Chapter 10 The lumbar spine
Functions of the lumbar spine
Movements of the lumbar vertebrae include flexion, extension, some bilateral sideflexion, a small amount of axial rotation, distraction, compression, anterior/posterior translation and medial/lateral translation (Fig. 10.1).
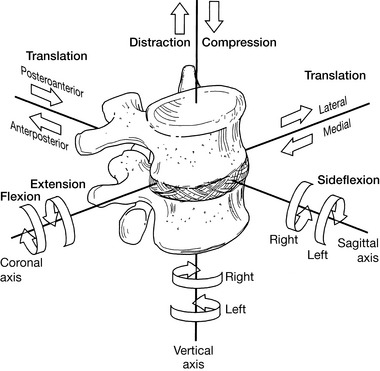
Figure 10.1 In mechanical terms, there is the potential for 12 degrees of motion of the lumbar vertebrae
(reproduced with permission from Lee (2004)).
Coupling
Under normal conditions these spinal movements are usually combined (coupling); for example, the combination of sagittal rotation and translation occurring during flexion and extension (Bogduk 2005). Rasch & Burke (1978) created a generally accepted, extremely simple definition of coupling: ‘Lateral flexion of the spine is always accompanied by some rotation.’
It is rare for a single movement to take place rather than these common combinations, that appear to be dependent on the structural features that regulate and constrain them, notably the spine itself, the intervertebral discs, the facet joints, the ligaments and the myofascial network, which both supports and moves all the other structures (Waddell 1998). Zhao et al (2008) used stereophotogrammetry techniques to record movements of the spine in 3D, during the gait cycle. They observed that the lateral and transverse rotations of the lumbar segments have the largest range of motion in the spine, reinforcing the notion that this region plays a crucial role in modulating upper body positioning, while maintaining body stability. All the subjects tested demonstrated that adjacent regions of the thoracic, lumbar spines and pelvic segments, exhibited coupled rotations during gait – in both the transverse and frontal planes. However, these patterns of rotation varied considerably from one subject to the next – indicating that they are individual, and not universally predictable. It is suggested that these individual patterns may be due to variable muscular features, which differ significantly between individuals.
In many instances, structural modifications associated with functional changes will also be visibly or palpably identifiable. Of course, this structure-function continuum also applies to the normal physiological functions of structures, based on the intrinsic architectural design of the area. For example, in the thoracic spine functional movements (such as extension) are limited by the structural features of the vertebrae, which effectively prevent backward bending. Sideflexion as well as flexion potential in the thoracic spine is also limited by the inter- and supraspinal ligaments and by the ribs, especially in the upper thoracic region. The flexion potential of thoracic vertebrae, therefore, exists mainly in the lower thoracic segments, in which rib fixation is less of a factor. Rotation potential for the thoracic region is freer, however, with the axis of rotation being in the mid-thoracic area. As Lewit (1985) explains:
Lumbar vertebral structure (Bogduk 2005, Gray’s anatomy 2005, Lee 2004, Ward 1997)
A healthy representative lumbar vertebra consists of a number of distinctive parts (Fig. 10.2).
• Vertebral body, which is level along its superior and inferior surfaces, with slightly concave anterior and lateral surfaces. The body is constructed of cancellous bone (structured for strength and lightness) as a honeycomb of struts or rods, known as trabeculae, running vertically as well as transversely. Additional hydraulic strength is created within the vertebral body by the presence of blood. Waddell (1998) elaborates: ‘We tend to think of bone as rigid but that is not strictly true. Vertebrae are six times stiffer and three times thicker than the discs and only allow half the deformation, but they do have some elasticity’. Bogduk (2005) paints a vivid picture of the interior of the vertebral body: ‘When filled with blood, the trabeculated cavity of the vertebral body appears like a sponge, and for this reason it is sometimes referred to as the vertebral spongiosa’.
• The sponge-like honeycomb of the body is surrounded by a raised rim of smoother bone known as the ring apophysis.
• From the posterior surface of the vertebral body two strong projections emerge, the pedicles, which are part of the neural arch that surrounds and protects the spinal cord.
• The remainder of this neural arch comprises the laminae, which project from each pedicle before curving toward the mid-line where they merge into each other and become the spinous process.
• The function of the hollow, thick-walled, cylindrical pedicles is to transmit bending and tension forces between the potentially highly mobile body of the vertebra (anterior element) and the posterior element, with its muscular attachments and projecting leverage arms (transverse, spinous processes, etc.).
• Bogduk (2005) notes that it is significant that all the muscles acting directly on the lumbar vertebrae pull inferiorly obliging forces to be transmitted to the vertebral body through the pedicles.
• Projecting laterally from the junction of the pedicles and the laminae are the transverse processes and projecting from the inferior surface of each transverse process, close to the pedicle, is the accessory process. Superior and medial to the accessory process, separated from it by a notch, lies the mamillary (‘breast-like’) process.
