Abstract:
This chapter is intended to continue to advance our understanding and respect for this limbic system, which is a complex system integrated in ways that its contribution is considered within the evaluation of every patient, regardless of setting or diagnosis. The first section of the chapter includes an overview of the limbic network and a discussion of limbic behavior and how to begin to differentiate true motor responses from those entangled in limbic interactions. The second section delves into the anatomy and physiology of the limbic network, the biology of learning and memory, neurochemistry, and neuroplasticity. The third section applies our understanding of the limbic network to our patient assessments, treatment, environment, and interactions. In the last section, current advances and future research possibilities in the role of the limbic network and its impact on the professional roles of physical and occupational therapists are explored.
Keywords:
amygdala, declarative memory and learning, emotional behavior, F2ARV (fear and frustration, anger, rage, violence/withdrawal) continuum, General Adaptation Syndrome (GAS), hippocampus, hypothalamus, limbic network, limbic neutral state, motor learning, MOVE (motivation or memory, olfaction, visceral, autonomic nervous system, emotional), procedural memory and learning, therapeutic alliance, therapeutic environment
Objectives
After reading this chapter the student or therapist will be able to:
- 1.
Describe the structures of the limbic network.
- 2.
Understand the complexity of the limbic network and the influence of the limbic network on both behavior and function.
- 3.
Describe the behavioral responses directly and indirectly influenced by the limbic network.
- 4.
Identify signs of both positive and negative limbic network influence on observable behavior, motor learning, neuroplasticity, and functional responses.
- 5.
Identify patient populations with the potential for negative limbic network influence over observable behavior, motor learning, and ultimately functional outcome.
- 6.
Differentiate between limbic-driven motor control responses and frontal, cerebellar, and basal ganglia motor regulation of motor responses.
- 7.
Describe the role of the limbic network on both declarative and procedural learning.
- 8.
Describe physical therapy examination procedures or tests and measures that could identify the presence of limbic network involvement requiring intervention and establish a responsive intervention baseline.
- 9.
Describe interventions or program modifications with potential to improve motor learning through integration of limbic network treatment techniques into current intervention models.
- 10.
Understand the influence of the therapist over the limbic network and behavioral and functional responses.
Introduction
Our understanding and respect for the limbic network has expanded since the publication of the sixth edition, particularly when analyzing emotions, behavior, learning, and their influence on activities and participation in normal personal and societal roles. At no time has this understanding been more critical, with the prevalence of known mental health challenges faced within our country. In the United States, 18.3%, or 44.7 million adults, experience mental illness (AMI) or a mental, behavioral, or emotional disorder each year. This represents approximately one in five adults, which theoretically could reflect one to two patients of a given therapist’s treatment load per day. Beyond those mental health disorders that can be considered functional disorders of the limbic system, the prevalence of key central nervous system (CNS) disorders, in which the structures of the limbic network are involved, is also high, including patients with acquired brain injury (ABI, such as concussion or traumatic brain injury [TBI]), cerebrovascular accident (CVA), tumors, inflammatory disorders, Alzheimer disease, Parkinson disease, and vestibular disorders. Clearly, the prevalence of coincident limbic system impairment in our patients may therefore be substantial within the practice of any physical therapist.
This chapter has been prominently positioned within this textbook on basic neurological rehabilitation. Traditionally, the limbic system may have only been discussed within a basic science course of neuroanatomy and neurophysiology. In other curricula, the role of the limbic system in declarative memory and emotional responses may be presented as part of a discussion on behavior or cognitive function within a psychology course. Yet today, motivation and attention are stressed and accepted as key factors in neuroplasticity and motor learning.
Therapists are familiar with the need to prioritize primary body system impairments as they relate to a movement disorder. Moreover, they are familiar with the need to individualize treatment to the primary impairment(s) identified through the evaluation process. However, they are less comfortable with those “other systems” encountered as Doctors of Physical Therapy or Occupational Therapy in their role as functional diagnosticians. The profession’s acceptance of the foundational, integrated role of the cardiopulmonary system to the function of all other systems has advanced, and the acceptance of the role of the limbic system(s) needs to be similarly advanced, given the current evidence illuminating the role of the limbic network in motor output, control, and learning. Coincident is the opportunity to identify, develop, and validate requisite screening and examination tools that help physical therapists to determine the state of the limbic network and its implications for diagnosis, prognosis, and treatment planning. This chapter is intended to continue to advance our understanding of and respect for this complex, integrated system, such that its contribution is considered within the evaluation of every patient regardless of setting or diagnosis.
This chapter is designed to address this opportunity regardless of experience, from the student learner to the more seasoned clinician. For the student learner, the first section of the chapter is an overview of the limbic network and a discussion of limbic behavior and how to begin to differentiate true motor responses from those entangled in limbic interactions. For the therapist learner desiring ongoing clinical mastery, the second section delves into the anatomy and physiology of the limbic network and the the biology of learning and memory, neurochemistry, and neuroplasticity. The third section discusses the immediate relevance of this material to both the student and the practicing clinician—how can we apply our understanding of the limbic network to our patient assessments, treatment, environment, and interactions? In other words, how might it change what we do as rehabilitation professionals “come Monday morning”? And finally, in the last section, current advances and future research possibilities in the role of the limbic network and its impact on the professional roles of physical and occupational therapists are explored.
The limbic network
Our discussion must begin with a working definition of the limbic network and an overview of its function. It is not easy to find a generally accepted definition of the “limbic network or complex,” its boundaries, and the components that should be included. Mesulam likens this to a 5th century BC philosopher’s quotation: “the nature of God is like a circle of which the center is everywhere and the circumference is nowhere.” Brodal suggests that functional separation of brain regions becomes less clear as we discover their interrelatedness through continuing research. He sees the limbic network reaching out and encompassing the entire brain and all its functional components and sees no purpose in defining subdivisions. Although the anatomical descriptions of the limbic network may vary from author to author, the functional significance of this system is widely acknowledged in defining human behavior and behavioral neurology.
Morgane and colleagues describe the limbic network as both an “anatomical entity as well as a physiological concept.” Historically termed a limbic system, more current evidence suggests it to Limbic network comprises several emotional, behavioral, learning, and memory neuroanatomical subsystems. The term “limbic cortex ” refers to only an anatomical part of the network and the two C-shaped gyri surrounding the corpus callosum, specifically comprising the cingulate gyrus, the parahippocampal gyrus, and the olfactory lobe.
Brooks divided the brain anatomically and functionally into a limbic brain and a nonlimbic somatomotor brain, Fig. 4.1 with the somatomotor portion involved in both the perception of afferent sensory input and the generation of efferent motor output. Historically, the limbic brain was seen as primitive and essential for survival, sensing the “need” to act. Today, the limbic network is considered in evolutionary terms as an older brain system that provides fundamental support (alertness, motivation, memory, and learning) to enable the phylogenetically newer brain systems to regulate more complex behaviors, movement, and function. Thus the overall purpose of the limbic network is to initiate need-directed motor activity for survival based on experience, through its neuronal connections up to the frontal lobe (intentional responses) or down to the brain stem (automatic responses), thus regulating motor output.

We will discuss the neuroanatomy and neurobiology as they relate to both function and dysfunction of the limbic network later in this chapter, but briefly outline the major interacting systems, structures, and neurotransmitters here. The limbic network can generally be thought to include two system divisions: the prefrontal-limbic “forebrain” system (emotional and behavioral) and the limbic midbrain (motor control and movement). The major structures comprising the network include the limbic cortex (anterior cingulate cortex/gyrus [ACC]), parahippocampal gyrus, and olfactory lobe), the prefrontal cortex, amygdalar complex, limbic thalamus, stria medullaris (epithalamus), hippocampus, nucleus accumbens (NAc), the ventral tegmental area (VTA), and midbrain raphé nuclei of the basal ganglia. These structures are primary components of the network as well as essential neuromodulators upon the network. As one example, the ACC, amygdala, and hippocampus serve to connect the limbic network and the prefrontal cortex, while the network is modulated by the hypothalamus, basal ganglia, and midbrain. Given the broad structural components, the neurotransmitters of the system are also extensive and include serotonin, norepinephrine (NE), dopamine, and acetylcholine. As one major example, dopamine plays a critical role in the motivation to move as well as motor function ( Fig. 4.2 ). ,

This summary does not account for the direct and indirect influences of the limbic network upon other body systems. In the past, review of the literature on the limbic network was limited to investigating potential interactions of other systems with nuclei within the limbic network. This is no longer the case, as neuroscience research has helped to identify the critical nature of behaviors modulated or influenced by the limbic network. Based on research at a cellular level, a consciousness level, a bodily systems level, and a quantum level it is now clear that many systems are affected by the complex limbic network, and most notably, the motor systems. A patient example of the complex and critical interactions between the limbic network, body systems, and its influence upon human behavior can be considered in Clinical Case 4.1 .
A patient example of limbic interactions can be found in a case description of a middle-aged woman admitted to the intensive care unit (ICU) with multiple pelvic fractures and diagnosed with severe internal bleeding, kidney failure, pneumonia, pulmonary emboli, and severe clotting in the lower extremities. The physician took her husband aside to let him know that she was going to die, to which her husband replied, “I understand. Juggling one system problem is easy, juggling two systems takes a little practice, and three-system involvement may challenge the best medical skill. She is presenting four or five body system failures, and you are sure no one can juggle that many problems.” The doctor said yes, and the patient’s husband then said, “Please keep juggling and don’t worry about me, because if you do, I would then be one more ball to juggle.” And it did seem that every time the doctors got a handle on a body system problem, another system would fail. She required services from an endocrinologist, infection control specialists, interventional radiologists, a pulmonologist, a hematologist, a vascular surgeon, an internal medicine specialist, a urologist, and a nephrologist. Each specialist shared his or her limited experience with a complex clinical problem like this and that there was nothing in the literature to help his or her respective understanding. After 2{1/2} months in the ICU, the woman survived. The physician who had foretold her death met again with the patient and her family. He stated, “How are you still alive? I know what we did medically, but that was not enough to keep you alive.” And he was right. No model within each respective field could account for her recovery. However, the piece not considered within her medical management was the beliefs and spiritual strength of the patient and her family, a positive limbic network influence on the function of each failing body system.
The limbic network “MOVEs” us
Moore eloquently describes the limbic network as the area of the brain that moves us. The word MOVE can be used as a mnemonic for the functions of the limbic network that drive movement. As seen in Fig. 4.3 the M (motivation, memory) depicts the drive component of the limbic network; the O (olfaction) refers to the sense of smell and of other sensory inputs that drive motor, emotional, or visceral responses; the V (visceral) outlines the visceral and autonomic responses that serve as an indicator of the limbic network state; and E (emotional), the value or significance of individual feelings, attitudes, and beliefs that possess emotional significance for attention and learning.

