihood of restrictions showing up at ‘tension points’.
3. Movement of extraneural tissues in another plane can be engineered.
CAUTION: General precautions and contraindications
• Care should be taken of the spine during the ‘slump test’ if disc problems are involved or if the neck is sensitive (or the patient is prone to dizziness).
• If any area is sensitive, care should be taken not to aggravate existing conditions during performance of tests.
• If obvious neurological problems exist, special care should be taken to avoid exacerbation that vigorous or strong stretching might provoke.
• Similar precautions apply to diabetic, MS or recent surgical patients or where the area being tested is affected by circulatory deficit.
• Tests of the sort described below should be avoided if there has been recent onset or worsening of neurological signs or if there is any cauda equina or cord lesion.
Straight leg raising (SLR) test
Note: See text relating to hamstring tests (for shortness) in Chapters 10 and 12. See Figure 12.46 in particular. In Chapter 10 Box 10.5, see discussion under the subheading: Protocol for assessment of symptoms caused by nerve root or peripheral nerve dysfunction (p. 237).
The leg is raised in the sagittal plane, knee extended.
Sensitizing additions to SLR
• Ankle dorsiflexion (this stresses the tibial component of the sciatic nerve).
• Ankle plantarflexion plus inversion (this stresses the common fibular nerve, which may be useful with anterior shin and dorsal foot symptoms).
• Increased medial hip rotation.
• Altered spinal position (for example, left SLR may be ‘sensitized’ by lateral flexion to the right of the spine).
Notes on SLR test
• During the SLR test there is caudad movement of the lumbosacral nerve roots in relation to interfacing tissue (which is why there is a ‘positive’ indication of pain and limitation of leg-raising potential when SLR is performed in the presence of a prolapsed intervertebral disc).
• The tibial nerve, proximal to the knee, moves caudad (in relation to the mechanical interface) during SLR, whereas distal to the knee it moves cranially. There is no movement of the tibial nerve behind the knee itself, which is therefore known as a ‘tension point’.
• The common fibular nerve is attached firmly to the head of the fibula (another ‘tension point’).
Prone knee bend test (PKB)
• The patient is prone and the knee is flexed, taking the heel toward the buttock to assess reproduction of existing symptoms, or other abnormal symptoms, or altered range of movement (heel should approximate buttock easily). See Figure 12.18 for positioning in prone position.
• During the test, the knee of the prone patient is flexed while the hip and thigh are stabilized, which moves the nerves and roots from L2, L3, L4 and, particularly, the femoral nerve and its branches.
• If, however, the test is conducted with the patient sidelying, the hip should be maintained in extension during the test (this alternative position is thought more appropriate for identifying entrapped lateral femoral cutaneous nerve problems).
• It is obvious that the PKB test stretches rectus femoris and rotates the pelvis anteriorly, thus extending the lumbar spine, which can confuse interpretation of nerve impingement symptoms. Care should be taken to avoid this by stabilizing the pelvis or by placement of a pillow under the abdomen to support the lumbar spine. A bodyCushion™ would most ideally achieve this goal.
• Sensitizing maneuvers include (in either prone or sidelying use of the test):
The ‘slump test’
Butler (1994) regards this as the most important test in this series. It links neural and connective tissue components from the pons to the feet and requires care in performance and interpretation (Fig. 14.45). The slump test is suggested for all spinal disorders, most lower limb disorders and some upper limb disorders (those that seem to involve the nervous system).
The test involves the seated patient introducing the following sequence of movements:
• thoracic and then lumbar flexion, followed by
• sometimes also hip flexion (produced by either bringing the trunk forward on the hips or by increasing SLR).
Additional sensitizing movements during slump testing are achieved by changes in the terminal positions of joints. Butler (1994) gives examples.
• If the test reproduces (for example) lumbar and radiating thigh pain, a change in head position – say into slight extension – could result in total relief of these symptoms (desensitizing).
• A change in ankle and knee positions could significantly change cervical, thoracic or head pain produced by the test.
• In both instances this would confirm that AMT was operating, although the site would remain obscure.
• Trunk sidebending and rotation or even extension, hip adduction, abduction or rotation and varying neck positions are all sensitizing movements.