• Projecting posteriorly from the junction of the laminae is the spinous process. The laminae seem to act as stabilizing structures that absorb or transfer forces imposed on the spinous and inferior articular processes.
• Between the superior and inferior articular processes lies the pars interarticularis, that part of the laminae which copes with the transfer of horizontally and vertically oriented stresses. If this is not adequate to the stresses imposed on it, stress fractures can occur.
• The transverse, spinous and various accessory processes all provide anchorage for muscular attachments. The larger and longer the process involved, the greater the degree of leverage potential the attaching muscle will have on the posterior elements of the spine. Some psoas fibers and the crura of the diaphragm are the only significant muscular attachments to the bodies of the vertebrae. These are not thought to exert any primary action on the segments to which they attach by some (Bogduk 2005) while others (Kapandji 1974, Platzer 2004, Rothstein et al 1991, Travell & Simons 1992) vary in opinion as to lumbar spinal movement (see discussion of psoas on p. 287).
• Inferior and lateral to the laminae are specialized hook-like structures known as the inferior articular processes, which articulate with the superior articular processes of the vertebra below, which project superiorly from the junction of the pedicles and the laminae. The synovial joints thus formed provide an excellent locking mechanism, which helps to prevent excessive rotation, as well as anterior translation (glide) movement of one segment on another.
• Smooth surfaces, covered with cartilage, exist on the medial surfaces of the two superior articular processes, as well as on the lateral surfaces of the two inferior articular processes. These are the articular facets of these articular processes, which make up the zygapophysial (‘facet’) joints.
• The architectural design of the vertebral bodies is such that they can slide in all directions in relation to each other’s endplate surfaces. As Bogduk (2005) expresses it: ‘There are no hooks, bumps or ridges on the vertebral bodies that prevent gliding or twisting movements between them. Lacking such features, the vertebral bodies are totally dependent on other structures for stability in the horizontal plane, and foremost among these are the posterior elements of the vertebrae’– namely the laminae, the articular and spinous processes and, to a lesser degree, the annular fibers of the disc and the ligaments of each segment.
• The posterior elements collectively comprise an uneven mass of bone characterized by a variety of projections which manage the multiple forces imposed on the vertebrae.
• Some key characteristics of the five lumbar vertebrae include the following (Bogduk 2005, Gray’s anatomy 2005, Lee 2004, Ward 1997).
• Lumbar vertebrae are relatively large in size compared with thoracic vertebrae.
• The vertebral body of L5 is wide transversely and vertically deep anteriorly (so contributing to the sacrovertebral angle).
• There is an absence of costal facets and transverse foramina, which are present in the vertebrae above the lumbar region.
• Transverse processes protrude virtually horizontally.
• The superior articular facets are angled posteromedially
• The inferior articular facets are angled anterolaterally
• The 5th lumbar vertebrae, which is itself very large, has ‘more substantial’ transverse processes (Gray’s anatomy 2005), compared with other lumbar vertebrae in which transverse processes are long and thin.
• The lumbar transverse processes increase in length from the first to the third and then shorten.
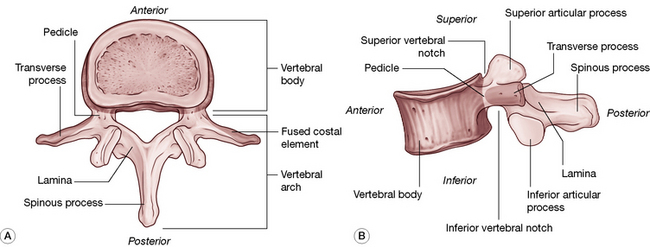
Figure 10.2 The parts of a typical lumbar vertebra. A: Superior view. B: Lateral view.
(Reproduced, with permission, from Gray’s anatomy for students, 2nd edn, 2010, Churchill Livingstone)
The intervertebral disc joint (see also Volume 1, Figs 11.2 & 11.5)
• The three features of the intervertebral disc are the peripheral annulus fibrosus, the core nucleus pulposus and the vertebral endplates that lie superiorly and inferiorly and which attach the disc to the vertebrae above and below. Bogduk (2005) suggests that the endplates are regarded as part of the intervertebral disc rather than part of the vertebral body, since they are strongly bound to the disc and only weakly attached to the vertebral body.
• The annulus and the nucleus pulposus meld and merge into one another rather than having distinct boundaries.
• The annulus fibrosus is a superbly configured collagen construction, made up of 10–20 spiral and inter-digitated layers, the lamellae, capable of restraining movements and stabilizing the joint (Cailliet 1995) (see Volume 1, Fig. 11.2). Each fiber is a trihelix chain of numerous amino acids, which gives it an element of elasticity, making each annulus fibrosus, in all but name, a ligamentous structure. As Bogduk (2005) puts it, ‘The anuli fibrosi can be construed as the principal ligaments of the vertebral bodies. [It] functions as a ligament in resisting distraction, bending, sliding and twisting movements of the intervertebral joint … It is only during weightbearing that it functions in concert with the nucleus pulposus’.