Motivation
“The most powerful force in rehabilitation is motivation.” Without motivation the individual will not attend to the task and the neurobiology of learning will not occur. Motivation drives our motor system to develop motor programs that will enable us to perform and execute movements with the most efficient patterns available. In addition, motivation drives our ability to integrate the somatosensory map of functional skills (cortical) and attention (limbic) necessary to process both sequential and simultaneous components (parts) of the movement task to be learned (whole).
Moore considers motivation and memory as part of the MOVE system. Esch and Stefano link motivation with reward, illustrating how the limbic network “learns” through repetition and reward. They state that the concept of motivation includes drive and satiation, goal-directed behavior, and incentive. They recognize that these behaviors maintain homeostasis and ensure the survival of the individual and the species. Motivation mediated by limbic (forebrain) and subcortical structures forms an interlocking neural network with the cognitive representation within the frontal regions and thus plays an important role in movement execution. ,
Memory
The brain stores sensory and motor experiences as memory. Current theory supports a “dual memory system,” each utilizing different pathways in the nervous system. Terms such as verbal and nonverbal and intrinsic and extrinsic have been given to these two memory systems. For this discussion, two specific categories of learning— procedural and declarative —will be used, although in today’s neuroscience environment, the terms implicit and explicit memory are used as frequently. The limbic network plays a key role in both declarative and procedural aspects of memory and learning. Before the limbic network’s impact on learning and memory can be delved into, a clear understanding of what is meant by these functions is needed. Declarative (explicit) memory entails the capability to recall and verbally report experiences. This recall requires deliberate conscious effect, whereas the procedural counterpart is the recall of “rules, skills, and procedures (implicit),” which can be recalled unconsciously. Both categories of learning have been correlated to limbic function. These systems do not operate autonomously, and many therapeutic activities seem to combine these memory systems to achieve functional behavior. The limbic amygdala and hippocampal structures and their intricate circuitries play a key role in the declarative aspect of memory. The hippocampus may be more concerned with sensory and motor signals relating to the external environment, whereas the amygdala is related more to those of the internal environment. They both contribute in relation to the significance of external or internal environmental influences. , Procedural learning is vital to the development of sequence-specific learning and motor control. Acquisition and learning (memory) of new motor tasks involves dopaminergic projections from the VTA to the primary motor cortex for motor execution and initiating neuroplastic change, and from the NAc for the reward and reinforcement of that motor learning. The dorsal prefrontal cortex and the ACC are more active in the skill acquisition phase of motor learning than in the execution of pre-learned motor skills, consistent with the need for motivation as a requisite. Consolidation and retention occur as information passes through the limbic network nuclei; it is stored in cortical areas and retention and retrieval occurs without limbic involvement. ,
Olfaction
The O refers to olfaction, or the incoming sense of smell, which exerts a strong influence on alertness and drive. This is clearly illustrated by the billions of dollars spent annually on perfumes, deodorants, mouthwashes, and soap as well as scents used in stores to increase customers’ motivations to purchase. Olfactory input first synapses within the olfactory bulb. Subsequently, afferents travel to and synapse within the cerebral cortex without second-order synapse in the thalamus, as well as with structures within the limbic network. Although collaterals do project to the thalamus, unlike all other sensory afferent information, olfaction does not need to use the thalamus as a relay center to access the cortical structures. , Olfactory input that enters the limbic network may be used to calm or to arouse the patient, based upon the specific odor selected; for example, the use of aromatherapy to promote muscle relaxation prior to therapeutic massage. , Thus olfaction indirectly influences motor output via the limbic network’s influence on muscle tone through brain stem modulation of the indirect or “emotional” motor pathways. Through brain stem connections to the reticular activating system, olfaction can have a powerful effect upon alertness. The evaluation of this system seems even more critical when a patient’s motor control system is locked, with no volitional movement present. Research has shown that retrieval processing and retrieval of memory have a distinctive emotionality when they are linked to odor-evoked memories. ,
Beyond olfaction, input from the other senses may not be reaching the cortical levels, such as in patients with lesions in the internal capsule or thalamus, and the patient may have a sensory-deprived environment. If the sensory input to the patient is deprived, the limbic network may place the patient in a “limbic low” state and the patient may withdraw physically or mentally, lose focus or attention, decrease motivation, and demonstrate a decrease in motor tone and output. Conversely, if the sensory input to the patient is excessive, whether through internal (patient/systems generated) or external (therapist, environment generated) feedback, the limbic network may go into an alert, protective mode, or “limbic high” state. The patient may withdraw physically or mentally, lose focus or attention, decrease motivation, and become frustrated or even angry. The resultant overload on the reticular system may be the reason for the shutdown of the limbic network versus dysfunction of the limbic network itself. Both are part of the same neuroloop circuitry and as such, in either situation, the CNS will not function optimally and learning will diminish. All these behaviors may be expressed within the hypothalamic-autonomic system as motor output, no matter where in the loop the dysfunction occurs. Having a functional understanding of the neuroanatomical sectors and their relationships with each other helps therapists unravel some of the challenging patient presentations after CNS insult. ,
Therapists often try to increase motor activity through sensory input; however, they must cautiously avoid indiscriminately bombarding the sensory systems. The limbic network may demonstrate overload from stimulation at the same time the spinal motor generators reflect inadequate activation. One way that a therapist might assess for potential limbic overload would be to closely monitor the autonomic nervous system (ANS) responses, such as blood pressure, heart rate, internal temperature, and sweating, versus observing or measuring muscle tone.
Visceral or autonomic
The V represents visceral or autonomic drives to movement. At the heart of the limbic network is the hypothalamus, the primary regulator of autonomic and endocrine functions. The hypothalamus controls and balances homeostasis of the internal and peripheral (skin) organ systems through regulation of sympathetic and parasympathetic reactions. Protective drives such as thirst, hunger, temperature regulation, and sexuality are controlled by this system. However, the hypothalamus is also an important modulator of the limbic network, given its direct connections with the limbic midbrain, the amygdala, and hippocampus, as well as close reciprocal interaction with most centers of the cerebral cortex, brain stem, and spinal cord. As such, the fact that autonomic and somatomotor responses controlled by the hypothalamus are closely aligned with the expression of emotions and strong emotions and emotionally linked memories can change or support movement has clearly been established.
Of clinical importance is that autonomical responses may reflect the presence of a limbic network imbalance that may go unnoticed by therapists. For example, patients with unstable body temperature regulation may exhibit signs of hypothalamic-pituitary involvement and overactivity. Similarly, severe sweating of the palms or increased oral motor activity may be the first sign that the stress of an activity is becoming overwhelming to a patient. A therapist must continually monitor the patient’s response behaviors to ascertain that they reflect true or desired motor output and not limbic influences over the motor system.
Emotional
E relates to emotions: the feelings, attitudes, and beliefs that are unique to that individual. These beliefs include psychosocial attitudes and prejudices, ethnic upbringing, cultural experiences, religious convictions, and concepts of spirituality. When stimuli or tasks are endowed with emotional value or significance, a patient’s attention is drawn to those with emotional significance, selecting these for attention and learning. Emotionally charged events will leave a more significant impression and enhance subsequent recall. All these aspects of emotions are strongly linked to the amygdaloid complex of the limbic network, and activity within the frontal lobe. , , As a primary emotional center, it regulates not only our self-concept but our attitudes and opinions toward our external environment and the people within it. One aspect of self-concept is the emotional component of body image. A second self-concept deals with the patient’s perceived worth or value to society, the world, and their role within it. Both of these attitudes may be changed through enhanced mood or positive experience, enabling emotions to influence what is perceived and learned via the amygdala’s reciprocal connections with the cortex.
Preconceived attitudes, social behaviors, and opinions have been learned by filtering the input through the limbic network. If new attitudes and behaviors need to be learned after a neurological insult, the status of the amygdalar pathways seems crucial. Damage to these limbic structures may prevent learning ; thus socially maladaptive behavior may persist, making the individual less likely to adapt to the social environment. It is often harder to change learned social behaviors than any other type of learning. Because our feelings, attitudes, values, and beliefs drive our behaviors through both attention and motor responses, the emotional aspect of the limbic network has great impact on our learning and motor control. If a patient is not motivated to participate and places little value on a motor output, then low complacency results, and little learning will occur. On the other hand, if a therapist places an extremely high value on a motor output as the expression of motor control success without interlocking that with the patient’s sense of control, the behavioral response (limbic influence) may lead to inconsistency, lack of compliance/participation, and thus lack of motor learning and carryover. In addition, it can cause the patient significant stress, which can prevent recovery and in some cases, cause disease.
Repeated experience of reinforcement and reward leads to learning, changed expectancy, changed behavior, and maintained performance. Emotional learning through the limbic network is very hard to unlearn once the behavior has been reinforced over and over. , For that reason, motor behavior that is strongly linked to a negative emotional response might be a very difficult behavior to unlearn. Repetition of motor performance with either the feeling of emotional neutrality or the feeling of success (positive reinforcement) is a critical element in the therapeutic setting. Consistently making the motor task more difficult just when the patient feels a need to succeed may decrease positive reinforcement or reward, lessen the patient’s motivation to try or participate, and decrease the probability of true independence once the patient leaves the clinical setting. When pressure is placed on therapists to produce changes quickly, strategic and meaningful repetition and thus long-term learning are often jeopardized. Rapid progression based solely upon therapist/clinic requirements may impede progress and yield a dramatic effect on the quality of the patient’s life and the long-term treatment effects once he or she leaves the medical facility. Motor control theory (see Chapter 3 ) coincides with limbic research regarding reinforcement. Inherent feedback within a variety of environmental contexts allowing for error with correction leads to greater retention. Repetition or the opportunity to practice a task (motor or cognitive) in which the individual desires to succeed will lead to long-term learning. Without practice or motivation, the chance of successful motor learning is minimized. This continues to highlight the importance of both therapeutic environment and therapeutic alliance, which we will discuss in greater detail.
Multiple nuclei and interlinking circuits of the limbic network play crucial roles in behavioral and emotional changes and declarative memory. , The loss of any link can affect the outcome activity of the whole circuit. Thus damage to any area of the brain can potentially cause malfunctions in any or all other areas, and the entire circuit may need reorganization to restore function.
The neuroscience of the limbic network
The limbic network comprises a group of nuclei, tracts, and cortical areas that lie beneath the cortex and surround the thalamic structures. All of the areas of the limbic system are interconnected with each other through both simple and complex reverberating neural pathways. There are extensive connections between the limbic system and the hypothalamus and brain stem structures, these together play a substantial role in the regulation of homeostasis and autonomic control. Similarly, there are extensive connections to the structures of the basal ganglia, thus influencing motivation to move, as well as initiation and modulation of motor output. A limited overview of the anatomy and physiology of the limbic network is presented in the following sections, designed to guide our understanding of the limbic network. The complexities of the network and its complex circuitry can be found upon deeper review of primary and secondary evidence. , ,
Neuroanatomy
Basic structure and function
Broca first conceptualized the anatomical regions of the limbic lobe as forming a ring around the brain stem. Today, neuroanatomists do not differentiate an anatomical lobe as limbic, but rather refer to a complex network that encompasses cortical, diencephalon, and brain stem structures. This description is less precise and includes (but is not limited to) the orbitofrontal and prefrontal cortex, hippocampus, parahippocampal gyrus, cingulate gyrus, dentate gyrus, amygdaloid body, septal area, hypothalamus, and some nuclei of the thalamus. , Anatomists stress the importance of looking at the interrelated structures, pathways, and functional loops within the complex limbic region. ,
The limbic network can be best visualized as consisting of both cortical and subcortical structures, with the hypothalamus located at the central position ( Figs. 4.4 and 4.5 ). The hypothalamus is surrounded by a circular alignment of the subcortical limbic structures linked with one another and the hypothalamus. These subcortical structures include the amygdalar complex and the hippocampal formation as the major input structures, and the anterior nuclei of the thalamus (limbic thalamus), as well as the NAc, the fornix, and the septal nuclei areas. The septal area projects to emotion-generating areas and plays an important role in feelings of social connectedness and bonding. The fornix contains major efferent fibers from the hippocampus, as well as carrying some afferent fibers to the hippocampus from structures in the thalamus and basal forebrain. The cingulate fasciculus carries amygdalic and hippocampal projections to and from the prefrontal cortex, linking many basic perceptual strategies, such as body schema, hearing, vision, and smell, to the emotional and learning centers of the limbic network.

The subcortical structures are again similarly surrounded by a ring of cortical structures collectively called the limbic cortex , which includes the frontal cortex, the ACC/gyrus, the parahippocampal gyrus, and the uncus. The limbic network circuitry contains parallel and reverberating connections between the subcortical and limbic cortex structures, the anterior nuclei of the thalamus (limbic thalamus) , as well as to the limbic forebrain NAc and limbic midbrain structures: VTA and midbrain raphé nuclei of the basal ganglia. There are neuroanatomists who include the olfactory system and the basal forebrain area (cognition and memory) within the network as well (see Fig. 4.5 ). Olfaction is included as part of a “mesolimbic” system, linking olfaction to the midbrain structures of the NAc and the VTA.