Cadaver studies demonstrate that neuromeningeal movement occurs in various directions, with C6, T6 and L4 intervertebral levels being regions of constant state (i.e. no movement, therefore ‘tension points’). Butler (1994) reports that many restrictions identified during the slump test may only be corrected by appropriate spinal manipulation.
• Hamstring and posterior knee pain occurring with trunk and neck flexion, when the knees are extended and increasing further with ankle dorsiflexion.
• Restrictions in ankle dorsiflexion during trunk/neck flexion while the knee is in extension.
• There is a common decrease in pain noted on release of neck flexion and an increase in range of knee extension or ankle dorsiflexion on release of neck flexion.
Butler (1994) suggests that in treating adverse mechanical tensions in the nervous system, initial stretching of the tissues associated with neural restrictions should commence well away from the site of pain in sensitive individuals and conditions. It is not within the scope of this text to detail methods for releasing abnormal tensions, except to suggest that the treatment positions are commonly a replication of the test positions (as in shortened musculature, where MET is used).
Mobilization with movement (MWM) to release the fibula head
• The patient is lying or standing.
• The practitioner applies anterior or posterior pressure to the fibula head (with thumb pressure toward the direction of joint play restriction), as the patient actively, slowly, flexes and extends the knee several times.
• If the pain-free range is increased during the exercise, the indication is that the problem is of a mechanical nature at the proximal tibiofibular joint and this procedure may well normalize it (Mulligan 1999, Petty 2006). Due to their reciprocal movements, the distal tibiofibular joints should also be accessed.
MET for releasing restricted proximal tibiofibular joint
For posterior fibular head dysfunction (where anterior glide is restricted)
• The patient sits on the treatment table with legs hanging over the edge.
• The practitioner sits in front of the patient supporting the foot with one ‘supporting’ hand
• The practitioner’s other hand engages the posterior aspect of the fibular head and introduces an anteriorly directed force, while avoiding compression of the fibular nerve.
• At the same time the supporting hand passively inverts, plantarflexes and adducts the foot (creating supination), to the first resistance barriers in these directions.
• When slack has been removed via these movements, the patient is asked to evert, abduct and dorsiflex the foot, using a moderate degree of effort (‘Try to use no more than 25% of your strength, while I resist your effort’).
• This isometric effort is held for 5–7 seconds (Greenman [1996] suggests just 3–5 seconds).
• Following complete relaxation of the muscular effort by the patient, slack is removed by the contacts on the fibular head and also the foot, as a new barrier is engaged (i.e. increased inversion and internal rotation) and the process is repeated once or twice more.
• According to Goodridge & Kuchera (1997) the muscles that are likely to be involved in this resisted isometric effort include extensor digitorum longus and tibialis anterior. The sustained contraction should ‘draw the fibula anteriorly along the tibial articular surface’. Additionally, the isometric action of these muscles should inhibit their antagonists, which may be holding the fibular head posteriorly (see discussion of muscle energy technique in Chapter 9).
For anterior fibular head dysfunction (where posterior glide is restricted)
• The patient sits on the treatment table with legs hanging over the edge.
• The practitioner sits in front of the patient supporting the foot with one hand
• The practitioner’s other hand engages the anterior aspect of the fibular head and introduces a posteriorly directed force.
• At the same time the supporting hand passively inverts and dorsiflexes the foot.
• When slack has been removed via these movements, the patient is asked to evert and plantarflex the foot, using a moderate degree of effort (‘Try to use no more than 25% of your strength, while I resist your effort’).
• This isometric effort is held for 5–7 seconds (Greenman [1996] suggests just 3–5 seconds).
• Following complete relaxation of the muscular effort by the patient, slack is removed by the contacts on the fibular head, and also the foot, as a new barrier is engaged (i.e. increased inversion and dorsiflexion) and the process is repeated once or twice more.
• The muscles that are likely to be involved in this resisted isometric effort include fibularis (peroneus) longus, ‘to draw the fibula laterally from the tibia, making posterior gliding easier’ and soleus, ‘to draw the fibula posteriorly along the tibial articular surface’ (Goodridge & Kuchera 1997). Additionally, the isometric action of these muscles should inhibit their antagonists, which may be holding the fibular head anteriorly (see discussion of MET in Chapter 9).