• The annular fibers course on a diagonal to connect adjacent vertebral endplates. Each layer of fibers lies in the opposite direction to the previous layer so that when one layer is stretched by rotation or shearing forces, the adjacent layer is relaxed (see Volume 1, Fig. 11.2).
• The disc fibers may be stretched to their physiological length and will recoil when the force is released. If stretched beyond their physiological length, the amino acid chains may be damaged and will no longer recoil.
• The vertebral endplates comprise a layer of cartilage that covers the superior or inferior surface of the body of the vertebra and is encircled by the ring apophysis. The endplate attaches the body to the disc itself, completely covering the nucleus pulposus and, to a large extent, the annulus. The attachment of the endplate to the vertebral body is far weaker than is its attachment to the disc.
• The nucleus, when in a young and healthy state, is an incompressible but deformable paste-like, semi-fluid proteoglycan gel (approximately 80% of which is water) which is designed to conduct and tolerate pressure, relating mainly to weight bearing. With age it dessicates and loses many of its valuable protective properties.
• Though the discs have a vascular supply in the early stages of life, by the third decade the disc is avascular and nutrition to the disc is thereafter in part supplied through imbibition, where alternating compression and relaxation create a sponge-like induction of fluids.
• As long as the container remains elastic, the gel cannot be compressed but can merely deform in response to any external pressure applied to it.
• Since the nucleus conforms to the laws of fluids under pressure, when the disc is at rest, external pressure applied to the disc will be transmitted in all directions, according to Pascal’s law. When external forces compress the disc, the nucleus deforms and the annular fibers, while remaining taut, bulge.
• While the design offers optimal conditions of hydraulic support as well as numerous combinations of movements, postural distortions brought on by overuse, strain and trauma can lead to degenerative changes in the disc, usually accompanied by muscular dysfunction and often associated with chronic pain.
• The permeability of the endplates and the disc is enhanced by exercise and lessens with age.
• There is an approximately 20% reduction in disc volume and height through the day, due to gravity and activity. In health, the disc volume is restored after rest (lying down) through osmotic forces (imbibition).
The zygapophysial (facet) joints
• The zygapophysial (facet) joints (see discussion on p. 221 as to terminology) carry approximately a quarter of the weight of the trunk under normal conditions, although Waddell (1998) reports: ‘This may rise to 70% when the discs narrow with degenerative changes’.
• The oval-shaped facet joints provide stability and facilitate movements such as rotation and translation (shunt, glide, shift) and are exposed to shearing and compression forces (Figs 10.3, 10.4). Figure 10.3 depicts moderate differences between the cervical, thoracic and lumbar joints, which ultimately affects the range and degree of motion of each vertebra as well as that of the spinal region.
• The degree to which a pair of facet joints achieves its influence on rotation and displacement depends on the relative curved or flat nature of the surfaces involved (Fig. 10.5).
• The articular surface of each facet joint is cartilaginous and is surrounded on its dorsal, superior and inferior margins by a collagen-based capsule. Anteriorly, the ligamentum flavum borders the facet joint capsule.
• The facet joint structure houses fat as well as meniscoid structures, composed of connective tissue, adipose tissue and fibroadipose tissue. These are interpreted (there is no consensus – Bogduk 2005) as acting as shock absorbers or protective surfaces.
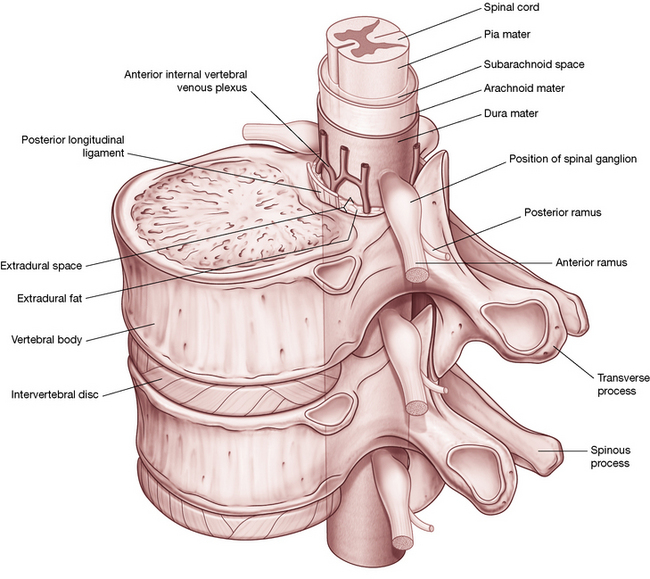
Figure 10.4 If an intervertebral joint is compressed (1), the inferior articular processes of the upper vertebra impact the laminae below (2), allowing weight to be transmitted through the inferior articular processes (3). Note the almost vertical angle of the facet joint, a factor of particular importance in application of SNAGs, as described in Box 10.3.