Vitally linked to the limbic network is the excitatory reticular activating system and other brain stem nuclei of the midbrain. Some consider components of the midbrain a very important region for emotional expression. Derryberry and Tucker found that attack behavior aroused by hypothalamic stimulation is blocked when the midbrain is damaged and that midbrain stimulation can be made to elicit “attack behavior” even when the hypothalamus has been surgically disconnected from other brain regions. Recent research has clearly identified the neurochemical precursors to this aggressive behavior. , , This “septo-hypothalamic-mesencephalic (midbrain)” continuum, connected by the medial forebrain bundle (see Fig. 4.5 ), is suggested as vital to the integration and expression of emotional behavior. The linking of other brain structures to emotions came initially from the work of Papez, who first identified the hippocampal-fornix circuit. He saw this as a way of combining the “subjective” cortical experiences with the emotional subcortical (hypothalamic) contribution. Earlier, Broca labeled the cingulate gyrus and hippocampus “circle” as “the great limbic lobe.” Today, the concept of the limbic network and its interaction with sensory inputs and motor expression has become extremely complex. Mood can change motor output, and motor activity can change mood. ,
Klüver and Bucy linked the anterior half of the temporal lobes and the amygdaloid complex to the limbic network. They showed changes in behavior, with specific loss of the amygdaloid complex and anterior hippocampus input, resulting in (1) restless overresponsiveness, (2) hyperorality, or examining objects by placing them in the mouth, (3) psychic blindness of seeing and not recognizing objects and the possible harm they may entail, (4) sexual hyperactivity, and (5) emotional changes characterized by loss of aggressiveness. These changes have been named the Klüver-Bucy syndrome . Myriad connections link the amygdala to the olfactory pathways, the frontal lobe and cingulate gyrus, the thalamus, the hypothalamus, the septum, and the midbrain structures of the substantia nigra, locus coeruleus, periaqueductal gray matter, and the reticular formation. The amygdala receives feedback from many of these structures it projects to by reciprocal pathways.
At the heart of the limbic network is the hypothalamus. The hypothalamus, in close reciprocal interaction with most centers of the cerebral cortex and the amygdala, hippocampus, pituitary gland, brain stem, and spinal cord, is a primary regulator of autonomic and endocrine functions and controls and balances homeostatic mechanisms. Autonomical and somatomotor responses controlled by the hypothalamus are closely aligned with the expression of emotions.
In the temporal lobe, anteromedially is the amygdaloid complex of nuclei, with the hippocampal formation situated posterior to it. Located medial to the amygdala is the basal forebrain nuclei, which receive afferent neurons from the reticular formation, the hypothalamus, and the limbic cortex. From this basal forebrain, efferents project to all areas of the cerebral cortex, the hippocampus, and the amygdaloid body, providing an important connection between the neocortex and the limbic network. These nuclei represent the center of the cholinergic system, which supplies acetylcholine to limbic and cortical structures involved in memory formation.
Interlinking the components of the system
The limbic network has many reciprocating interlinking circuits among its component structures, which provide for much functional interaction as well as allow for continuing adjustments with continuous feedback (see Fig. 4.2 ). , Neuroimaging has helped reduce uncertainty concerning the anatomical pathways, and neurochemistry has widened the possibilities of variations across synaptic connections. The largest of the interlinking pathways is the fornix, providing efferent and afferent connections to the hypothalamus and connecting the limbic network structures. The stria terminalis, another of the linking circuitry components, originates in the amygdaloid complex and follows a course close to the fornix to end in the hypothalamus and septal regions. The amygdala is also directly connected to the septal region by a short pathway, the diagonal band of Broca. These pathways serve as foundation for emotion memory and bonding. A third pathway, the uncinate fasciculus, connects the amygdala and the orbitofrontal cortex serving as a foundation for decision making. , ,
The medial forebrain bundle and other parallel circuits (see Fig. 4.5 ) are vital connections of the limbic network. These pathways course through the lateral hypothalamus to terminate in the cingulate gyrus in its ascending limb and in the reticular formation of the midbrain in its descending part; these pathways have strong interconnections and control over the periaqueductal gray area, and thus influence upon descending modulation of pain signals.
Commissural fibers from the vestibular nuclei run through the lateral medullary reticular formation (parvicellular reticular formation [PCRF]) and connect the vestibular nucleus to the reticular formation. The PCRF and the solitary nucleus receive both vestibular and nonvestibular input from the cortex, cerebellum, and the limbic network and are considered functionally as the “vomiting center.” It also receives heavy input from the chemoreceptor region in the floor of the fourth ventricle (area postrema), resulting in vomiting in response to noxious chemicals. The PCRF also projects fibers to the parabrachial nuclei that contain the respiratory centers and to the hypoglossal nucleus to activate and coordinate the protective gag reflex. Visceral autonomic input from multiple sources, including the vestibular nuclei, converges on the parabrachial nucleus. The locus ceruleus and autonomical brain stem nuclei also receive vestibular nuclear input. Thus cardiovascular activity and respiration (brain stem–mediated autonomic activity), as well as vomiting, are highly influenced by the status of the vestibular system and the limbic system. This helps us to understand how cold to the neck or forehead, pressure to the wrist, or taste or olfactory input of ginger depresses autonomic reactions and nausea in response to chronic vestibular or interneuronal connection problems. There are three different types of drugs that neuroanatomically suppress or modulate vestibular input and thus have a dramatic effect on dizziness and nausea. Additionally, functional magnetic resonance imaging (fMRI) confirms that there is increased activity within the inferior frontal cortex when nausea is induced by either vestibular stimulation or ingestion of an emetic. This research supports that there is a strong interconnection among vestibular input, limbic nuclei, and autonomic responses.
There are also connections between the parabrachial nucleus and higher brain centers, including the amygdala, which is known to be critical in the development of conditioned avoidance, such as found in agoraphobia, as an example. Thus vestibular input results in a sensory stimulus that may induce a state of general autonomic discomfort as a trigger of avoidance that precedes the onset of a panic attack. , , , Vestibular firing rates are further modulated and regulated by the dorsal raphe nucleus of the midbrain and rostral pons. The dorsal raphe nucleus is the largest producer of serotonin in the brain, which further explains the significant linkage between vestibular dysfunction and anxiety, and sleep deprivation and anxiety.
Additionally, animal research has shown that bilateral vestibular lesions result in changes in the morphology and function of the hippocampus, specifically associated with hippocampal atrophy. The hippocampus contributes to spatial and gravitational orientation, cognition, learning, and memory (spatial and nonspatial), which are symptoms and functional limitations often identified by individuals with vestibular dysfunction.
Central pattern generators found in the caudal brain stem and the spinal cord are understood to generate fixed rhythmic motor output patterns such as biting and swallowing. These motor tracts receive direct and indirect afferent information from the periphery and are part of the interneuronal projection system to motor neurons, contributing to the activity within the motor neuron pool. Their output is linked to the proximal (axial) and distal motor control system through the ventromedial and lateral descending motor pathways, modulated by a variety of structures, and regulated by the prefrontal area. , Their output is also strongly linked to the limbic network, resulting in an emotional context linked to the motor output. , The functional motor implication of these tracts is determined by whether the fibers project as part of the medial or lateral descending system. The medial components of this system originate within the medial portion of the hypothalamus, and the lateral portion originates in the limbic network (lateral hypothalamus, amygdala, and bed nucleus of the stria terminalis). The medial system, through the locus coeruleus, periaqueductal gray matter, and raphe spinal pathways, contributes to the general level of activity of both somatosensory and motor neurons. As such, the limbic network can have an effect on both somatosensory input and motor output. A change in activity in this system can alter the level of excitation at the first order synapse, thus altering the processing or sensitivity to that afferent information as it enters the nervous system. Similarly, it can alter the level of motor activity and thus motor output or expression, which may account for the increase in extensor muscle tone and extension seen with anger and the flexion and decrease muscle tone seen with depression. The lateral system seems to be involved in more specific motor output related to emotional behavior and may explain some of the loss of fine motor skill when one is placed in an emotional situation such as competition. The clinical relevance of this information highlights the need during patient examination to differentiate whether observed postural/muscle tone abnormalities are deficits within the motor system itself, or are a result of limbic system influence on that motor output. The clinician would need to observe and assess the emotional state, as well as how it changes with patient mood or activity, or with modification of the therapeutic environment. If the abnormal state consistently alters with mood shifts of control of emotional variables, then limbic involvement causing motor control disturbances could be identified. Human social behavior requires motor expression, yet that behavior is driven through the limbic circuitry.
These links enable the limbic network itself and the non–limbic-associated structures to act as one neural task system. No portion of the brain, whether limbic or nonlimbic, has only one function. Each area acts as an input-output station. At no time is it totally the center of a particular effect, and each site depends on the cooperation and interaction with other regions. The concept of neuroplasticity within the motor system and motor learning is well understood by physical therapists, but less understood is the integration of this emotional system interaction with sensory and motor components of motor performance and learning. Research is identifying that these neurocircuitries are present and interactive, and therefore should be considered.
Neurochemistry
Discussion of the limbic network’s intricate regulation of many neurochemical substances is not within the scope of this chapter. Yet therapists need to appreciate how potent this system can be with respect to the influence of neurochemical imbalance or loss on motor control, memory, or motor learning. The amount of research reflecting new understanding of the role of neurochemistry in brain function highlights the importance of frequent review of the literatures.
More than 200 neurotransmitters have been identified within the nervous system. The hypothalamus, the physiological center of the limbic network (see Figs. 4.1 and 4.5 ), is involved in neurochemical production and is essential for the passage of information along specific neurochemical pathways within the network. Squire and colleagues consider it the major motor output pathway of the limbic network, which also communicates with every part of this system.
Neuropeptides
The importance of neuropeptides is being recognized relative to the limbic network’s role in the regulation of affective and motivated behaviors. , , , Certain nuclei of the hypothalamus produce and release these neuroactive peptides, which have long-acting effectiveness as neuromodulators, controlling the levels of neuronal excitation and effective functioning at the synapses. Through their long-lasting effects, they regulate motivational levels, mood states, and learning. These peptide-producing neurons extend from the hypothalamic nuclei to the ANS and to the nuclei of the limbic network, where they modulate neuroendocrine and autonomic activities. Lesions in the medial hypothalamus affect hormone production and thus alter regulation of many hormonal control systems. For example, head injury may lead to medial hypothalamic lesions general hyperactivity, signs of hostility after minimal provocation, as well as huge weight gain resulting from the increase of insulin in the blood (increasing feeding and converting nutrients into fat) and loss of satiety.
The nuclei of the amygdaloid complex represent the center of the cholinergic system, which supplies acetylcholine to limbic and cortical structures involved in memory formation. Depletion of acetylcholine in patients with Alzheimer disease relates to memory loss. , ,
Monoamines
The monoamines play a critical role in many aspects of function and dysfunction within the limbic network. As a quick overview, the noradrenergic system (noradrenaline and adrenaline) plays a significant general role in the body’s response to stressors. In the brain, the noradrenergic system is located primarily in the locus coeruleus and plays a significant role in modulating behavioral and cognitive function within the cortex. The adrenal corticosteroids also contribute to modulation of long-term potentiation within the hippocampus. Novelty or reward-seeking and motivational behaviors of the limbic network seem to be dopamine dependent, whereas coordination of circadian body rhythm and sleep (critical for motor performance and learning) is influenced by levels of all of the monoamine neurotransmitters.
A functional deficiency in monoamines, especially serotonin , is hypothesized to be a primary cause of depression. , The serotonin systems originate in the rostral and caudal raphe nuclei in the midbrain. Ascending serotonergic tracts start in the midbrain and ascend to the limbic forebrain and hypothalamus; they are concerned with mood and behavior regulation. Damage to the ascending pathways contributes to depression as a mood disorder. In addition, newer evidence has highlighted the important role serotonin plays in motor control.
Descending pathways from the raphe nuclei to the substantia gelatinosa are involved in pain mechanisms. Through a complex sequence of biochemical steps, a reduction in serotonin in the raphe nuclei contributes to the increased sensitization of the presynaptic terminals of the cutaneous sensory neurons. Increased central sensitization of these terminals can lead to a hyperactive withdrawal reflex or hypersensitivity to cutaneous sensory input. This would account for the behavior patterns seen in patients with head trauma, when the therapist sees a flexed posture with a withdrawn or depressed affect associated with an extreme sensitivity to touch or even cutaneous input from clothing, air, etc.
Lesions in the lateral hypothalamus lead to damage of dopamine -carrying fibers that begin in the substantia nigra and filter through the hypothalamus to the striatum of the basal ganglia. Lesions, either along this tract or within the lateral hypothalamus, lead to aphagia and hypoarousal, as well as marked passivity with decreased functioning. Decreased sensory awareness contributing to sensory neglect is also present in lateral hypothalamic lesions. Disruptions of the indirect (hypothalamic) and direct (independent of hypothalamus) pathways from the mammillary bodies to the anterior thalamus contribute highly to loss of spatial orientation and memory. Bilateral infarcts within the mammillothalamic tract result in acute Korsakoff syndrome.
Dopamine is critical for motor cortex plasticity and motor skill acquisition and learning. Dopamine released from the VTA in the midbrain projects to the primary motor cortex (M1) and enables motor skill acquisition. Moreover, the dopaminergic VTA neurons projecting to M1 are activated when rewards are obtained during motor skill acquisition, but not during task execution in later associative or autonomous stages of motor learning, or when rewards are not associated with performing a skilled movement. Dopamine released from the VTA to the NAc and the frontal cortex plays an important role in reward processing for motor learning. Furthermore, in animal study, destruction of the VTA dopaminergic neurons prevented improvements in forelimb reaching, but learning recovered on administration of levodopa directly into the M1 of these VTA-lesioned animals. Of note is that lesioning the VTA did not affect performance of an already learned skill, meaning movement execution remained intact.
It is hypothesized that the underlying pathophysiological mechanism of one form of schizophrenia involves an excessive transmission of dopamine within the mesolimbic tract system. The dopaminergic cell bodies are located in the VTA and the substantia nigra. Some of these neurons project to the limbic network, specifically to the NAc, the stria terminalis nuclei, parts of the amygdala, and the frontal entorhinal and ACC, serving to modulate the flow of neural activity through the limbic network. The NAc may serve a critical role, acting as a filtering system with respect to affect and certain types of memory through its influence over the hippocampus, frontal lobe, and hypothalamus.
The specific roles of the noradrenergic pathway are numerous and affect almost all parts of the CNS. The center for the noradrenergic pathways is located within the caudal midbrain and upper pons. Its nucleus is referred to as the locus coeruleus. This nucleus sends at least five tracts rostrally to the diencephalon and telencephalon. Of specific interest for this discussion are the projections to the hippocampus and amygdala, which have an excitatory effect on the regions where they terminate. Thus the activation of this system will heighten the excitation of the two nuclei within the limbic network that are involved in declarative learning and memory. This excitation or hyperactivation may cause “overload” or impair focus of attention and learning. Decreased activity may prevent learning and memory. Attention to task may depend on continuing noradrenergic stimulation. These tracts, travelling rostrally from the midbrain, play a key role in alertness. The correlation of alertness and attention to performance and learning of motor tasks can be demonstrated.
In conclusion, the neurochemistry of the limbic network is intricately linked to the neurochemistry of the brain and the body organs regulated by the hypothalamus. All systems within the limbic circuitry seem to be interdependent, with the summation of all the neurochemistry being the determinants of the specific processing of information. Similarly, the interdependence of the limbic network with almost all other areas of the brain and the activities of those areas at any time reflect the complexity of this system.
Neurobiology of learning and behavior
Strub and Black view behavior as occurring on distinct interrelated levels that represent behavioral hierarchies. Starting at level 1, a state of alertness to the internal and external environment must be maintained for motor or mental activity to occur. The brain stem reticular activating system brings about this state of general arousal by relaying in an ascending pathway to the thalamus, the limbic network, and the cerebral cortex. To proceed from a state of general arousal to one of “selective attention” requires the communication of information to and from the cortex, the thalamus, and the limbic network and its modulation over the brain stem and spinal pattern generators. ,
Level 2 of this hierarchy lies in the domain of the hypothalamus and its closely associated limbic structures. This level deals with subconscious drives and innate instincts. The survival-oriented drives of hunger, thirst, temperature regulation, and survival of the species (reproduction) and the steps necessary for drive reduction are processed here, as well as learning and memory. Most of these activities relate to limbic functioning. If an individual or patient is in a perceived survival mode, little long-term learning regarding either cognition or motor programming will occur. Thus making the patient feel safe is initially a critical role for the therapist. This approach may require placing the therapist’s hands on the patient initially to take away any possibility of falling. The therapist would first deal with the emotional aspect of the patient’s environment and then shift to the motor learning and control component, in which the patient is empowered to practice and self-correct within the program she or he can control.
On level 3 only cerebral cortical areas are activated. This level deals with abstract conceptualization of verbal or quantitative entities. It is at this level that the somatosensory and frontal motor cortices work together to perceptually and procedurally develop motor programs. The prefrontal areas of the frontal lobe can influence the development of these motor programs, thus again illustrating the limbic influence over the motor system.
Level 4 behavior is concerned with the expression of social aspects of behavior, personality, and lifestyle. Again, the limbic network and its relationship to the frontal lobe are vital. The shift to the World Health Organization International Classification of Functioning, Disability and Health (WHO-ICF) model, which reflects patient-centered therapy, has actualized the critical importance of this level of human behavior.
The interaction of all four levels leads to the integrative and adaptable behavior seen in the human. Our ability to become alert and protectively react is balanced by our previous learning, whether it is cognitive-perceptive, social, or affective. Adaptability to rapid changes in the physical environment, in lifestyles, and in personal relationships results from the interrelationships or complex neurocircuitry of the human brain. When insult occurs at any one level within these behavioral hierarchies, all levels may be affected.
A fifth level of limbic function may one day be recognized as the link between the hard science of today and unexplained medical mysteries of healing. Meanwhile, how might the function of the limbic system explain certain mysteries, such as why some people heal from terminal illnesses spontaneously, others heal in ways not accepted by traditional medicine, , and others just die without any known disease or pathological condition? , , , It is proposed that a characteristic of the fifth level of limbic function is the patient’s strong, emotional belief that he or she will heal or will not heal. How conscious intent drives hypothalamic autoimmune function is being unraveled scientifically, and clinicians often observe these changes in their patients. Through observation it becomes apparent that patients who believe they will get better, often do, and those who believe they will not, generally do not. Whether belief comes from a religious, spiritual, or hard science paradigm, that belief drives behavior, and that drive has a large limbic component. Intellectual curiosity and human compassion motivates practitioners to explore these mysteries that otherwise might remain unexplained, overlooked, disbelieved, or forgotten.
As Western medicine continues to explore the intricate neurochemistry of the limbic network, alternative medicine is establishing effectiveness and efficacy for various interventions, approaches, and philosophies (see Chapter 39 ). The intersection of evidence and treatment efficacy is this: there is an interlocking dependence among somatosensory mapping of the functional skills (cognitive), attention (limbic) necessary for any type of learning, and the sequential, multiple, and simultaneous programming of functional movement (motor). The limbic amygdala and hippocampal structures and their intricate circuitries play a key role in the declarative aspect of memory and learning. The dorsal prefrontal cortex and the ACC were activated more when the subjects learned , a new sequence than they were when subjects simply paid attention to a pre-learned sequence. , Once this syntactical, intellectual memory is learned and taken out of short-term memory by passing through limbic nuclei, the information is stored in cortical areas and can be retrieved at a later time without limbic involvement.
Limbic influence on memory and motor learning
Recovery of function after injury may involve mechanisms that allow reorganizing of the structure and function of cortical, subcortical, and spinal circuits. In very young infants, areas within opposite hemispheres may “take over” function, whereas in more mature brains reorganization of existing parallel and silent pathways within and between hemispheres, as well as synaptogenesis are accepted recovery mechanisms within the expanding knowledge of neuroplasticity. For complex behavior, such as in motor functioning requiring many steps, the limbic network, cortex, hypothalamus, basal ganglia, and brain stem work as an integrated unit. As such, damage to one area may cause the whole system to initially malfunction. In addition, a lesion in one area may cause secondary dysfunction of a different area that was not damaged by the primary lesion. Without appropriate task (activity), internal and external environmental challenge designed to promote neuroplasticity, the initial malfunction can become permanent. The optimal rehabilitation timing for neuroplastic change has not yet been firmly established; however, it is accepted that there are key plastic “time windows.” The use of drug therapies to alter cellular activity and plasticity within these key windows after CNS damage has become a huge area of pharmaceutical research. This line of research is focused on the effect of the sensorimotor representation of movement within the cortex, which is a net effect of the stimulation of multiple pathways. The activation of specific direct, indirect, or modulating pathways engaged during the process of function-induced neuroplasticity, such as those of the limbic network, is less understood.
“Ultimately, to be sure, memory is a series of molecular events. What we chart is the territory within which those events take place.” The brain stores sensory and motor experiences as memory. In processing incoming information, most sensory pathways from receptors to cortical areas send vital information to the components of the limbic network. For example, extensions can be found from the visual pathways into the inferior temporal lobe (limbic network). , , Visual information is “processed sequentially” at each synapse along its entire pathway, in response to size, shape, color, and texture of objects. In the inferior temporal cortex, the total image of the item viewed is projected. In this way the sensory inputs are converted to become “perceptual experiences.” This also applies to other sensory stimuli, such as tactile, proprioceptive, and vestibular. The process of translating the integrated perceptions into memory occurs bilaterally in the limbic network structures of the amygdala and the hippocampus. , , ,
Before the limbic network’s impact on learning and memory can be investigated, a clear understanding of what is meant by these functions is needed. Current theories support a “dual memory system” consisting of different pathways in the nervous system. Terms such as verbal and nonverbal, habit versus recognition, intrinsic and extrinsic, and procedural and declarative have been given to these two memory systems. These systems do not operate autonomously, and many therapeutic activities seem to combine these memory systems to achieve functional behavior. As such, in reality, the complexity of memory is not a two-category system. Verbal and nonverbal memory both interact with declarative function. Even within spatial memory, additional areas of integration and parallel circuitry have been identified. ,
For this discussion, two specific categories of learning—procedural and declarative—will be used, although in today’s neuroscience environment, the terms implicit and explicit memory are used as frequently. Both categories of learning have been correlated to limbic function. Declarative (explicit) memory entails the capability to recall and verbally report experiences. This recall requires deliberate conscious effect, whereas the procedural counterpart is the recall of rules, skills, and procedures (implicit), which can be recalled unconsciously.
Procedural learning is vital to the development of motor control. A child first receives sensory input from the various modalities through the thalamus, terminating at the appropriate sensory cortex. That information is processed, a functional somatosensory map is formulated, , and the information is programmed and relayed to the motor cortex. From there, it is sent to both the basal ganglia and the cerebellum to establish plans for postural adaptations, refinement of motor programs, and coordination of direction, extent, timing, force, and tone necessary throughout the entire sequence of the motor act. Procedural learning and memory do not require limbic network involvement as long as an emotional value is not placed on the task. This memory deals with skills, habits, and stereotyped behaviors. Summarizing, the frontal lobe, basal ganglia, and cerebellum are critical nuclei for changing and modulating existing programs. Storage and subsequent retrieval of memory of these semiautomatic motor plans are thought to occur throughout the motor control system. This motor system is involved in developing procedural plans used in moving us from place to place or holding us in a position when we need to stop. The complexity of this process has had an impact on the study of motor control and variables that might affect that control.
Unlike procedural learning and memory, declarative (explicit) learning and memory require the wiring of the limbic network and are closely associated with limbic function. Declarative thought deals with factual, material, semantic, and categorical aspects of higher cognitive and affective processing. A strong emotional and judgmental component is linked with declarative thought. Thus as soon as a motor behavior has value placed on the act, it becomes declarative as well as procedural, and the limbic network may become a key element in the success or failure of that movement. , Most functional tasks or activities practiced in a clinical setting have value attached to them. That value can be clearly seen by observing the emotional intent placed on the activity by the patient.
Two reciprocal pathways, or circuits, within the limbic network are intimately involved in the process of declarative learning and memory (1) the amygdaloid, dorsomedial thalamic nucleus, and cortical pathways and (2) the hippocampal, fornix, anterior thalamic nucleus, and cortical pathways. Both pathways contribute in relation to the significance of external or internal environmental to learning of a concept or task. The amygdala may be more concerned with sensory and motor signals relating to the internal environment, whereas the hippocampus is concerned more with those of the external environment. The hippocampus is rich in stem cells and may be a primary nuclear mass that directs the bodily systems to heal after injury. This is especially true when the external environment is enriched and nurtures the emotional environment for that healing. ,
For initial declarative learning and memory, function of the hippocampus and the amygdala of the limbic network is required. These two structures play an important role within the “cortico-limbo-thalamo-cortical” circuit, which plays a significant role in memory storage. For memory formation to occur in early motor learning (acquisition), there must be a storing of the “neural representation” of the stimuli in the association and the processing areas of the cortex. This “cortico-limbo-thalamo-cortical” circuit serves as the “imprinting mechanism” by which pathway activation by stimulus is reinforced. Limbic involvement in the declarative memory and learning processes creates a chemical bond that allows cortical storage of “stimulus representation” necessary for subsequent recognition and recall of the information. , , , , Therefore on subsequent stimulation, a stimulus recognition or recall would be elicited. In the associative phases of recall and transfer of learning, stored representations of any interconnected imprints might be evoked simultaneously.
The amygdaloid circuits seem to deal with strongly emotional and judgmental thoughts, whereas the hippocampal circuits are less emotional and more factual. The amygdala may be more involved in emotional arousal and attention, as well as motor regulation, whereas the hippocampus may deal with less emotionally charged learning. It is postulated that the amygdala is the area of the brain that adds a “positive association,” associating a stimulus and reward and placing an emotional value memories or learning. In this way, stimulus and reward are associated by the amygdala, and an emotional value is placed on them. , These limbic circuits seem crucial in the initial processing of material that leads to learning and memory. Once the thought has been laid down within the cortical structures, retrieval of that specific intermediate and long-term memory does not seem to require the limbic network, although new associations will need to be run through the system. , , ,
The hippocampus and amygdala are also linked both structurally and functionally to each other and to specific thalamic nuclei in the medial diencephalon. The medial diencephalon is an important relay station along the pathway that leads from the specific sensory cortical region to the limbic structures in the temporal lobe to the medial diencephalic structures and ends in the ventromedial part of the prefrontal cortex ( Fig. 4.6 ). , , A vital processing area for all sensory modalities is located in the region of the anterior temporal lobe. Patients with temporal epileptic seizures and whose temporal lobes have been surgically removed develop global anterograde amnesia—that is, amnesia develops for all senses, and no new memories can be formed. Experimental removal of only the hippocampus does not bring about these changes, although processing is slowed down. When both the hippocampus and the amygdala are removed bilaterally, the amnesia is both retrograde and anterograde. A third component in the memory pathway involves pathways between the amygdala, hippocampus, and the thalamic nuclei in the medial diencephalon. When the medial diencephalic region is damaged by neurological trauma such as strokes, neoplasms, infections, or chronic alcoholism, global amnesias result. Given that the limbic network and the diencephalon cooperate in the memory circuits, the destruction of these pathways causes the same amnesic effect.