The ankle joint and hindfoot
The ankle joint composes of the malleoli of the tibia and fibula, the distal surface of the tibia and the body of the talus (see Figs 14.1, 14.2, 14.4). The tibia is weight-bearing onto the head of the talus, while the fibula has very little weight-bearing responsibility, with ‘no more than 10% of the weight that comes through the femur being transmitted through the fibula’ (Levangie & Norkin 2005).
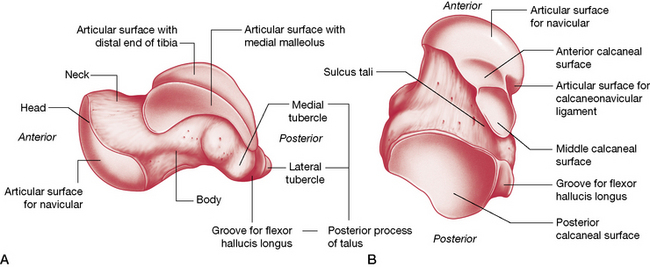
Figure 14.4 The (A) medial and (B) posterior aspects of the talus.
(Reproduced, with permission, from Gray’s anatomy for students, 2nd edn, 2010, Churchill Livingstone).
The tibiofibular component supplies three facets, which together form an almost continuously concave surface, resembling an adjustable mortise (similar to an adjustable wrench). Levangie & Norkin (2005) observe:
The proximal head of the talus is a wedge-shaped structure, wider anteriorly than posteriorly, that is held in an arch (mortise) created by the medial (tibial) and lateral (fibular) malleoli. Approximately one-third of the medial aspect of the talus is bounded by the tibial malleolus, while the lateral aspect of the talus is entirely bounded by the fibular malleolus, which is more posteriorly situated when compared to the tibial malleolus. The relative oblique axis between the malleoli results in ‘a toeing out (by about 15°) of the free foot…with dorsiflexion and toeing in, with plantarflexion’. We are reminded by Goodridge & Kuchera (1997) that this position of the free foot is important ‘when setting up manipulative techniques addressing this joint’.
The tibia rests on the proximal trochlear surface of the talus. The talus projects a long neck that ends in a rounded distal head for articulation with the navicular bone and the plantar calcaneonavicular ligament, and it offers a facet for each of the malleoli and three articulations with the calcaneus (Fig. 14.4).
The talus has no direct muscular attachments so its ligamentous structure is significant (see below). Its movements are influenced by muscular action on bones that lie above and below it (Greenman 1996). Because of the strong ligaments of the ankle, the shape of the crural concavity and the length of the lateral malleolus on the talus, joint dislocation is extremely unlikely unless accompanied by fracture.
The ankle mortise (also called talocrural, tibiotalar or talotibiofibular joint) is designed to handle enormous degrees of force. (Figs 14.5) Gray’s anatomy (1995) reports that: Compressive forces transmitted across the joint during gait reach five times body weight while tangential shear forces, the result of internally rotating muscle forces and externally rotating inertial forces associated with the body moving over the foot, may reach 80% body weight.
Gray’s anatomy (2005) describes the ankle joint as follows:
Levangie & Norkin (2005) artfully describe the mortise:
Anterior to the malleoli on the dorsum of the talocrural joint:
Posterior to the medial malleolus (Fig. 14.6):
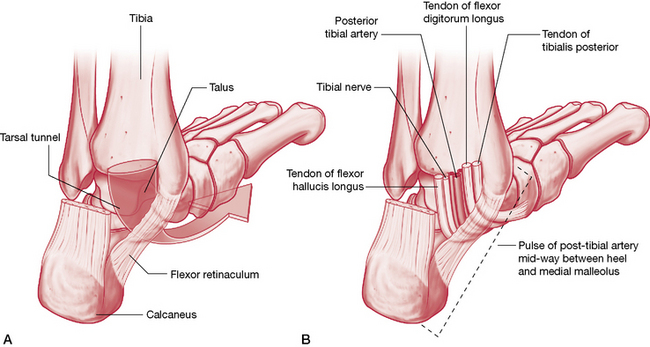
Figure 14.6 The course of the neurovascular structures beneath the medial malleolus
(Reproduced, with permission, from Gray’s anatomy for students, 2nd edn, 2010, Churchill Livingstone).
Posterior to the lateral malleolus (in a groove):
The ankle ligaments
The bones that make up the crural arch (the distal tibia and the medial and lateral malleoli) are connected to the talus by the joint capsule and powerful ligaments (Fig. 14.7ABC).