Box 10.3 Sustained natural apophyseal glides (snags) for the lumbar spine
Mulligan (1999), who developed the SNAGs approach, writes the following in discussing the various theories on the origin of low back pain.
SNAGs application method
Note: The segment to be treated using SNAGs, which is restricted or painful on movement, should previously have been identified by motion palpation and/or direct manual palpation. Also note that the application of SNAGs in the lumbar region calls for a stabilization ‘strap’/belt which links the practitioner and the patient (see Fig. 10.15). Straps of this sort (seatbelt width and materials) are widely used in physical and manual therapy and can be obtained from suppliers to the physical therapy profession.
The patient is seated on a treatment table with legs over the side (Fig. 10.15A) or stands alongside the table (Fig. 10.15B). If seated, a strap/belt is placed around the patient’s lower abdomen, below the anterior iliac spines, and loops around the practitioner’s upper thighs (ideally below the hip joints) (Fig. 10.15A). If the patient is standing a similar strap connection should be established (see Fig. 10.15B) and the patient should ensure that he maintains the knees in slight flexion throughout the procedure in order to minimize hamstring or neural tension influences.
Mulligan (1999) suggests that at times the ulnar border contact of the treatment hand should be made unilaterally, rather than centrally as described above.
When treating the lumbosacral segment (L5 on the sacrum) Mulligan (1999) suggests:
(Reproduced, with permission, from Gray’s anatomy for students, 2nd edn, 2010, Churchill Livingstone)
Ligaments
• A major function of viscoelastic structures such as ligaments is to establish limits to movement while providing stability.
• Ligaments are also sensory organs and have significant input to sensation and reflexive/synergistic activation of muscles. In some joints, such as the intervertebral joints of the spine, the role of the muscles as restraints is amplified (Solomonow 2009).
• The two major ligaments of the spine are the extremely powerful anterior and posterior longitudinal ligaments.
• The anterior longitudinal ligament extends from the sacrum to the cervical spine, with some fibers extending from one segment to the next and others extending for up to five segments.
• The attachment of the fibers of the anterior longitudinal ligament is into bone, mainly the anterior margin of the lumbar vertebral bodies and also, via collagen fibers, to the concave anterior surface of the bodies.
• The anterior longitudinal ligament is distinct from the annulus fibrosus, which attaches mainly to the vertebral endplates. It also merges with the crura of the diaphragm on the anterior surfaces of (at least) the first three lumbar vertebrae.
• Bogduk (2005) suggests: ‘… detailed examination of the crura and their attachments suggests that many of the tendinous fibers of the crura [of the diaphragm] are prolonged caudally beyond the upper three lumbar vertebrae… [and that]… these tendons constitute much of what has otherwise been interpreted as the lumbar anterior longitudinal ligament. Thus it may be that [this] is, to a greater or lesser extent, not strictly a ligament but more a prolonged tendon attachment’.
• The posterior longitudinal ligament contains fibers of different lengths, some of which span two discs while others span up to five discs, attaching from the superior margin of one vertebrae to insert into bone on the inferior margin several segments above. As with the anterior longitudinal ligament, the posterior one protects against undue separation forces and offers protection to the deeper structures.
• As indicated previously, the annuli fibrosi should also be regarded as ligamentous, due to their task of connecting adjacent vertebrae and restricting their excessive movements. Since the annulus fibrosus resists vertical distraction and other movements of the intervertebral joint, it is in effect acting as a ligament during all spinal movements, as well as offering structural protection to the nucleus.
• The ligamentum flavum, the most elastic of the spinal ligaments, connects the laminae of one vertebra to the laminae of the vertebra below it, while laterally it forms the anterior capsule of the facet joint. The precise purpose of the elastic nature of this ligament remains to be established but Bogduk (2005) points out that its location near the neural structures is likely associated with its high degree of elastic properties. He notes that were it more collagenous in nature, it would buckle upon relaxation and could encroach upon neural structures. With its higher elastic properties, it will simply retract to its normal thickness without buckling, thereby reducing the likelihood of neural compression (Fig. 10.6).
• The largely collagen (i.e. inelastic) based interspinous ligaments attach neighboring spinous processes to each other. There are ventral, middle and dorsal aspects to the ligament, with the latter merging to a great extent with the supraspinous ligament (Fig. 10.7).
• The supraspinous ligament attaches to and joins adjacent spinous processes, crossing the interspinous space. The reality of its claim to be a ligament is challenged, since much of it comprises tendinous fibers that derive from the thoracolumbar fascia and back muscles, such as the multifidi and the aponeurosis of longissimus thoracis.