As shown in Fig. 4.6 , memories may be stored in the sensory cortex area, where the original sensory input was interpreted into “sensory impressions.” Today, concepts regarding memory storage suggest that declarative memory is stored in categories similar to a filing system. Those categories or files seem to be stored in several cortical areas bilaterally depending on the context. , This system allows for easy retrieval from multiple areas. Memory has stages and is continually changing. It was once thought that the hippocampus only dealt with long-term memory, but it is now accepted that it also supports multi-item working memory. To go from short-term to long-term memory, the brain must physically change its chemical structure (a plastic phenomenon). Memory first begins with a representation of information that has been transformed through processing of perceptual systems. The transferring of this new memory into a long-lasting chemical bond requires the neuronal network of the limbic complex. Owing to the multiple tracts or parallel circuits in and out of the limbic network and throughout neocortical systems, patients, even with extensive lesions, can often learn and store new information. , This may also explain why damage to the limbic network structures does not destroy existing memory nor make it unavailable because it is actually stored in many places throughout the neocortex. The circular memory circuit illustrated in Fig. 4.6 shows only one system. The reader must remember that many parallel circuits function simultaneously. The circular memory circuit shown reverts to the original sensory area after activation of the limbic structures to cause the necessary neuronal changes that would inscribe the event into retrievable stored memory. This information can be recognized and retrieved by activation of storage sites anywhere along the pathway. , Patients with brain lesions localized in the limbic network components of the amygdala and hippocampus have the ability to acquire and function with “rule-based” games and skills but have lost the capacity to recall how, when, or where they gained this knowledge or to give a description of the games and skills learned. Relating this to clinical performance, patients may develop the skill in a functional activity but not the problem-solving strategies necessary to associate danger or other potentially harmful aspects of a situation that may develop once out of the purely clinical setting. , Similarly, if a patient needs to learn a procedural task such as walking, transfers, eating, and so on, it may be extremely important to direct the attention off the task while the task is being practiced procedurally.
The last station or system to be added to the circuit is the “basal forebrain cholinergic system,” which delivers the neurochemical acetylcholine to the cortical centers and to the limbic network, with which it is linked. Visual recognition memory can be augmented or impaired by administration of drugs that enhance or block the action of acetylcholine. The loss of this neurotransmitter is linked to memory malfunctioning in Alzheimer disease and plays a key role in dementia problems in Parkinsonism. Currently, many chemicals are being studied for their influence on brain structures and specially limbic structures. , ,
The hippocampus and the amygdala are involved in recognition memory. Hippocampal (indirect) and nonhippocampal (direct and independent) pathways influence the anterior thalamus and thus play an important role in memory, particularly spatial memory and location of objects in space. The the amygdala is necessary for the association of memories derived through the various senses with a specific recognition recall. For example, a whiff of ether might bring to mind a painful surgical experience or the sight of some food may cause a recall of its pleasant smell. Removal of the amygdala brings out the behavior shown in Klüver-Bucy syndrome. For patients with this neurological problem, familiar objects do not bring forth the correct associations of memories experienced by sight, smell, taste, and touch and relate them to objects presented. Association of previously presented stimuli and their responses appear to be lost. Animals without amygdaloid input had different response patterns that ignored previous fears and aversions. Thus the amygdala adds the “emotional weight” to sensory experience. Loss of the amygdala takes away many positive associations and potential rewards, thereby altering the shaping of perceptions that lead to memory storage.
When stimuli are endowed with emotional value or significance, attention is drawn to those possessing emotional significance, selecting these for attention and learning. This would give the amygdala a “gatekeeping” function of selective filtering. The amygdala may enable emotions to influence what is perceived and learned by reciprocal connection with the cortex. Emotionally charged events will leave a more significant impression and subsequent recall. The amygdala alters perception of afferent sensory input and, hence, affects subsequent actions. , ,
In the human, memory functioning has been associated with the phenomenon of long-term potentiation observed in hippocampal pathways. This potentiation of synaptic transmission, lasting for hours, days, and weeks, occurs after brief trains of high-frequency stimulation of hippocampal excitatory pathways. Whether this long-term potentiation occurs presynaptically, postsynaptically, or both has not yet been established. , , The adrenal corticosteroids contribute to modulation of long-term potentiation within the hippocampus. Recent literature has linked a neurotropic factor usually considered for long-term potentiation within the hippocampus as a factor in amygdala-dependent learning, thus reiterating the interaction between these two nuclei and their role in memory and learning.
Learning and memory evoke alterations in behavior that reflect neuroanatomical and neurophysiological changes. , These alterations include the phenomenon of long-term potentiation as an example of such changes. The hippocampus demonstrates the importance of input of long-term potentiation in associative learning. In this type of learning, two or more stimuli are combined through the “associative” interaction of afferent inputs. As such, tetanizing of more than one pathway needs to occur simultaneously. If only one pathway is tetanized, the effect is decreased synaptic transmission. Thus long-term potentiation serves as one model for understanding the neural mechanism for associative learning. Hormones interact with this neural mechanism, which, if combined with stress, can change the specific circuitry active during the experience. As an example, the amygdala is not only involved in learning related to emotional experiences but is also responsible for changing motor expression or conditioned response generated as part of an autonomic expression, such as with fear. , Limbic responses to input stimuli need to be differentiated from limbic memory and initiation of a response without the stimuli. Although this is an area of practice not yet fully understood, clinical implications to patient examination and evaluation will be generally discussed later in this chapter.
Limbic influence on emotions and behaviors: The F 2 ARV and general adaptation syndrome continua
Noback and co-workers state that the limbic network is involved with many of the expressions that make us human, namely, emotions, behaviors, and feeling states. That humanness is highly individual even in patients with normal functioning limbic networks, and even greater variance with involvement of the primary or modulating limbic network structures or pathways.
As a brief clinical example, a patient with severe brain injury with decreased responsiveness (Ranchos Los Amigos Level of Consciousness II–III) may show increased alertness and an increase in base muscle tone or motor function through effective use of sensory stimuli. Alternatively, although the desired response may be improved alertness or a motor response, a patient may become highly over-sensitive to a strong odor or noxious tactile stimuli, and the response may become autonomic or behavioral instead, reflected in an increased heart rate or blood pressure, or even as fear or anger. Overstimulation may move the patient into a “protective state of survival,” whereas a pleasant/personally desirable smell, touch, or sound (e.g., melody) will more likely lead to “safety.” The former can lead to strong emotions or responses such as anger; the latter often leads to bonding, engagement (attention), and motivation to learn.
Both motivation (“feeling the need to act”) and concentration (“ability to focus on the task”) are interlinked with the limbic network and are both critical to participation in the motor activities. The amygdaloid complex with its multitude of afferent and efferent interlinkages is specially adapted for recognizing the significance of a stimulus, and it assigns the emotional aspect of feeling the need to act. These neuroanatomical loops have tremendous connections with the reticular system. Hence, some authors call it the reticulolimbic network. , The interaction of the limbic network and the motor generators of the brain stem and ultimate direct and indirect modulation over the spinal system lead to need-directed and therefore goal-directed motor activity. It also filters out insignificant from significant information through selective processing, storing the significant for memory, learning, and recall. These interconnected neuroloop circuitries reinforce the concept that areas have both specialization and generalization and thus work closely together with other areas of the brain. ,
Some of the earliest understanding of the limbic network was limited to that of a primary protective role in “fight or flight.” With normally functioning limbic networks, patients can appropriately recognize the significance of a stimulus, effectively filter out insignificant from significant stimuli or information, and appropriately assign a need to act. However, in the impaired patient, inaccurate stimulus interpretation and therefore action/reaction occur. The therapist needs to be aware that a patient may overrespond to stress, frustration, or fear of failure in both cognitive and motor activities. A small or unfiltered stimulus may generate one of two powerful and predictable limbic motor response programs: the fear and frustration, anger, rage, and violence continuum (F 2 ARV) and the general adaptation syndrome (GAS). The F 2 ARV and GAS continua are often interrelated in individuals who have direct or indirect limbic network involvement.
Fear and frustration, anger, rage, and violence or withdrawal continuum.
One sequence of behaviors used to describe the emotional circuitry of the limbic network through the amygdala is the F 2 ARV continuum ( Fig. 4.7 ). , , This continuum begins with fear or frustration. This fear can lead to avoidance behavior. If the event inducing the fear or frustration continues to heighten, avoidance behaviors can continue to develop. In a simple example, we recall or have seen these behaviors in our teens and as young adults, when the challenges faced in high school can lead to avoidance of activities. Alternately, extreme fear and frustration can also lead to anger. Anger is a neurochemical response that is perceived and defined cognitively (at the cortical level) as anger. If the neurochemical response continues to build or is prolonged, the anger displayed by the person may advance to rage (internal chaos) and finally into violence (strong motor response). A common societal example is in the case of domestic discord and violence. Women who attain the level of rage may become withdrawn and thus become victimized by a partner who is also in rage or inflicting physical or emotional violence. Another current example is posttraumatic stress disorder (PTSD), in which the prolonged stress of deployment and unique challenges of warfare lead to limited adaptive reserves in warriors and returning veterans.

How quickly and completely any individual will progress from fear to violence is dependent on several factors. First, the genetic neurochemical predisposition (initial wiring) will influence behavioral responses. Second, “soft-wired” or conditioned responses resulting from experiences and reinforced patterns will influence output. For example, it is commonly known that abusive parents were usually abused children , ; they learned that anger quickly leads to violence and that the behavior of violence was somehow acceptable. Last, the quality and intensity of the stimulus initiating the continuum will influence the level of response.
The neurochemistry within an individual’s CNS, whether inherently active or altered through drugs or injury, will have great influence on the plasticity of the existing wiring. , Repetitive or prolonged exposure to negative environmental stimuli may also lead to a chronically imbalanced neurochemical state that results in a lowered threshold or tolerance to a given stimulus. Chemistry or wiring can become imbalanced from damage, environmental stress, learning, or other potentially altering situations, changing an individual’s control over this continuum. , , , When neurochemical imbalance exists, these behaviors will persist, and balance may be restored only through natural neurochemical activity (e.g., sleep, exercise, diet, spirituality) or medication support (chemical replacement).
Therapists need to be acutely aware of this continuum in patients who have diffuse axonal shearing within the limbic complex. Diffuse axonal shearing is most commonly seen and reported in research on individuals with head trauma (see Chapter 23 ). , Resulting lesions within the limbic structures may cause an individual to progress down this continuum at a rapid speed. This point cannot be overemphasized. Patients with an accelerated F 2 ARV continuum, whether through prior learning or due to the injury, may physically strike out at a clinician or caregiver out of simple frustration during care. Knowing the social history of the patient and the causation of the injury often can help the therapist gain insight into how an individual patient might progress down this continuum. Not all head-injured patients had prior difficulty with the F 2 ARV continuum; however, it is important to note that many individuals received their head injuries in violent confrontational situations or in wartime conflict. Some individuals, primarily females, when confronted with stress, anger, and potential violence from another, will withdraw and become depressed. This behavior, similar to violence, will change the structure of the limbic network. A brief patient example of accelerated limbic-driven responses along the F 2 ARV continuum is described in Clinical Case 4.2 .
A 40-year-old man presented with moderate physical and severe cognitive functioning deficits resulting from a brain aneurysm, associated with violent episodes of rage. He would escalate very rapidly along the F 2 ARV continuum when presented with unpredictable environmental stimuli, such as passing another patient with his wheelchair in the hallway. Although his physical rehabilitation had progressed well and he had made functional gains in mobility, because his assaultive outbursts posed risks to other patients as well as to caregivers, this patient appeared to be heading for placement in a locked facility, a more restrictive environment than he would need considering his level of physical limitation. Although this unfortunate man had no short-term memory function or insight into his behaviors, assessment revealed that he was highly responsive to calming music and was able to access some intact long-term memories that could be used to elicit a relaxation response. A highly positive or neutral limbic state of deep relaxation was therefore facilitated using meaningful, calming music, and simple verbal cues were presented/timed to develop a conditioned response that any staff member could then call forth with the verbal cue alone. The entire rehabilitation team was briefed on the use of this intervention and reported success using this approach during nursing care and rehabilitation interventions when the patient would become resistant or angry in response to clinician instructions. The patient was able to be trained to self-regulate by giving himself the same verbal cue when confronted with challenging situations. This treatment supported the patient’s ability to regain emotional controls and allowed him the opportunity to be placed in a less-restrictive community environment. The patient never recalled a previous music therapy treatment session and asked each time to have the purpose of the treatment explained to him. But his body remembered the set of behavioral experiences, and he quickly complied with the relaxation procedure. The success of this approach demonstrates that even in the absence of short-term memory and other cognitive functions usually considered essential for new learning, the skillful engagement of positive limbic states and intact areas of patient functioning (strengths) can support development of new adaptive skills, which can be generalized to new environments.
General adaptation syndrome.
The autonomic responses to stress also follow a specific sequence of behavioral changes and are referred to as the general adaptation syndrome. The sequential stages of GAS are a direct result of limbic imbalance and can play a dramatic role in determining patient progress.
Stress can be caused by many internal or external factors, often unique to the individual. Examples include pain, acute or chronic illness or the ramification of illness, confusion, sensory overload, and a large variety of other potential sources. The initial reaction to a stressor is a neurochemical change or “alarm” that triggers a strong sympathetic nervous system reaction. Heart rate, blood pressure, respiration, metabolism, and muscle tonus will increase. It is at this stage that the grandmother lifts the car off the child as in our previous example. If the overstimulation or stress does not diminish, the body will protect itself from self-destruction and trigger a subsequent parasympathetic response. At this time, all clinical symptoms reverse and the patient exhibits a decrease in heart rate, blood pressure, and muscle tonus. The bronchi become constricted, and the patient may hyperventilate and become dizzy, confused, and less alert. As the blood flow returns to the periphery, the face may flush and the skin may become hot. The patient will have no energy to move, will withdraw, and again will exhibit decreased postural tone and increased flexion.
This stress or overstimulation syndrome is characterized by common symptoms as described earlier. , If the acute symptoms are not eliminated, they will become chronic and the behavior patterns much more resistant to change.
GAS is often seen in the elderly, with various precipitating health crises (and also in neonatal high-risk infants), victims of head trauma, and other patients with neurological conditions. The initial alarm can be precipitated by moderate to maximal internal instability with less intensive external stress, or by minimal internal instability with severe external sensory bombardment. For instance, in the elderly, stresses such as change of environment, loss of loved ones, failing health, and fears of financial problems can each cause the patient’s system to react as if overloaded. As another example, individuals with head trauma, vestibular dysfunction, inflammatory CNS problems, and brain tumors often possess hypersensitivity to external input such as visual environments, noise, touch, or light. In these individuals, typical clinical environments and therapeutic activities may create a sensory overload and trigger a GAS response.
Stress, no matter what the specific precipitating incident (confusion, fear, anxiety, grief, or pain), has the potential to trigger the first steps in the sequence of this syndrome. , The clinician’s sensitivity to the patient’s emotional system will be the therapeutic technique that best controls and reverses the acute condition.
Similarly, patients with dizziness and instability, particularly within visually stimulating environments, can develop feelings of panic, which can evolve into full attacks and agoraphobic responses. These individuals avoid participating in activities that put them within visually overstimulating environments in an effort to control the dizziness and prevent the associated autonomic reactions. Similar types of reactions have been documented, such as space-motion discomfort (SMD), postural phobic vertigo, visual vertigo, and dizziness of “psychogenic” origin. Often these individuals are referred first to psychology or psychiatry. However, there is an underlying physiological explanation for these symptoms. In a majority of individuals with SMD, there is a documented increase in vestibular sensitivity (increased vestibulo-ocular reflex [VOR] gain) and an impairment in velocity storage (shorter VOR time constant). In addition, the dorsal raphe nucleus (dorsal reticular nucleus of the midbrain and rostral pons) is the largest serotonin-containing nucleus in the brain and directly modulates the firing activity of the superior and medial vestibular nuclei. It is this interaction between serotonin and vestibular function that helps to explain the link between vestibular and anxiety disorders. It can also help explain how patients with sleep disorders or other serotonin-depleting disorders develop vestibular-like symptoms and anxiety. , A comprehensive clinical example of the influence of the limbic system in patients with vestibular dysfunction can be found in Clinical Case 4.3 .
Although there are physiological reasons underlying the vestibular system disorder in a majority of these cases, the symptoms triggered are part of a spectrum of limbic responses to aberrant vestibular, cerebellar, and brain stem interactions. Normal clinic activity or typically appropriate therapeutic activity may trigger an autonomic cascade versus the desired somatomotor response. The rehabilitation of the resultant visual and postural movement dysfunction is typically more complicated in the absence of limbic network management. The clinician’s strategic prescription (or “dosing”) of therapeutic activities with careful monitoring of the patient’s emotional system and physiological response will be one of the therapeutic techniques that best controls the aberrant responses and allows vestibular adaptation and compensation to occur. This must be done to manage limbic network activity for successful motor learning to occur.
Developing limbic network assessment tools (or repurposing existing tools) for their ability to screen or identify the presence of direct or indirect limbic involvement is of critical value. In addition, the ability to discriminate the type of limbic involvement (decreased responsiveness and withdrawal from increased responsiveness or overresponsiveness) is important to treatment planning. Treatment techniques will be discussed later in the chapter. However, the specific techniques appropriate for treating these syndromes are tools all therapists possess. These tools range from simple variations in approach (e.g., lighting, sound, and smell) to more formal therapeutic techniques, such as the Feldenkrais approach, or the Bonny Method of Guided Imagery and Music. How each clinician uses those tools is a critical link to success or failure in clinical interaction.
Limbic influence on motor performance and function
Kandel and colleagues state that functional behavior requires three major systems: the sensory, the motor, and the motivational or limbic systems. When a seemingly simple action, such as swinging a golf club, is analyzed, the sensory system is recruited for visual, tactile, and proprioceptive input to guide the motor systems for precise, coordinated muscle recruitment and postural control. The motivational (limbic) system does the following: (1) provides intentional drive for the movement initiation, (2) integrates the total motor input, and (3) modifies motor expression accordingly, influencing both the autonomic and the somatic somatomotor systems. It thereby plays a role in controlling the skeletal muscles through input to the frontal lobe and brain stem and the smooth muscles and glands through the hypothalamus, which lies at the “heart” of the limbic network ( Fig. 4.8 ).