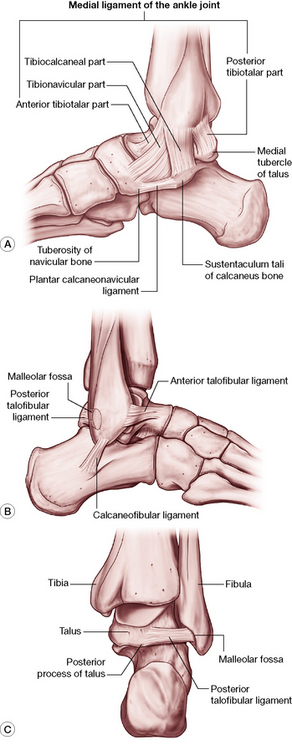
Figure 14.7 The ligaments of the A) medial ankle B) lateral ankle and C) posterior aspect of the ankle joint
(Reproduced, with permission, from Gray’s anatomy for students, 2nd edn, 2010, Churchill Livingstone).
Medial (deltoid) ligament
This extremely powerful, medially located ligament is triangular in shape with its superior attachments on the apex as well as the anterior and posterior borders of the medial malleolus. Inferiorly it has a variety of fibers and attachments, as described by Gray’s anatomy (2005).
Posterior talofibular ligament
This attaches to the lower aspect of the lateral malleolus from where it runs virtually horizontally to the lateral tubercle of the posterior talar process. Gray’s anatomy (2005) reports that: ‘A “tibial slip” of fibres connects it to the medial malleolus’.
Movements of the ankle joint
Kuchera & Goodridge (1997) suggest that the ankle joint is in fact two joints, which should be considered together as a functional unit: the talocrural joint (ankle mortise) and the subtalar joint (described below). They point to the research of Inman (1976) who showed that during the gait cycle, as weight is taken on the foot, there is ‘visible medial rotation of the tibia [that] is greater than can be attributed to movement solely at the talocrural joint’. Inman demonstrated that the increased tibial rotation resulted from ‘relative calcaneal eversion about the subtalar axis’. As the stance phase progresses the tibia then externally (laterally) rotates, at the same time as calcaneal inversion occurs, again about the subtalar axis (see Box 14.1).
The motions of the ankle joint are as follows.
• Plantarflexion (up to 50°, if motion is not isolated and includes subtalar or transverse tarsal joint excursion) achieved by soleus and gastrocnemius, assisted by plantaris, fibularis (peroneus) longus and brevis, tibialis posterior, flexor digitorum longus and flexor hallucis longus.
• Dorsiflexion (10° with knee straight, 30° with knee flexed) achieved largely by tibialis anterior, extensor digitorum longus and fibularis (peroneus) tertius, assisted by extensor hallucis longus (Schiowitz 1991, Travell & Simons 1992).
• Accessory minor motions of anterior glide with plantar flexion and posterior glide with dorsiflexion (Goodridge & Kuchera 1997).
• Additionally, Kuchera & Goodridge (1997) note that: ‘Plantar flexion is accompanied by adduction and supination of the foot….[and]…the proximal fibular head glides posteriorly and inferiorly…[and]…the talus glides anteriorly, placing the narrow position of the talus in the ankle mortise, a less stable position’.
• Although it appears to be simply a hinge, during plantarflexion it is dynamic and can shift (Gray’s anatomy 2005). Platzer (2004) notes that in plantarflexion (open-packed position), some side-to-side movement is possible.
• Stability during symmetrical standing requires continuous action by soleus, which increases during forward leaning (often involving gastrocnemius) and decreases with backward sway. If a backward movement takes the center of gravity posterior to the transverse axes of the ankle joints, the plantarflexors relax and the dorsiflexors contract (Gray’s anatomy 2005).