• The iliolumbar ligaments occur bilaterally and link the transverse processes of L5 to the ilium, preventing anterior drift of L5 on the sacrum. The iliolumbar ligaments, which are apparently not present in infants where the tissue is muscular, gradually become ligamentous during adult life and later in life degenerate into fatty tissue. Parts of the superior aspects of the iliolumbar ligament arise from fascia surrounding quadratus lumborum (Thompson 2001). See further discussion of this ligament on p. 220 (Fig. 10.8).
• The intertransverse ligaments comprise sheets of connective tissue that extend from one transverse process to the next. Bogduk (2005) notes that they are more membranous than ligamentous, fulfilling a role of separating and defining particular prevertebral compartments that divide the anterior and posterior lumbar musculature.
• Approximately 50% of individuals have transforaminal ligaments, which span various aspects of the intervertebral foramina. As with the intertransverse ligaments, these are more fascial than ligamentous.
• The so-called ‘mamillo-accessory’ ligament is a tendon-like collagen structure, running from the mamillary process to the ipsilateral accessory process. Thus, because it links parts of the same bone, it is not a true ligament. It frequently ossifies in later life with no apparent negative effects.
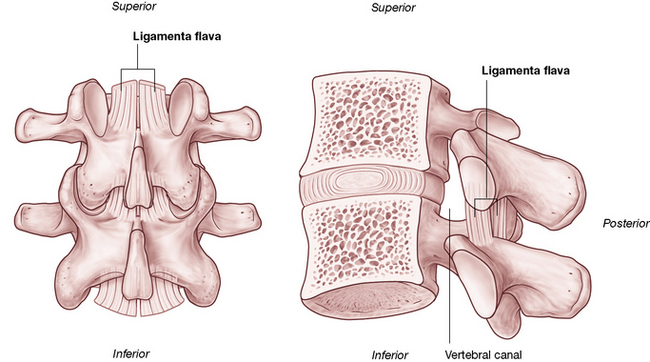
Figure 10.6 The ligamentum flavum. A: Posterior view. B: Lateral review. (note proximity to the spinal canal.
(Reproduced, with permission, from Gray’s anatomy for students, 2nd edn, 2010, Churchill Livingstone)
Additional notes on associated spinal structures
Lumbar biomechanics are discussed at length by Bogduk (2005) and are well illustrated by Kapandji (1974). The biomechanics of the cervical region and thoracic region, as well as the structure of the disc components, are discussed at length in Volume 1 of this text. Some points of particular interest to the lumbar region are listed here.
• It is virtually automatic for sidebending of a vertebral segment to be accompanied by rotation and, in the lumbar spine, this is primarily (but not always) to the opposite side (type 1) (Zhao et al 2008) (Fig. 10.9).
• L5, however, sidebends to the same side during flexion and rotation (type 2) and the ‘L4–5 joint exhibits no particular bias; in some subjects the coupling is ipsilateral while in others it is contralateral’ (Bogduk 2005).
• Descending through the 1st lumbar vertebral foramen is the conus medullaris of the spinal cord.
• The lower lumbar foramina house the cauda equina and the spinal meninges.
• The cord may be traumatized in numerous ways and may also become ischemic due to spinal stenosis, a narrowing of the neural canal, which may be exacerbated by osteophyte formation.
• Other factors that might cause impingement or irritation of the cord include disc protrusion, as well as excessive laxity allowing an undue degree of vertebral translation anteroposteriorly and from side to side.
• The nerve plexus that supplies the lower extremity derives from the cord at the lumbar and sacral levels, which means that any nerve root impingement (disc protrusion, osteophyte pressure, etc.) of the lumbar intervertebral foramina could produce both local symptoms as well as neurological symptoms involving the lower extremity.
• Postural dysfunction, once established, tends to lead to biomechanical adaptation and a self-perpetuating, habitual pattern of use in which dysfunction begets ever greater dysfunction.
• It is important to consider global posture rather than local factors alone when assessing biomechanical dysfunction, together with awareness of previous compensation patterns. While some compensatory patterns can be seen as common, almost ‘normal’, how the body adapts when traumas (even minor ones) and/or new postural strains are imposed will be strongly influenced by existing compensatory patterns. In other words, there is a degree of unpredictability where compensations are concerned, especially when recent demands are overlaid onto existing adaptation patterns.
• Structural compensations can involve a variety of influences, for example as the body attempts to maintain the eyes and ears in an ideally level position. Such adaptations will almost always involve the cervical region and may involve lumbar compensations. These adaptations will be superimposed on whatever additional compensatory changes have already occurred in that region. The practitioner, therefore, has to keep in mind that what is presented and observed may represent acute problems evolving out of chronic adaptive patterns. ‘Unpeeling’ the layers of the problem to reveal core, treatable obstacles to recovery of normal function involves patience and skill.