The motivational or limbic component is further understood to be linked to the NAc, which has been described as the “limbic-motor interface.” The NAc has been found in animal studies to link motor responses with the value of expected rewards associated with a given motor plan. The expected reward is encoded with a behavioral response within a given neural signal. The spatial and motor components of a given behavioral response are similarly encoded together, including the timing and accuracy components of a movement. It is important to note that within this encoding process, the predictability of the behavior and reward are critical for activation of the NAc neurons. This supports the concept that predictability within the therapeutic tasks, environment, and alliance are critical to motivation and, as such, to motor output and learning.
Though MOVE has been used to delineate specific functions of the limbic network that drive movement, it is important to remember that these functions are highly interactive and individual. Our unique memory storage, our variable responses to different environmental contexts, and our control or lack thereof over our emotional sensitivity to environmental stimuli all play roles in the expression of our motor output, motor performance, and function. As such, an understanding of the influence of the limbic network on an individuals’ function helps further define our need to assess the state of this system—and for those findings to factor into our treatment plans.
To appreciate the sensory system’s influential interaction with the limbic network directly, the reader might also look at the literature on music and how it interacts with emotions. , Most people can give examples of instances where music has elicited immediate and compelling emotional responses of various types. Pleasant and unpleasant musical stimuli have been found to increase or decrease limbic network activity and influence both cognitive and motor responses, although the neurological mechanisms are not completely understood. Consider the clinical implications. Reflect on the typical treatment environment: frequent and variable noise, loudspeaker announcements, piped-in music, and a cacophony of therapists’ voices can quickly overload the CNS of a patient and result in a limbic response versus a desired motor or functional response. These responses can be highly emotional or limbic high , resulting in visceral behavior and affecting motor expression. Alternately, the response may be calming or relaxing to a limbic neutral state, allowing for full engagement (motivation, attention) in the movement or task at hand. Musical consonance or dissonance (stable versus unstable) of an auditory stimulus is subjectively experienced by the listener as pleasant or unpleasant. Listening to music uniquely affects limbic emotional states, and the influence may direct the hypothalamus in regulation of blood flow within the CNS. With music or sound being just one input system, the therapist must consider that sensory influence from smell, taste, touch, proprioception, and vestibular and organ system dysfunction can lead to potential limbic involvement in all aspects of CNS function and directly affect the emotional stability of the patient.
Clinical implications of the limbic state
Today the medical profession is rediscovering the importance of how the systems interact with and influence one another. , Similarly, it is very important for movement specialists to look at movement not only from a biomechanical, muscular, neurological, cardiopulmonary, or integumentary system perspective, but also to accept that interactions between systems highly influences movement. What is less understood, or perhaps considered, by physical therapists are the interactions between the environment and behaviors, learning and movement, including the therapeutic alliance between therapist and patient. Cognitive impairment and limbic network involvement can lead to tremendous errors in motor responses even when the motor system is intact.
Although far from yielding a complete understanding, this research and knowledge are increasing daily and force today’s therapist not only to recognize limbic behavior but also to develop an understanding of how involvement of the limbic network will positively and negatively affect the movement of each patient. Therapists can no longer think of motor control and motor learning as controlled exclusively by an anatomically unique motor system, nor can we understand movement using motor control or motor learning principles alone. Therapists must consider how the limbic network strongly influences motor sequencing and control, behaviors that influence function and participation (attention, motivation, and cooperation), as well as beliefs and attitudes. Any of these factors may affect outcomes through influence on engagement or empowerment over treatment planning and/or responsibility for, and compliance with, home programs. Without an understanding of limbic interactions and modulations over motor expression, patient performance and outcomes may show greater variability, even with consistent and accepted interventions. And, given the limitations in today’s health care delivery models, stresses, and the growing dependence on home programs, without a keen awareness of the limbic responses of both the patient and the provider, a therapist will have reduced influence over the best functional outcome for their patients.
The complexity of the limbic network and its associated influence over both the motor control system and cortical structures are enormous. A therapist dealing with a patient with motor control or learning problems needs to understand how the limbic network affects behavioral responses and functional performance. This understanding should lead to a greater awareness of the individual factors associated with limbic network involvement, as well as the clinical activities (interventions) and environmental factors that will result in positive neuroplastic change and motor learning. Without this knowledge of how to differentiate limbic from other system dysfunction within the physical therapist evaluation, objective measurements of motor performance or cognitive abilities may be inaccurate, inconsistent, or variable between therapists or sessions without apparent explanation. Similarly, with aberrant limbic activity (high or low), a patient’s ability to store and retrieve either declarative or procedural learning may be negatively affected, thus limiting the patient’s ability to benefit from traditional interventions or to achieve optimal quality of life.
Examination and evaluation
Throughout this chapter we have discussed negative limbic influences or states and positive limbic states. Negative limbic states include the overloaded or overactive state, termed limbic high, whereas underactive limbic network states can be termed limbic low (underactive). The optimal state of any human limbic network is one that is devoid of negative limbic influences, or a limbic neutral state. A limbic neutral state provides a strong foundation for procedural learning to occur. We discuss how various disorders are typically associated with negative limbic states in Table 4.1 .
Limbic State | Often Associated With | Observed Behaviors |
---|---|---|
Neutral | Health and wellness | Relaxed state |
Low | Depression and stroke | Decreased eye contact and crying Lack of motivation |
High | Anxiety and panic disorders Vestibular disorders Mild or traumatic head injury Blast injury, PTSD | Anxiety, anger, fear, increased respiratory rate, higher blood pressure, high muscle tension or tone, and hyperactivity |
Overload (F 2 ARV, GAS) | Traumatic head injury Blast injury, PTSD, frail individual (either young or old), and physiologically unstable individual | Violence, extreme withdrawal, loss of inhibition, and reversal of expected behavior |
Therapists are familiar with the need to prioritize primary body system impairments as they relate to a movement disorder. Moreover, they are familiar with the need to individualize treatment to the primary impairment(s) identified through the evaluation process. Logically, the next consideration for medical and rehabilitative professionals is how we determine or assess the limbic network state of our patients, using qualitative versus quantitative assessment. The master clinician can surmise this intuitively, based upon both observation and experience. But how do we develop this as a requisite skill in our students and colleagues, and can we agree that there is an opportunity to do so? Considering the evidence presented within this chapter, one might challenge that there is a need to do so. And if this is true, what existing assessment tools are there to assist us in doing so ?
Traditionally, observation was our main assessment tool in the identification of these impairments. Observation of patient presentation and behaviors would provide an astute clinician with insight into the system. It was not included as a standard part of the assessment, but rather a notation of extremes. There are no assessment tools validated to provide insight into the limbic network state. This certainly makes sense given the broad-reaching structural and functional influences of the system as outlined within this chapter. Similarly, beyond strategic management of the environmental stimuli for patients with head injury or clear agitation, there was no clear path from observation to screening to assessment to intervention.
As such, it is recommended that we agree to the importance of elevating the limbic network to a primary body system that has significant, well-established influence over movement. As the movement specialists, it is therefore recommended that we commit to the validation and/or development of screening and assessments of the limbic state. Screening of the system is designed to be binary, ruling individuals either in or out of the limbic impaired group. Just as in the identification of fall risk, high specificity and potential errors in ruling in might be considered. However, despite the absence of such tools, we can propose that observation of limbic-associated presentation and behaviors could trigger a therapist to be alert and to set the therapeutic environment in general. In Table 4.2 we outline the ways in which a therapist might use existing skills and knowledge to quickly screen patients for negative limbic network involvement, with examination of the system if negative influence ( limbic high or limbic low state) is suspected. It might be recommended that all at-risk patients by history, as well as those individuals who have primary or secondary involvement of the limbic structures or have neurochemical risk, undergo examination. The potential population of individuals is described in examples throughout the foundations of this chapter, common disorders summarized at the end of this chapter, and outlined again for the reader here, in the context of the evaluation, in Table 4.2 .
Limbic Low | Limbic High | |
---|---|---|
Observation (behaviors) | Vision —decreased eye contact; downward cast eyes Voice —low volume and rate and poor articulation Muscle —low muscle tone; decreased extensor muscle tone and often increased flexor tone. Posture —flexed posture; forward head; and may have flexed extremities, often have poor postural trunk coactivation. Activity level —slowed movements/decreased responsiveness or engagement and decreased attention, motivation, and fatigues quickly. | Vision —decreased eye contact; darting or scanning eye movement Voice —increased rate, variable volume, and poor articulation Muscle —high muscle tone; increased extensor muscle tone. Posture —extended posture; may have extended extremities. Sensory —lower thresholds to stimuli Activity level —hyperactive or hypervigilant; “nervous” behaviors (twitching, leg or finger tapping, fidgeting, etc), agitated or irritated engagement; attention deficit. |
Systems review | Skin —pallor Respiratory rate/quality —slowed, shallow or deep; heavy sighs and yawns | Skin —can be red, blotchy in color Sensory —tactile sensitivity or hypersensitivity; dysesthesias; and Allodynia Respiratory rate/quality—rapid and shallow |
History | Psychological —depression, bipolar depression; grief; substance abuse Neurological —left CVA; normal pressure hydrocephalus; acquired cerebral disorders (stroke, tumor, and ventricular disorders); progressive cerebral disorders (PD, MS, ALS, etc) Cardiopulmonary —primary cardiac disorders Chronic disorders/illness —chronic pain; prolonged hospitalization; multifactorial disequilibrium; and dysequilibrium of aging | Psychological —Anxiety? PTSD? Bipolar depression; fear/fear of falling. (Can come from a prior abusive environment) Neurological —right CVA; late phase dementia, Parkinson disease; head injury (traumatic of any severity; concussion; blast injury); and vestibular dysfunction Cardiopulmonary —primary cardiac disorders; COPD and primary respiratory disorders Musculoskeletal —MVA Social/vocational —contact sports and military service (with or without blast exposure); domestic violence Chronic disorders/illness —chronic pain |
Examination (examples, but not limited to) | Vital signs —decreased HR, RR, and gait velocity Tests and measures : Positive and Negative Affective Scale (PANAS) Depression Anxiety Stress Scales (DASS21) Geriatric Depression Scale (GDS) | Vital signs —increased HR, RR, gait velocity Tests and measures : Positive and Negative Affective Scale (PANAS) Depression Anxiety Stress Scales (DASS21) Agitated Behavior Scale Ranchos Level of Cognitive Function (RLOCF) Moss Attention Rating Scale |
Interventions
No single input causes any one or multiple limbic responses, nor does one treatment counteract their progression. Being aware of clinical signs is critical. In a time when therapists are often rushed by the realities of a full schedule or stressed by a third-party or short discharge demands, a clinician may inadvertently miss key signs and opportunities to treat. In addition, he or she may actually create a less optimal environment by physically moving faster than the pace best tolerated by the patient, who may need more time to process stimuli or to practice a target skill. The challenge for both the therapist and the patient is to find harmony within the given environment to allow for optimal outcomes. If a therapist misses these limbic signs that reflect the emotional state of the patient, the patient may perceive that the therapist doesn’t truly care about him or her as a person and thus the therapeutic alliance (patient/therapist bond) may be negatively affected.
The positive and negative effects of sensory input or the therapeutic environment on the limbic system, on motor output, and on behaviors are as much a part of the treatment plan as are the therapeutic activities. The limbic network state may support or limit consistent responses of the motor systems and thus dampen procedural learning and limit the success of the therapeutic setting. The optimal therapeutic environment for an individual is a positive limbic state where the system is open to learning and not trying to protect or withdraw from the learning environment. This state would be described as limbic neutral, or devoid of negative limbic influence, to ensure a strong foundation for both declarative and procedural learning to occur. Carryover of procedural learning into adaptive motor responses needs to be practiced with consistency. If a patient is frustrated or angry and simultaneously has hypertonicity, then a therapist might spend the entire session trying to decrease the abnormal motor response when the causation may be limbic. If the patient could be helped to neutralize the emotional system during the therapy session and achieve a limbic neutral state, then the specific problems could be treated effectively and with confidence that whatever motor problems exist are truly motor-driven. Differentiating the limbic network component from the motor control system when establishing treatment protocols has not typically been within the spectrum of a therapist’s skills, but is a skill that must be developed and practiced if rehabilitation professionals are to be considered movement specialists.
The critical responsibility of physical therapists is to strategically manage the interaction between sensory inputs of the therapeutic alliance and the therapeutic environment and thus the activation of the limbic network or limbic network state. Successful management of these components, paired with the correct tasks or therapeutic activities , serves to set a strong, neutral foundation for effective motor learning to occur. Initially practicing motor skills with a limbic neutral environment will lead to motor learning. The therapist should then slowly introduce limbic stimuli and continue to practice the motor learning. In that way, the motor system learns the control no matter the emotional environment society might introduce.
Therapeutic alliance: Clinician and patient
The concept of patient/client-centered therapy has evolved to become an important aspect of health care delivery. , The desire to improve or regain function can be self-motivated, but very often it is instilled through the clinician to the patient that his or her best interests and unique goals are the focus of the health care team. This belief is based on trust, hope, and attainable steps toward desired and realistic goals, all of which are limbic driven. Patients know when their desires, interests, and needs as unique and valued members of society are considered as part of therapy. They first believe and then recognize that they are persons with specific problems and desired outcomes when the therapist empowers them to link those beliefs to their own therapeutic environment. Although they may have specific medical diagnoses, be placed on clinical pathways, administered drugs, and sent off to the next facility in a couple of days, patients need to feel that they, as individuals, have not lost all individuality and that someone cares. That need is a feeling of security and safety that bonds a patient to a therapist along the journey of learning.
Before understanding and becoming compassionate regarding the needs of other people, such as patients with signs and symptoms of neurological problems, therapists need to understand their own limbic network and how it affects others who might interact with them. , The emotions felt by the therapist learner in pursuit of mastery and the ability to have the intellectual memory of the learning are also limbic functions. These behavioral or limbic responses will play an important role in our own lives, but also may either positively or negatively influence the lives and recovery of our patients/clients, and we will investigate this concept further in this chapter.
Many types of emotions create motivation, such as pleasure, reward processes, emotions associated with addiction, appreciation of financial benefits, amusement, sadness, humor, happiness, and depression. , Some emotions tend to drive learning, whereas others may discourage learning, whether that learning be cognitive or motor. If a person is fearful or apprehensive, motor performance and the ability to learn either a motor skill or intellectual information will be very different , , from that of an individual who feels safe, is given respect, and becomes part of the decision making process and thus functions inherently with control. , , , ,
An individual will naturally have feelings of loss and reservations or fears about the unknown future after injury to any part of the body, but especially the CNS. Yet that individual needs to be willing to experience the unknown to learn and adapt. The willingness, drive, and adaptability of that individual will affect the optimal plasticity of the CNS. The limbic network is a key player that drives and motivates that individual. The lack of awareness of that variable or its effect on patient performance will ultimately lead to questions and doubts about the effectiveness and efficacy of both assessment and intervention results. Similarly, if this system is overwhelmed either internally or externally, it will dramatically affect neuroplasticity and motor learning as well as cognitive, syntactical learning.
At the conclusion of this chapter it is hoped that therapists will comprehend why there is a need to learn to modulate or neutralize the limbic network so that patients can functionally control movement and experience cognitive learning. Then therapists need to strategically reintroduce emotions into the activity and allow the patient to once again experience movement and cognitive success during various levels of emotional demands and environments. This change in the emotional environment will create novelty of the task. This novelty is a critical motivator for learning and will drive neuroplasticity.
Yet a therapist deals with the limbic network of patients on a moment-to-moment functional level throughout the day. Fig. 4.9 illustrates the interlocking co-dependency of all major CNS components with the environment. At no time does any system stand in isolation. Thus, from a clinical perspective the therapist should always maintain focus on the whole environment and all major interactive components within it, while directing attention to any specific component. How the feedback (internal and external) to the patient’s CNS changes the neurochemistry and membrane potential, triggers memory, creates new pathways, or elicits other potential responses is not the responsibility of the clinician or therapist. The responsibility of the clinician is accurate documentation of changes and consistency of those changes toward desired patient outcomes. The professions that focus on movement science are interacting more closely with the neurosciences and other biological sciences and many related professions to unravel many of these mysteries and create better assessment and intervention procedures for future patients.