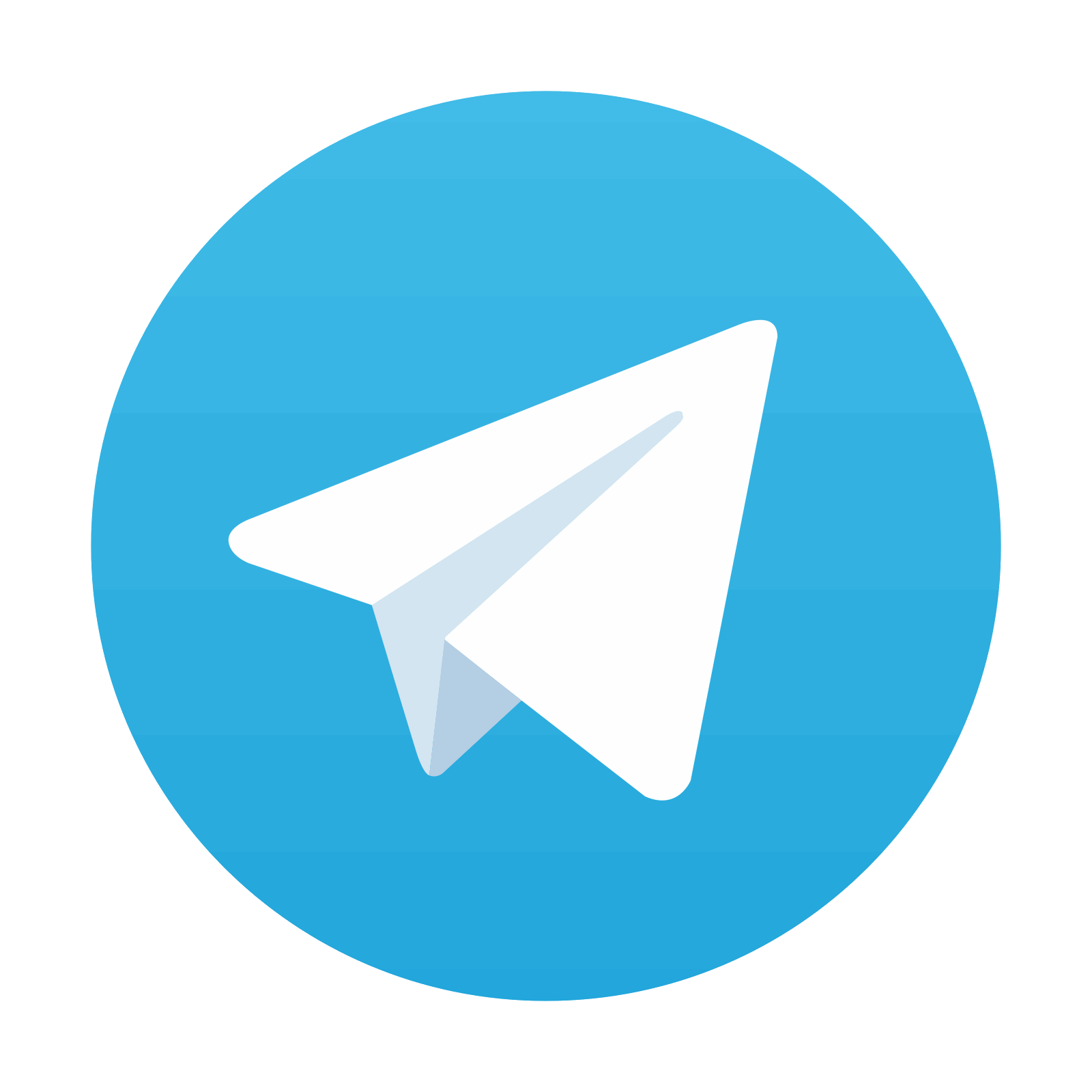
Stay updated, free articles. Join our Telegram channel

Full access? Get Clinical Tree
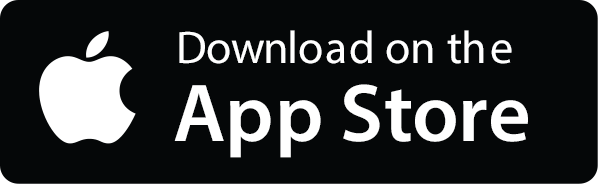
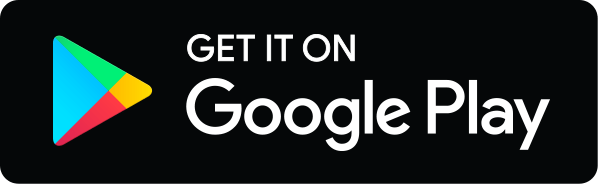