• Chapter 2 examines posture and postural compensations in more depth, as do the remaining technique chapters where the pelvis and feet, the very foundations of the body’s structural support, are discussed.
• The following muscular attachments are of particular importance in the lumbar region.
• The crura of the diaphragm attaches to the 2nd and 3rd lumbar vertebral bodies, lateral to the anterior ligament.
• Psoas major attaches posterolaterally to the upper and lower margins of all the lumbar vertebral bodies (Gray’s anatomy 2005).
• The spinal processes serve as attachments for the posterior lamellae of the thoracolumbar fascia, erector spinae, spinalis thoracis, multifidi, interspinal muscles and ligaments and the supraspinous ligaments (Fig. 10.10).
• There is a vertical ridge on all the lumbar transverse processes, close to the tip, to which the anterior layer of the thoracolumbar fascia attaches and which separates a medial area, to which psoas major attaches, from a lateral area for quadratus lumborum attachment.
• The medial and lateral arcuate ligaments attach to the transverse processes of L1, while the iliolumbar ligament attaches to the transverse processes of L5 (and sometimes weakly to L4).
• The posterior aspects of the lumbar transverse processes are covered by deep dorsal muscles, with attachments from longissimus thoracis.
• The lateral intertransverse muscles attach to the upper and lower borders of adjacent transverse processes.
Transitional areas
Adaptive compensation forces (involving joints, ligaments, muscles and fascia), feeding upwards from the pelvic region or downwards from the upper trunk, commonly localize at the level of transition between the relatively inflexible thoracic spine and the relatively flexible lumbar spine: the thoracolumbar junction. The T12-L1 coupling is an important transitional segment as it is where free rotation is abruptly forbidden and where flexion and extension are suddenly and significantly allowed. As Waddell (1998) puts it: The transitional regions between fixed and flexible parts of the spine have greater functional demands, which might explain why these are the areas of most symptoms.
The spinal column: its structure and function
As noted, the integrated structure of the spine, together with its nerve supply and muscles, serves various functions that offer stability, mobility and defense. Each of these functions imposes different demands on the way the structure is constructed. A need to offer support alone might have resulted in a more rigid structure, while if protective functions were the dominant requirement, greater mass might have developed. A compromise has evolved, combining mobility with relative rigidity and bulk. As Vleeming et al (1997) put it: ‘The demands of support and those of mobility are always in conflict, and achieving balance between them requires good control mechanisms’. The relative flexibility and support offered by discs, ligaments and muscles are, therefore, key elements in allowing efficient delivery of the various functions that the spine demands.
Braggins (2000) asserts that the two seemingly opposing functions of the vertebral column, rigidity and mobility, have resulted in the development of tough bones that offer strength as well as protection for the soft tissues within and around them, while at the same time there are many small jointed bones that offer flexibility.
In the properly positioned spinal column, the vertebral bodies and the intervertebral discs carry most of the load of the structures above them (and anything being carried). When healthy, the discs themselves are sufficiently flexible to allow flexion, extension, sideflexion, translation (glide) and varying degrees of rotation. The ability of the spine to absorb mechanical stress, therefore, depends to a large extent on the integrity of the spinal discs as well as on the spinal curves. (See Volume 1, Chapter 11 and Chapter 14 for details regarding the cervical and thoracic spine, respectively.)
As Bogduk (2005) explains:
The term ‘zygapophysial’* is derived from the Greek words apophysis, meaning outgrowth, and zygos, meaning yoke or bridge. The term …. therefore means a ‘bridging outgrowth’, and refers to any articular process…other names that are used for the zygapophysial joints are ‘apophysial’ joints and ‘facet’ joints… ‘Facet’ joint is a lazy and deplorable term.
Flexible stability
• Stability clearly derives from a balanced degree of muscular tone in agonists and antagonists, rather than an imbalance such as is evident in lower crossed syndrome patterns where hypertonic extensors commonly overwhelm abdominal flexors (Janda 1996) (see Volume 1, Fig. 5.2).
• Flexibility relates to balanced tone as well as to healthy muscular, ligamentous and joint status and function (optimal strength, elasticity, etc.).
The lumbar spine, in particular, requires maximum stability and flexibility if back pain and dysfunction are to be avoided. As Liebenson (2000a) points out:
Identification of imbalances: essential first step
Successful therapeutic and rehabilitation approaches which meet these requirements for the recovery from musculoskeletal distress demand initial identification of underlying dysfunction, whether this involves hyper- or hypotonicity, hyper- or hypomobility, shortness, weakness, the presence of fibrosis, local myofascial trigger points and/or other evidence of chronic compensation, or indeed decompensation, where adaptive mechanisms break down and pathology ensues. Therapeutic attention needs to focus on the current dysfunctional pattern (which may have emerged as a result of overuse, misuse, disuse or abuse of already compromised structures) as well as on the underlying predisposing features. These issues are described in greater detail in Volume 1, Chapter 4.