Because of the potency of the limbic network’s connections into the motor system, a therapist’s sensitivity to the patient’s emotional state would obviously be a key factor in understanding the motor responses observed during therapy. In Fig. 4.10 an entire spectrum of motor responses can be observed in four statues. A patient who feels safe can relax and participate in learning without strong emotional reactions. The woman being held in Fig. 4.10 A is safe and relaxed. The man and woman are interacting through touch with deep pressure, warmth, and compassion that are often observed in the patient-therapist interaction of an experienced or master clinician. In Fig. 4.10 B, the patient and clinician seem to flow together during the treatment as if they shared one motor system. When looking at the therapist and patient or looking at the man and woman in the statue, it becomes obvious that the two figures seem to flow together. In the statue, those two figures make one piece of art.

With clinical emphasis on patients generating and self-correcting motor programming, it would perhaps seem reasonable for a therapist to conclude that he or she need not, or should not, touch the patient. This conclusion may be accurate when considering the motor system in isolation and assuming that patients can self-correct errors in motor programs. When correction by the therapist is through words rather than touch, external feedback through the auditory system has replaced internal feedback from the somatosensory system. The voice, as well as touch, can be soothing and instill confidence. Yet language in and of itself will not replace the trust and safety felt both physically and emotionally through the deep pressure of touch as illustrated in Fig. 4.10 . Bonding and trust occur much more often through touch than through conversation. Recall, also, that verbal instructions require intact auditory processing and translation from declarative to procedural information, a cognitive ability that the patient may not possess.
Referring again to Fig. 4.10 A, the two men in the statue on the left demonstrate a lack of bonding. In fact, if the artist could have brought them closer together, they might just have rejected or repelled each other with greater intensity. If one of the men were the therapist and one the patient, little interaction would be occurring, and thus an assumption that learning is occurring is probably false. The therapist could do nothing to the other person (and vice versa) without that person perceiving the act as invasive, negative, or even disrespectful, with little consideration of the person’s individual values. The therapist’s responsibility is to open the patient’s receptiveness to learning, not to close it. ,
These pictures clearly illustrate two types of therapist-patient interactions, or therapeutic alliance . If an artist can clearly depict the tonal characteristics of emotion, certainly the therapist should be able to recognize those behaviors in the patient. , Achieving a limbic neutral state in the patient through an effective therapeutic alliance is an essential foundation for successful intervention and functional outcome. There are many core tenets and techniques necessary to effectively achieve this neutral state, internal and external therapeutic environment, and optimal performance and learning in the patient. The first component of a successful intervention is self-awareness on the part of the helping professional. “Behavioral activity can often tell us about the inner state of another or ourselves” (p. 19). The willingness to be aware of one’s own internal state increases the therapist’s ability to perceive subtleties in the patient’s responses. Differentiating how a patient learns and emotionally responds to therapy from how the clinician learns and emotionally responds to the patient/therapist bond and to therapeutic environments allows the therapist to create a situation where patient can more easily learn. Subsequently, the therapist must establish predictability for their patient’s interventions through Trust, Responsibility, Flexibility and Openness, and Vulnerability.
Trust.
Trust is a critical component of a successful therapy session. The therapist gains the patient’s trust by his or her actions. The therapist may also build trust through sincere acknowledgment that the patient has life-limiting functional problems and that those problems are limiting normal participation. Trust is further developed when the therapist’s words can be supported by data. When the therapist can illustrate the presence of functional limitations and generate a treatment plan with the patient using objective data, a bond of trust between the patient and the therapist are created. In today’s environment the use of reliable, valid, objective tests and measures allows for this form of communication, which has not existed to the same extent in the past. Honesty and truth lead to trust. ,
A trusting relationship is strengthened when an agreement or “contract” can be established that sets the boundaries for discomfort (fatigue, dizziness, nausea, and imbalance) or pain that the patient will experience within a therapeutic session. As one example, telling a person that you will not hurt them is a therapist-patient contract. If the therapist continually ranges a joint beyond a pain-free range, that behavior is dishonest and untruthful and will not lead to trust. Trust can be earned by stopping as soon as the patient verbalizes symptoms or shows pain with a body response such as a grimace. Being sensitive to a patient’s pain, no matter the cause, and working with the patient to eliminate that pain often lead to very strong bonding and trust that will lead to compliance and learning. Ignoring the pain may be perceived as insensitivity and lack of caring, which can lead to distrust and often resistance to learning or performance.
As another example, a patient with vestibular dysfunction associated with significant dizziness and nausea will experience symptoms within the course of recovery (adaptation and compensation), but those symptoms must be carefully controlled in intensity and duration. Symptoms poorly controlled can trigger an ANS or GAS cascade and elevate the limbic state, preventing learning and recovery and destroying trust.
Because these symptoms can be overt or covert, the therapist needs to be aware of both the physical and emotional responses of the patient. The use of analog or perceived exertion scales can be a valuable way to make the covert more overt to the therapist. Symptoms are valuable to the therapist as well as the patient to cooperatively create environments for change. The intensity of those stimuli need close monitoring because they can dramatically affect motor responses and ultimately overwhelm the CNS and prevent learning. Compliance to participation is limbic, and the limbic system has tremendous control over intentional movement, no matter the context of the environment.
Once a patient gives his or her trust, a clinician can freely move with the patient and little resistance caused by fear, reservations, or need to protect the self will be felt or observed. When the patient is limbic neutral (the limbic network is emotionally neutralized), the tightness or limitations in movement that are present on examination can be considered true impairments within those systems or subsystems. Examination and interventions at this time will more consistently reflect true motor performance. Once limbic neutral has been achieved and examination is complete, it is recommended, for example, that if the pain is a result of peripheral tightness or joint immobility, the therapist does not elicit pain during that session. Deal with those issues in the next session after gaining the trust of the patient. Trust by the therapist or the patient does not mean lack of awareness of potential danger. Trust means acceptance that although the danger is present, the potential for harm, pain, or disaster is very slight and the expected gain is worth the risk (in this case, delay in intervention). In Fig. 4.11 , the student’s trust that the instructor will not hurt her can be seen by her lack of protective responses and by her calm, relaxed body posture. The student is aware of the potential of the kick but trusts her life to the skills, control, and personal integrity of the teacher. Those same qualities are easily observed in patient-therapist interactions when watching a gifted clinician treat patients. The motor activities in a therapeutic setting may be less complex than in Fig. 4.11 , but in no way are they less stressful, less potentially harmful, or less frightening from the patient’s point of view.

In addition, therapists must first trust themselves enough to know that they can effect changes in their patients. , Understanding one’s own motor system, how it responds, and how to use one’s hands, arms, or entire body to move someone else is based partly on procedural skills, partly on declarative learning, and partly on self-confidence or self-trust. Trusting that one, as a therapist, has the skill to influence the motor response within the patient has a limbic component. If a therapist has self-doubts about therapeutic skills, that doubt will change performance, which will alter input to the patient. This altered input can potentially alter the patient’s output and vary the desired responses if the patient’s motor system cannot run independently.
Responsibility.
Very close to the concept of trust is the idea of responsibility. Accepting responsibility for our own behavior seems obvious and is accepted as part of a professional role. Accepting and allowing the patient the right to accept responsibility for her or his own motor environment are also key elements in creating a successful clinical environment and an independent person. , , ,
Fig. 4.12 illustrates the concept through the following example: The instructor asked the student to perform a motor act, in this case, to perform a kick to the teacher’s head. The kick was to be very strong or forceful and completed. The student was instructed not to hold back or stop the kick in any way, even though the kick was to come within a few inches of the teacher’s head. This placed tremendous responsibility on the student. One inch too far might dangerously hurt the instructor, yet one inch too short was not acceptable. The teacher knew the student had the skill, power, and control to perform the task and then passed the responsibility to the student. The student was hesitant to assume the responsibility, for the consequence of failure could have been very traumatic. However, the student trusted that the teacher would not ask for the behavior unless success was fairly guaranteed. That trust reduced anxiety and thus neutralized the neurochemical limbic effect on the motor system of the student, giving her optimal motor control over the act. Once the task was completed successfully, the student gained confidence and could repeat the task with less fear or emotional influence while gaining refinement over the motor skill.

Although the motor activities described in this example are complex and different from functional activities practiced within the clinic by therapists and patients, the dynamics of the environment relate consistently with patient-therapist roles and expectations. A gifted clinician knows that the patient has the potential to succeed. When asked to perform, the patient trusts the therapist and assumes responsibility for the act. The therapist can facilitate the movement or postural pattern through the use of his and her hands and own body movements, thereby ensuring that the patient succeeds. This feeling of success by the patient stimulates motivation for task repetition, which ultimately leads to learning. The incentive to repeat and learn becomes self-motivating and then becomes the responsibility of the patient. As the therapist relinquishes control and empowers the patient to more and more of the function, novelty to the learning is occurring.
Current literature has shown that people are more motivated by novelty and change than by success at mastery or accomplishment of a goal. , , The limbic complex and its interwoven network throughout the nervous system play a key role in this behavioral drive. The task itself can be simple, such as a weight shift, or as complex as getting dressed or climbing onto and off of a bus. No matter what the activity, the patient needs to accept responsibility for her or his own behavior before independence in motor functioning can be achieved. Although the motor function itself is not limbic, many variables that lead to success, self-motivation, and feelings of independence are directly related to limbic and prefrontal lobe circuitry. The variance and self-correction within the movement expression also create novelty and motivation to continue to practice. ,
As another clinical example of responsibility, in a patient with vestibular dysfunction and dizziness compounded by anxiety, symptoms of dizziness are necessary during treatment to drive CNS change. The therapist has a responsibility to prescribe the appropriate activities, dosage (intensity, timing, and so on), and environment to retrain sensory organization and balance (motor output). The patient can be given the responsibility of monitoring and managing her or his own symptoms within these activities. For instance, empowering the patient to determine the maximal level of dizziness within the therapeutic activity is an example of how the patient and therapist can develop an optimal level of interaction and potential positive therapeutic outcomes. A tool as simple as a verbal or visual analog scale can empower the patient to manage symptoms, dampen the limbic network response, and improve motor output for balance control.
Flexibility and openness.
Another component of a successful clinical environment deals with learning and flexibility on the part of the therapist. A master clinician sees and feels what is happening within the motor control output system of the patient. Letting go of preestablished beliefs concerning what will happen is difficult. , It is important for a clinician to be open to what is present as the motor expression. This openness is critical to actually identifying what is being expressed by the nervous system of the patient. Master clinicians do not get stuck on what they have been taught but use that as a foundation or springboard for additional learning. Learning is constantly correlated to memories and new experiences.
To the therapist, each patient is like a new map, sparsely drawn or sketchy at the beginning, but one that is constantly revised as the terrain (patient’s condition) changes. The initial medical diagnosis may link to many paths provided within the map, but the comorbidities can result in great variance among patients. That initial map might be a critical care pathway for the patient, given her or his neurological insult. That pathway is a map, but only a sketchy one, and may not even be a map that a particular patient falls within, in spite of his or her medical diagnosis. It is the therapist’s responsibility to evaluate the patient and determine whether that pathway or map will work or is working and when features of that map need to be changed, or altered, further. That is, the therapist must let go of an outdated map or treatment technique and create a new one as the environment and motor control system of the patient change. This transference or letting go of old maps or ideas is true for both the patient and the therapist. If a position, pattern, or technique is not working, then the clinician needs to change the map or directions of treatment and let the patient teach the therapist what will work. The ability to change and select new or alternative treatment techniques is based on the attitude of the therapist toward selecting alternative approaches. Willingness to be flexible and open to learning is based on confidence in oneself, a truly emotional strategy or limbic behavior. Master clinicians have learned that the answers to the patient’s puzzle are within the patient, not the textbooks.
Fig. 4.13 depicts two maps, each with a beginning point and a terminal outcome or goal. The parameters of the first map illustrate the boundaries of that therapist’s experience and education. The clinician, through training, can identify what would seem to be the most direct and efficient route toward the mutually identified goal of the therapist and patient. When the patient becomes a participant within the environment or map, what would seem like a direct path toward a goal might not be the easiest or most direct path for the patient. If empowerment of the patient leads to independence, then allowing, even encouraging, the patient to direct therapy may provide greater variability, force the patient to problem-solve, and lead to greater learning. The therapist needs to recognize when the patient is not going in the direction of the goal. For example, the patient is trying to perform a stand-pivot transfer and instead is falling. If it is important to practice transfers, then practicing falling is inappropriate and the environment (either internal or external) needs modification. Falling can be learned and practiced at another time. Once both strategies are learned, the therapist must empower the patient to take ownership of the map. In the examples of transferring, if the therapist asks the patient to practice transfers and if the patient starts to fall, a change in required motor behavior must be made and the opportunity given to the patient to self-correct. In that way the patient is gaining independent control over a variety of environmental contexts and outcomes. Within the same figure (see Fig. 4.13 ) is a second map. That second map might represent another professional’s interaction and goal with the same patient. It is during these overlapping interactions that both professionals can empower the patient to practice, and that practice will help lead to those functional goals established by both practitioners. In some situations, a clinician from one profession may guide a patient toward obtaining the functional skill necessary for a member of the second profession to begin guiding the patient toward the expected outcomes of the second profession. These interlocking dependencies of the patient and the professions are illustrated in Fig. 4.13 . If the patient begins therapy striving for the first goal and ends at the functional outcome of the second goal, then additional functional outcomes have been achieved and both professions interacted for the ultimate prognosis for the patient. That interaction requires respect and openness of both professionals toward each other as well as toward the patient. Those attitudes and ultimate behaviors are limbic-driven.

Matching maps should be a collaborative effort instead of coincidence. These collaborative efforts include interactions with all professions within the rehabilitation setting. Occupational and physical therapists are very familiar with collaboration, and both often approach interventions as a team effort. There are many additional therapists and individuals within that same setting who could also collaborate. Recreational therapists, psychologists, nurses, family members, and music therapists are but a few. Within a profession such as music therapy, the existence of two maps may overlap within a multidimensional environment. When a physical or occupational therapist needs to challenge a patient, the music therapist may be able to calm the system at the same time (overlapping maps). Research on affective responses to consonance and dissonance in music supports the creation of a map within a rehabilitation environment that could overlap with either physical, occupational, or speech therapy. Words such as relaxed or calm correlated positively with higher levels of consonance in the music, whereas adjectives associated with negative emotions (unpleasant, tense, irritable, annoying, dissonant, and angry) were found to correlate positively with higher levels of dissonance. Creating a whole environment where potential frustrations within motor learning could be balanced with higher levels of consonance in the music would potentially balance the limbic network emotional response within the overlapping maps and bring balance or stability to the limbic network’s influence on motor learning and control. A later study by Peretz and colleagues related the same variables to a happy-sad rating task. Given the research evidence for activity within the limbic network as it relates to music, , motor learning, and cognitive enhancement, a natural multiple map system would be easy to incorporate within a therapeutic setting. The clinician needs to appreciate the uniqueness of each map while holding onto the concept of the interaction of the two maps.
Vulnerability.
To receive input from a patient that is multivariable and simultaneous, a therapist has to be open to that information. If a clinician believes that he or she knows what each patient needs and how to get those behaviors before meeting the patient, then the case falls into a category of a recipe for treating the problem. Using the recipe does not mean the patient cannot learn or gain better perceptual and cognitive, affective, or motor control, but it does mean that the individuality of the person may be lost. A more individualized approach would allow the clinician to identify through behavioral responses the best way for the patient to learn how to sequence the learning, when to make demands of the patient, when to nurture, when to stop, when to continue, when to assist, when to have fun, when to laugh, or when to cry. An analogy might be going to a fast food restaurant versus a restaurant where each aspect of the meal is tailored to one’s taste. It does not mean that both restaurants are not selling digestible foods. It does mean that at one eating place the food is mass-produced with some choices, but individuality, with respect to the consumer, is not an aspect of the service. Unfortunately, managed care, limited visits, reduced time for treatment, and therapists’ level of frustration all are pushing therapeutic interventions toward a “one size fits all” philosophy that may increase the time needed for learning, not reduce it.
To be open totally to processing the individual differences of the patient, the clinician must be relaxed and nonthreatened, and feel no need to protect himself or herself from the external environment. This environment needs to project beyond the therapist-patient relations and envelop all disciplines interacting with the patient. For these interactions to occur, the clinician’s emotional state requires some vulnerability, allowing him or her to be open to new and as-yet-unanalyzed or unprocessed input. This vulnerability implies the role not of an expert who knows the answers beforehand but of an expert investigator. Being open must incorporate being sensitive not only to the variability of motor responses but also to the variability of emotional responses on the part of the patient. , This vulnerability leads to compassion, understanding, and acceptance of the patient as a unique human being. It can also be exhausting. Therapists need to learn ways to allow openness without taking on the emotional responsibility of each patient.
A clinical example can be used to help identify unique characteristics of the patient that will set the stage for effective learning. A 72-year-old woman has sustained a left CVA. She comes from a low socioeconomic background and was a housekeeper for 40 years for a wealthy family of high social standing. She addresses you (the therapist) with “yes, ma’am” or “no, ma’am” and does just what is asked, no more and no less. It may be very hard to empower this patient to assume responsibility for self-direction within the therapeutic setting. She may not feel she has the right or the power to assume such responsibilities within a setting (a medical facility) that may, from her perception, have high social status. The concept of empowerment may play a crucial role in regaining independent functional skill and control over her environment. , , However, to get optimal outcomes from this patient/clinician interaction, the therapist must find an environment, wherein this person feels worthwhile and empowered to regain functional movement to participate in life activities. This can be very challenging to a therapist but comes automatically to a master clinician. It means that both the patient and the therapist need to be vulnerable and open to the unknown for learning.
Not only does the therapist need to recognize when a patient has not accepted responsibility for motor control or has withdrawn from the therapy environment, but must also notice when the observable motor control is exaggerated by limbic reactions. Newspapers sometimes carry a story that generally reads as follows: “Seventy-nine-year-old, 109-pound arthritic grandmother picks up car by bumper to free trapped 3-year-old grandson.” We read these articles and doubt their validity, questioning the sensationalism used by the reporter. But we know that these events are real. That elderly lady picked up the car out of fear of severe injury to her grandchild. Emotions can create tremendously high tonal responses, either in a postural pattern, such as in a temper tantrum, or during a movement strategy, such as picking up a car. Conversely, fear can immobilize a person and make it impossible to create enough tone to run a motor program or actually move. Evaluating muscle power or tone production in relation to emotional state (versus a pure reflection of motor control) is an aspect of evaluation often overlooked.
Therapeutic environment
The therapeutic environment refers to both the internal and external environmental influences upon the individual that may positively or negatively influence a desired therapeutic outcome, and certainly the therapeutic alliance can contribute to that environment. The therapist must establish consistency and predictability within the treatment environment through (1) facilitating or ensuring neutral or stable function of the patient’s body systems or milieu; (2) facilitating a limbic-neutral learning environment; and (3) linking reward and other motivational factors to desired motor/task outcomes.
Facilitating the internal environment.
First, consider that the structure and function of an individual’s body systems influence function of the limbic network and prepare the brain for motor learning. More specifically, the neurobiology must include adequate neurochemicals to enable effective transmission between the key motor learning structures, including the emotional/behavioral prefrontal-limbic “forebrain” system, the cerebellum, and the motor control limbic midbrain and its cortical M1 projections. Similarly, the environment should avoid oxidative or other stressors that negatively impact effective neurochemical production or transmission.
Nutrition and hydration.
The functions of noradrenergic receptors of the locus coeruleus decline in the presence of hyperoxia-induced oxidative stress. The negative effect of hyperoxia and high levels of oxidative stress are blocked by antioxidants, as determined through animal study. , Foods high in anthocyanin and other antioxidants, or “super foods” such as key legumes, blueberries, cranberries, raspberries, strawberries, etc. have been shown to be neuroprotective during high periods of stress, which can lead to impaired motor learning through “accelerated aging” and cognitive decline. Motor learning and coordination as well as memory are also highly dependent upon calcium. Calcium-dependent plasticity is present in many synapses within the motor output and control loops, and reductions in calcium may result in deficits in long-term potentiation at these various synapses.
Adequate hydration plays a role in behavior (mood, motivation), cognitive (attention), and motor performance. Even small amounts of dehydration (even less than 5%) have been found to impair performance in tasks that require attention, psychomotor, or immediate memory skills.
Sleep and biorhythms.
Coordination of circadian body rhythm and sleep are critical for motor performance and learning, and as such, lack of or altered patterns of sleep have a negative effect upon performance and retention. , , Sleep influences and is influenced by levels of all of the monoamine neurotransmitters, thus affecting mood, cognition, and learning. ,
Motor learning occurs “off-line” during sleep and is important for memory consolidation that is unrelated to practice. It is likely that procedural memory is consolidated during stage 2 non-rapid eye movement (REM) sleep as well as in REM sleep. Interestingly, sleep of 60 to 90 minutes duration that occurs between practice and retention testing has shown to improve motor task performance when compared with those who stay awake.
Medication compliance and effectiveness.
Effectiveness of prescribed medication is a complex issue, extending beyond compliance, with recommended dosing to include evaluation of effectiveness of prescribed dosing. The physical and occupational therapist play an important, supportive evaluative and educational role in both of these areas. Serotonin and dopamine are two key neurotransmitters required for effective motor control, yet endogenous effectiveness can be impaired, and exogenous over- and under-medication can also reduce their effectiveness. In the case of serotonin, in addition to difficulties in determining optimal dosing for effective neuromodulation, up to 50% of individuals with depression are prone to discontinue their prescribed selective serotonin reuptake inhibiting (SSRI) medication. In the case of dopamine, there are two problems; first, the challenges associated with delivery of an effective dose of its precursor, L-dopa; and second, the changing effectiveness of dopamine replacement as the disease progresses in years and severity. Initially, upon starting therapy, the neurons of the substantia nigra are responsive to dopamine replacement, but they lose their sensitivity; the drug’s prolonged effectiveness drops after about 10 years of the disease. Within a given day, individuals may experience a sudden improvement of symptoms followed by a rapid decline in symptomatic relief, termed the “On-Off” phenomenon. The “On” phase is of longer duration earlier in the disease; efficacy is decreased, and this phase is of very short duration after years of therapeutic dosing. Overmedication can occur. In both cases, evaluation of movement control and/or changes can give the prescribing provider valuable information to assist with achieving optimal dosing.
Achieving an optimal external environment.
It is critical that a therapist facilitate a limbic-neutral environment to optimize motor learning. Obtaining a limbic-neutral impact or learning environment is critical before evaluating functional movement to accurately determine true motor system involvement. Highlighting this again as an example, it can be difficult to differentiate hypertonicity resulting from or worsened by stress from true spasticity or clinical hypertonicity. Patient motivation, alertness, and concentration are critical to motor learning because they determine how well we pay attention to the learning and execution of any motor task. These processes of learning and doing are inevitably intertwined: “We learn as we do, and we do only as well as we have learned.”
A neutral limbic environment is one that has mitigated all obvious external stressors (extraneous activities or interruptions, visual interference, auditory interference, olfactory interference, etc.) and internal, patient-specific stressors. These patient-specific stressors require observation and intuition on the part of the therapist, and may be triggered by past memories or experiences associated with less obvious individuals or sensory inputs within the environment. The treating professional can also become aware, during assessments or treatments, of environmental sensory input that may trigger stress responses in patients who have limbic network involvement or who may have experienced traumatic physical or emotional injuries. These triggers can be something as simple as the therapist’s tone of voice in a sentence to the patient or as complex as the multiple-noise environment of a busy rehabilitation setting. Some patients may need to be scheduled for early morning, during lunchtime, or in the late afternoon to provide a decrease in the auditory environment.
The same awareness can lead a therapist to identify something within the environment that could result in a positive emotional state for learning. Positive emotional states may create a limbic environment in which the therapist can link reward and pleasure associations to new motor sequences. As one example, music or pleasurable sounds can be used to help neutralize or balance the limbic influence on motor expression, as discussed earlier in this chapter and also in Chapter 39 . Within the therapeutic environment, it may be advantageous to purposefully facilitate positive or pleasant physical and affective experiences using music before engaging individuals in more challenging therapeutic interventions. Although it may be impractical to provide appropriate music selections on the basis of individual assessment in the therapeutic environment (e.g., physical, occupational, music), other modalities such as heat, massage, or ultrasound treatment may also elicit relaxed, receptive physical states.
Predictability of the environment is also an important consideration. Gentile presents a taxonomy for the acquisition of motor skills. In this taxonomy, Gentile describes a continuum of predictability, from “closed skills” that are highly predictable and self-paced to “open skills” that are less predictable and externally paced. A classic example of closed versus open would be a private room (closed) versus the middle of the gym (open), highlighted above. Closed, predictable activities provide the patient with the greatest control over themselves and their environment.
Decreasing stimulation versus increasing facilitation may lead to attention, calmness, and receptiveness to therapy. When the patient feels that control over her or his life has been returned, or at least the individual is consulted regarding decisions (informed consent as opposed to forced choices), resistance to therapy or movement is often released and stress is reduced. Even patients in a semi-comatose state can participate to some extent. As a clinician begins to move a minimally responsive patient, resistance may be encountered. If slight changes are made in rotation or trajectory of the movement pattern, the resistance is often lessened. If the clinician initially feels the resistance and overpowers it, total control has been taken from the patient. Instead, if the clinician moves the patient in ways her or his body is willing to be moved, respect has been shown and overstimulation potentially avoided. This highlights the importance of a physical or occupational therapist managing and strategically planning the treatment environment for all patients, not just for those with known head injury or trauma.
Linking motivation and reward.
These words are strong and reflect the importance of the limbic network in rehabilitation. Motivated behavior is geared toward reinforcement and reward, which are based on both internal and external feedback systems. Repeated experience of reinforcement and reward leads to learning, changed expectancy, changed behavior, and maintained performance. Emotional learning, which certainly involves the limbic network, is very hard to unlearn once the behavior has been reinforced over and over. , For that reason, motor behavior that is strongly linked to a negative emotional response might be a very difficult behavior to unlearn. For example, a patient who is willing to stand up and practice transfers just to get the therapist off his back is eliciting a movement sequence that is based on frustration or anger. When that same patient gets home and his spouse asks him to perform the same motor behavior, he may not be able to be successful. The spouse may say, “The therapist said you could.” The patient may respond, “I never did like him!” Thus repetition of motor performance with either the feeling of emotional neutrality or the feeling of success (positive reinforcement) is a critical element in the therapeutic setting. Consistently making the motor task more difficult just when the patient feels ready to succeed will tend to decrease positive reinforcement or reward, lessen the patient’s motivation to try, and decrease the probability of true independence once the patient leaves the clinical setting. When pressure is placed on therapists to produce changes quickly, repetition and thus long-term learning are often jeopardized, which may have a dramatic effect on the quality of the patient’s life and the long-term treatment effects once he or she leaves the medical facility. Motor control theory (see Chapter 3 ) coincides with limbic research regarding reinforcement. Inherent feedback within a variety of environmental contexts allowing for error with correction leads to greater retention. Repetition or the opportunity to practice a task (motor or cognitive) in which the individual desires to succeed will lead to long-term learning. Without practice or motivation the chance of successful motor learning is minimal to nonexistent.
Goal-directed or need-directed motor actions are the result of the nervous system structures acting as an interactive system. Within this system (see Fig. 4.14 ), all components share responsibilities. The limbic network and its cortical and subcortical components represent the most important level. In response to stimuli from the internal or external environment, the limbic network initiates motor activity out of the emotional aspect of feeling the need to act. This message is relayed to the sensory areas of the cerebral cortex, which could entail any one or all association areas for visual, auditory, olfactory, gustatory, tactile, or proprioceptive input. These areas are located in the prefrontal, occipital, parietal, and temporal lobes, where they analyze and integrate sensory input into an overall strategy of action or a general plan that meets the requirements of the task. Therefore these cortices recognize, select, and prepare to act as a response to relevant sensory cues when a state of arousal is provided by reticular input. The limbic cortex (uncus, parahippocampal gyrus/isthmus, cingulate gyrus, and septal nucleus) has even greater influence over the somatomotor cortices through the cingulate gyrus, both directly and indirectly through association areas. The thalamus, cerebellum, and basal ganglia contribute to the production of the specific motor plans. These messages of the general plan are relayed to the projection system. The limbic structures through the cingulate gyrus also have direct connections with the primary motor cortex. These circuits certainly have the potential to assist in driving fine motor activities through corticobulbar and corticospinal tract interactions. The thalamus, cerebellum, basal ganglia, and motor cortices (premotor, supplementary motor, and primary motor) contribute to the production of the specific motor plans. Messages regarding the sensory component of the general plan are relayed to the projection system, where they are transformed into refined motor programs. These plans are then projected throughout the motor system to modulate motor generators throughout the brain stem and spinal system. Limbic connections with (1) the cerebellum, basal ganglia, and frontal lobe , and (2) the motor generator within the brain stem enable further control of limbic instructions over motor control or expression. If the limbic and the cognitive systems decide not to act, goal-driven motor behavior will cease. An individual’s belief (emotional and spiritual) can inhibit even the most basic survival skills, as has been clearly shown in history when individuals with particular religious beliefs were pitted against vicious predators and those people chose not to defend themselves.