Stress factors and homeostasis
It is worth emphasizing that repetitive minor stress has a cumulative effect equivalent to that of a single major stress event. Liebenson (2000a) informs us:
McGill (1998) confirms that low back injury is usually the result ‘of a history of excessive loading which gradually, but progressively, reduces the tissue failure tolerance’. These views are amplified by those of Paris (1997), as discussed later in this chapter.
In 1974 Selye discussed the ways in which multiple minor stress factors impact on the organism. As Shealy (1984) has explained:
Selye has emphasized that any systemic stress elicits an essentially generalized reaction…in addition to any specific damage each stressor might cause. During the stage of resistance (adaptation) a given stressor may trigger less of an alarm; however, Selye insists that adaptation to one [stress] agent is acquired at the expense of resistance to other agents. That is, as one accommodates to a given stressor, other stressors may require lower thresholds for eliciting an alarm reaction. Of considerable importance is Selye’s observation that concomitant exposure to several stressors elicits an alarm reaction at stress levels which individually are sub-threshold. (our italics) (Fig. 10.11).
For example, Lewit (1985) has shown that when the thoracolumbar junction region is painfully restricted, an array of local musculature might be shown to be involved, frequently including quadratus lumborum, the erector spinae, psoas and even tensor fascia latae. Therapeutic interventions and self-care that begin to normalize dysfunction in those most implicated (based on assessment and palpation findings) can often produce a satisfactory resolution without all the structures needing to be treated. As the process evolves, self-normalization commonly takes over, once key restrictions, weaknesses, shortnesses, etc. are addressed. In other words, once a part of the ‘stress load’ has been eased, or functionality restored, homeostatic mechanisms are usually capable of restoring normal function without everything that is demonstrably dysfunctional having to be treated. This theme of homeostatic self-regulation is discussed at length in Volume 1, Chapter 4 and is reviewed in Chapter 1 of this volume.
Examples of these interactions, and greater discussion of the mechanisms involved, are to be found in Volume 1. The following brief quote from Volume 1, Chapter 9, offers a sense of these interactions and how modification of one can influence the others and the overall status of the individual.
• Hyperventilation leads to alkalosis, alters neural reporting (initially hyper and then hypo), creates feelings of anxiety and apprehension, and directly impacts on the thoracic and cervical regions’ structural components, both muscles and joints. (Gilbert 1998).
• Altered chemistry (hypoglycemia, alkalosis, etc.) affects mood directly while altered mood (depression, anxiety) changes blood chemistry, as well as altered muscle tone and, by implication, trigger point evolution (Pongratz et al 2004, Brostoff 1992).
• Altered structure (posture, for example) modifies function (breathing, for example) and therefore impacts on chemistry (e.g. O2: CO2 balance, circulatory efficiency and delivery of nutrients, etc.) which impacts on mood (Gilbert 1998).
Within these categories – biochemical, biomechanical and psychosocial – are to be found most major influences on health, with ‘subdivisions’ (such as ischemia, postural imbalance, trigger point evolution, neural entrapments and compressions, nutritional and emotional factors) being of particular interest in NMT (Chaitow & DeLany 2008).
The contextual environment
The lumbar spine does not operate in a vacuum.
• It articulates at the thoracolumbar junction with its superstructure, the thoracic spine, and all that this indirectly attaches to, as well as with the pelvis, at the sacrum.
• It links directly to the upper extremities via latissimus dorsi and to the lower extremities via the psoas muscles
• It is intimately involved in respiratory function via the merging of both psoas and quadratus lumborum with the diaphragm, which also attaches to the lumbar spine.
• It also copes with gravitational demands, as well as a wide range of movement requirements involving flexion, extension, sideflexion, rotation, torsion, shearing forces, compression and elongation.
The unified nature of spinal stabilization (in particular) and spinal function (in general) is discussed by Tunnell (2000), who offers a useful summary of the background to spinal function and dysfunction.
The problem of spinal stabilization is unique. The term ‘axial organ’ has been coined to refer to the spine and highlights the fact that the spine, while composed of many segments, has a unique function as a distinct and unified organ within the motor system. It functions as the structural axis, or core, of the motor system, around which the peripheral trunk and extremities are organized. However, the spine can only function effectively in this capacity with adequate neuromuscular activity and coordination (Gardner-Morse et al 1995). This requires perception/proprioception, planning, timing, coordination, speed, endurance and strength.
Research has demonstrated the feed-forward spinal stabilization response that occurs before intentional movement begins (Cresswell 1994). The CNS must choose a postural set for each activity, whether static or dynamic. Thus effective stabilization of the spine consists firstly of stabilization of the individual spinal segments into a spinal posture which is both safe for the spine, and biomechanically consistent with the task at hand, and secondly of activity of the more peripheral trunk muscles which transfer loads from the trunk to the pelvis and minimizes the loads experienced by the spinal segments (Bergmark 1989).