Within the projection system and motor planning complexes, the specifics are programmed and the tactics are given a strategy. In general, “what” is turned into “how” and “when.” The necessary parameters for coordinated movement are programmed within the motor complex as to intensity, sequencing, and timing to carry out the motor task. These programs, which incorporate upper motor neurons and interneurons, are then sent to the brain stem and spinal motor generators, which in turn, through lower motor neurons, send orders regarding the specific motor tasks to the musculoskeletal system. The actions performed by each subsystem within the entire limbic–motor control complex constantly loop back and communicate to all subsystems to allow for adjustments of intensity and duration and to determine whether the plan remains the best choice of responses to an ever-changing three-dimensional world. , ,
The limbic network has one more opportunity to modify and control the central pattern generators and control the body and limbs through direct connections to the spinal neuronetwork. , , That is, the limbic network can alter existing motor plans by modulating those generators up and down or altering specific nuclear clusters and varying the patterns themselves. Therapists as well as the general public see this in sports activities when emotions are high, no matter the emotion itself. Individuals who have excellent motor control over a specific sport may find high-level performance difficult as the stress of competition increases. Having control over emotional variance as well as motor variance with a functional activity is an accurate example of empowerment. Thus for a therapist to get a true picture of a patient’s motor system function, the limbic network should be flowing in a neutral or balanced state without strong emotions of any kind. Generally, that balance seems to reflect itself in a state of safety, trust, and compliance. Once the motor control has been achieved, then the therapist must reintroduce various emotional environments during the motor activity to be able to state that the patient is independent.
In summary, the limbic complex generates need-directed motor activity and communicates that intent throughout the motor system. , , , This step is vital to normal motor function and thus patient care. Patients need the opportunity to analyze correctly both their internal environment (their present and feedforward motor plans and their emotional state) and the external world around them requiring action on a task. The integration of all this information should produce the most appropriate strategy available to the patient for the current activity. These instructions must be correct, and the system capable of carrying out the motor activity, for effortless, coordinated movement expression to be observed. If the motor system is deficient, lack of adaptability will be observed in the patient. If the limbic complex is faulty, the same motor deficits might present themselves. The therapist must differentiate what is truly a motor system problem versus a limbic influence over the motor system problem.
Schmidt stresses the significance of “knowledge of results feedback” as being the information from the environment that provides the individual with insights into task requirements. This insight helps the motor system correctly select strategies that will successfully initiate and support the appropriate movement for accomplishing the task. This knowledge of results feedback is required for effective motor learning and for forming the correct motor programs for storage. ,
The reader may better understand the role of the limbic network in motor programming through a nonmedical example. Imagine that you are sitting in your new car. The dealer has filled the tank with necessary fuel. The engine, with all its wires and interlocking components, is totally functional. However, the engine will not perform without a mechanism to initiate its strategies or turn on the system. The basal ganglia or frontal lobe motor mechanism plays this role in the brain. The car has a starter motor. Yet the starter motor will not activate the motor system without the driver’s intent and motivation to turn the key and turn on the engine. The limbic complex serves this function in the brain. Once the key has been turned, the car is running and ready for guidance. Whether the driver chooses reverse or drive usually depends on prior learning unless this is a totally new experience. Once the gear has been selected, the motor system will program the car to run according to the driver’s desires. It can run fast or slow, but for the plan to change, both a purpose and a recognition that change is necessary are required. The car has the ability to adapt and self-regulate to many environmental variables, such as ruts or slick pavement, to continue running the feedforward program, just as many motor systems within the CNS, especially the cerebellum, perform that function. The limbic network may emotionally choose to drive fast, whereas one’s cognitive judgment may choose otherwise. The interactive result will drive the pedal and brake pressure and ultimately regulate the car. The components discussed play a critical role in the total function of the car, just as all the systems within the CNS play a vital role in regulating behavioral responses to the environment.
Brooks distinguishes insightful learning, which is programmed and leads to skills when the performer has gained insight into the requirements, from discontinuous movements, which need to be replaced by continuous ones. This process is hastened when patients understand and can demonstrate their understanding of what “they were expected to do.” Improvement of motor skills is possible by using programmed movement in goal-directed behavior. The reader must be cautioned to make sure that the patient’s attention is on the goal of the task and not on the components of the movement itself. The motor plan needs programming and practice without constant cognitive overriding. The limbic/frontal system helps drive the motor toward the identified task or abstract representation of a match between the motor planning sequence and the desired outcome. The importance of the goal being self-driven by the patient cannot be overemphasized. , , ,
Without knowledge of results, feedback, and insight into the requirements for goal-directed activity, the learning is performing by “rote,” which merely uses repetition without analysis, and meaningful learning or building of effective motor memory in the form of motor holograms will be minimal. Children with cognitive and limbic deficits can learn basic motor skills through repetition of practice, but the insights and ability to transfer that motor learning into other contexts will not be high.
Schmidt suggests that to elicit the highest level of function within the motor system and to enable insightful learning, therapy programs should be developed around goal-directed activities, which means a strong emotional context. These activities direct the patient to analyze the environmental requirements (both internal and external) by placing the patient in a situation that forces development of “appropriate strategies.” Goal-directed activities should be functional and thus involve motivation, meaningfulness, and selective attention. Functional and somatosensory retraining uses these concepts as part of the intervention. Specific techniques such as proprioceptive neuromuscular facilitation, neurodevelopmental therapy, the Rood method, and the Feldenkrais method can be incorporated into goal-directed activities in the therapy programs, as can any treatment approach, as long as it identifies those aspects of motor control and learning that lead to retention and future performance and allows the patient to self-correct. With insights into the learned skills, patients will be better able to adjust these to meet the specific requirements of different environments and needs, using knowledge of response feedback to guide them. The message then is to design exercise activities or programs that are meaningful and need-directed, to motivate patients into insightful goal-directed learning. Thus understanding the specific goals of the patient, patient-centered learning, is critical and will be obtained only by interaction with that patient as a person with needs, desires, and anticipated outcomes. , A therapist cannot assume that “someone wants to do something.” The goal of running a bank may seem very different from that of birdwatching in the mountains, yet both may require ambulatory skills. If a patient does not wish to return to work, then a friendly smile and the statement, “Hi, I’m your therapist, and I’m going to get you up and walking so you can get back to work,” may lead to resistance and decreased motivation. In contrast, a therapist who knows the goal of the patient may help him or her become highly motivated to ambulate; that patient may be present in the clinic every day to meet the goal of birdwatching in the mountains, although never wishing to walk back into the office again.
Therapeutic activities
Strategic exercise prescription has a significant effect upon limbic activity and motor learning. First, exercise has a positive effect on high and low limbic network states, such as seen in chronic stress, anxiety (high), or in depression (low), for example. A recent Cochrane Collaboration Review investigated the effect of exercise upon depression through meta-analysis. Though there were methodological errors, the authors were able to conclude that exercise is moderately more effective than a control intervention for reducing symptoms of depression, but not more effective than psychological or pharmacological interventions alone. Individuals with depression can be considered to exhibit a low limbic state, which has been discussed as impairing motor learning. No similar systematic review has been published through the Cochrane Collaboration for anxiety. No Clinical Practice Guidelines could be identified to guide exercise prescription for the depression or anxiety populations, which would be reflective of limbic network involvement.
Exercise similarly establishes an internal environment favorable for neuroplasticity and for neuroprotection. Specifically, high-intensity aerobic exercise facilitates an exercise-induced response of peripheral brain-derived neurotrophic factor (BDNF), which improves the ability to achieve neuroplastic change and motor learning. For a discussion of neuroplasticity and the positive effects of exercise on neuroplastic change and motor learning in the cortex, the reader is referred to Chapter 3 . What is less discussed is the effect of exercise on interaction between motor and limbic networks for effective motor learning. High intensity exercise also improves neurotransmission of dopamine and glutamate in the basal ganglia, both of which play important roles in the initiation and control of movement. , As such, a positive neuroprotective effect can decrease the rate of degeneration of the dopaminergic cells within the basal ganglia; this can reduce the amount of medication required by an individual to control their symptoms and improve learning and carry-over to function.
Patient education concerning all aspects of limbic network influence on motor performance and learning can provide insight, enabling patients to achieve acceptance and engagement. In particular, education in those aspects of their internal and external environment within their control (sensory inputs, sleep, pharmacological management, etc.) may help maximize patients’ participation in interventions, activities, and in societal roles.
Emerging interventions
Advances in surgical neuromodulation of the limbic circuitry underlying these disorders offer hope for individuals suffering from addiction, PTSD, or memory disorders. Emerging evidence shows that deep brain stimulation (DBS) to specific nuclei within the limbic system may be at least as successful as traditional pharmacological therapies to improve dynamic memory and learning processes. In a single patient case investigation, Freund and colleagues reported that treatment with DBS of the nucleus basalis in the basal forebrain enhanced the synthesis of nerve growth factor, resulting in markedly improved cognitive function, specifically attention, concentration, alertness, and drive. The critical importance of these cognitive functions to motor learning has been stated frequently throughout the evidence presented within this chapter.
Advancements in genetic profiling and genetic modification bear close attention, with meaningful influence upon all aspects of brain function. As one example, it is understood that adequate dopamine must be present for brain plasticity, motor skill acquisition, and motor learning, given projections from the midbrain VTA to the M1 primary motor cortex. Electrical stimulation of the VTA resulted in improved function of the dopaminergic pathway and improved expression of the gene c-fos in M1 by more than 142%, which has been shown to improve the function of the primary motor cortex. Five genetic polymorphisms have been identified as having effect upon dopaminergic neurotransmission. Specifically the DRD2/ANKK1 genotype has been significantly associated with motor learning and its modulation through medication management of dopamine precursors (L-dopa). These genetic variations help to explain the individual differences in patient response to medications designed to improve motor performance (in Parkinson disease [PD]); insight into pharmacological management can be based upon these biological subgroups. The knowledge of genetic variation and specific polymorphisms that negatively impact motor learning can direct future efforts in genetic modification. The knowledge of genetic advancements may assist physical and occupational therapists to help their patients understand their unique positive, neutral, or negative responses to interventions or pharmacological management.
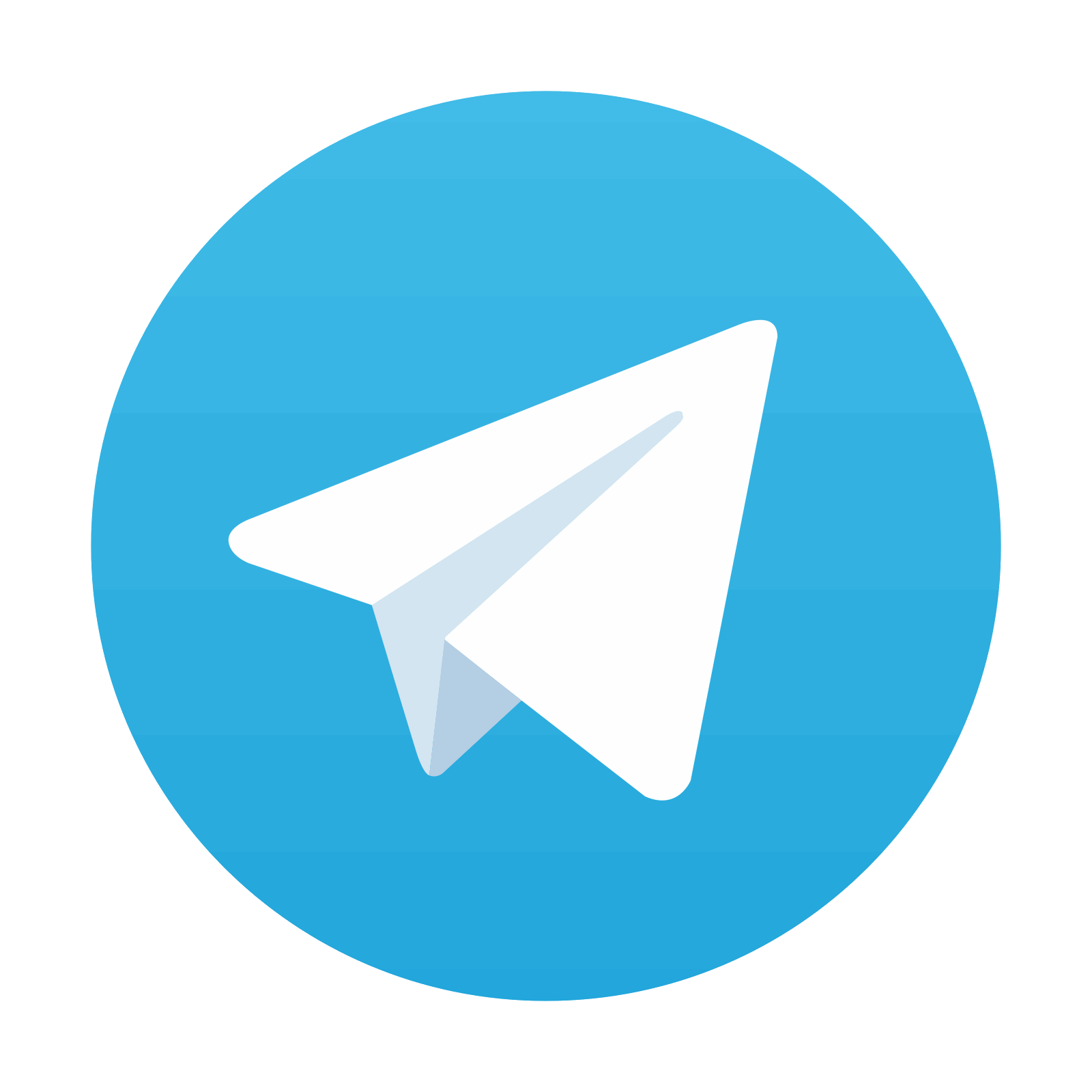
Stay updated, free articles. Join our Telegram channel

Full access? Get Clinical Tree
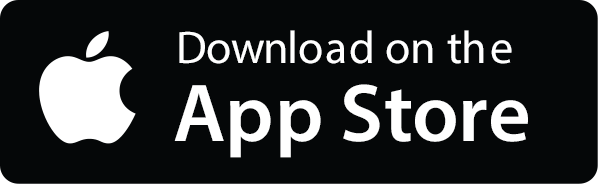
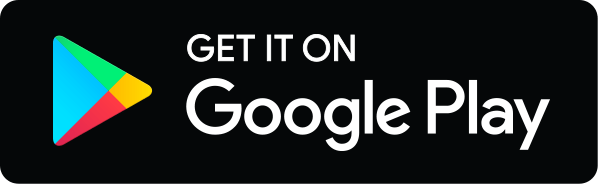