Gender issues and posture
The complexity of postural influences is evident in a study by Roberts & Arefi-Afshar (2007). They sought to establish whether proprioceptive feedback from body postures operate differently for women and men. Participants received feedback regarding their posture, and results showed that when men received ‘success feedback’ regarding standing upright as opposed to slumping, this enhanced their performance. In contrast, after adopting an upright posture, women went on, after ‘success feedback’, to perform more poorly. The reasons for this disparity in responses remain unclear.
Soft tissue spinal support
The lumbar spine transfers the weight of the upper body to the pelvis and the lower extremities and also provides mobility for the trunk and protection to central neural structures. The stability of the lumbosacral spine depends on a variety of soft tissue supports as well as its own intrinsic architecture. Willard (in Vleeming et al 2007) provides an insight to the cohesive nature of the soft tissue support.
Coordination
Within this integrated musculoligamentous corset, incorporating both spinal and abdominal structures, stability is more probable if a coordinated relationship exists between agonists and synergists. Low back pain has been shown to be more likely and more severe where inco-ordination exists; for example, overactivity in antagonist back muscles during the swing phase of the gait cycle (see Chapter 3) (Arendt-Nielson 1984), as well as delayed activation of transversus abdominis (for spinal stabilization) during arm movement (Hodges & Richardson 1999). Malcoordination of this sort leads to an unstable situation where injury can more easily occur.
Other forms of coordination may involve co-contraction of antagonist muscles as a stabilizing feature. Cholewicki et al (1997) have shown that lumbar stability was enhanced by the coactivation of agonists and antagonists, but that increased levels of such behavior might be an indication that the passive stabilizing systems of the lumbar spine were less than optimal.
Brief moments of co-contraction are, however, seen as being vital in maintaining safe joint stabilization when unexpected loading occurs. One of the most important muscles responsible for creating stability in the lumbar spine when it is in a neutral range (i.e. not end range) is transversus abdominis (Cholewicki et al 1997). Indeed, studies that employed EMG demonstrated that transversus abdominis is the first muscle recruited when a sudden, alarm perturbation requiring stabilization occurs (Cresswell 1994).
Liebenson (2000d) elaborates to the contrary: ‘While the abdominal muscles receive much of the attention for their protective function in the low back, it is the extensors that are perhaps of even more importance. Decreased trunk extensor endurance has been shown to correlate with low back trouble’. See ‘Endurance factors’ on p. 226 and also Box 10.2, which describes aspects of rehabilitation for these vital supporting structures.
Box 10.2 Core stabilization assessment and exercises
There is an ongoing debate among experts as to the relative importance in back stability of the abdominal musculature as against the trunk extensors. In truth, the debate matters little in the broad context, since the functional integrity of both groups is essential for normal healthy spinal function. Indeed, there is much evidence, as explained earlier in this chapter, that co-contraction of opposing musculature in the spinal region is a common event during normal activities, accentuating the need for good tone and status in both anterior and posterior muscular groups (Cholewicki et al 1997, Liebenson 2000b, d). A variety of exercises have been developed to achieve core stability involving the corset of muscles which surround, stabilize and, to an extent, move the lumbar spine.
Richardson & Jull (1995) have described a ‘coordination’ test that assists in evaluating the patient’s ability to maintain the lumbar spine in a steady state during different degrees of loading. Norris (2000b) describes this procedure of assessment of spinal stability.
• The patient adopts a supine hook-lying position, with a pressure (bio)feedback pad (inflatable cushion attached to pressure gauge, similar to the unit used to test blood pressure) under the lumbar spine.
• The inflated pad registers the degree of pressure being applied by the lumbar spine toward the floor. The objective is to maintain the pressure throughout the performance of various degrees of activity (see below).
• First the patient is asked to hollow the back, bringing the umbilicus toward the spine/floor, so initiating co-contraction of transverse abdominis and multifidus (Fig. 10.13) and to maintain this position as increasing degrees of load are applied by gradually straightening one leg by sliding the heel along the floor. This causes the hip flexors to work eccentrically and, if this overrides the stability of the pelvis, it will tilt. Therefore, if there is a change (reduction) in pressure on the gauge or if a pelvic tilting/increased lumbar lordosis is observed or palpated before the leg is fully extended, this suggests deep abdominal muscular insufficiency involving transversus abdominis and internal obliques.
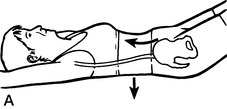
Figure 10.13 A ‘Neutral spine’ coordination test
reproduced with permission from Journal of Bodywork and Movement Therapies 2000;4(2):110)
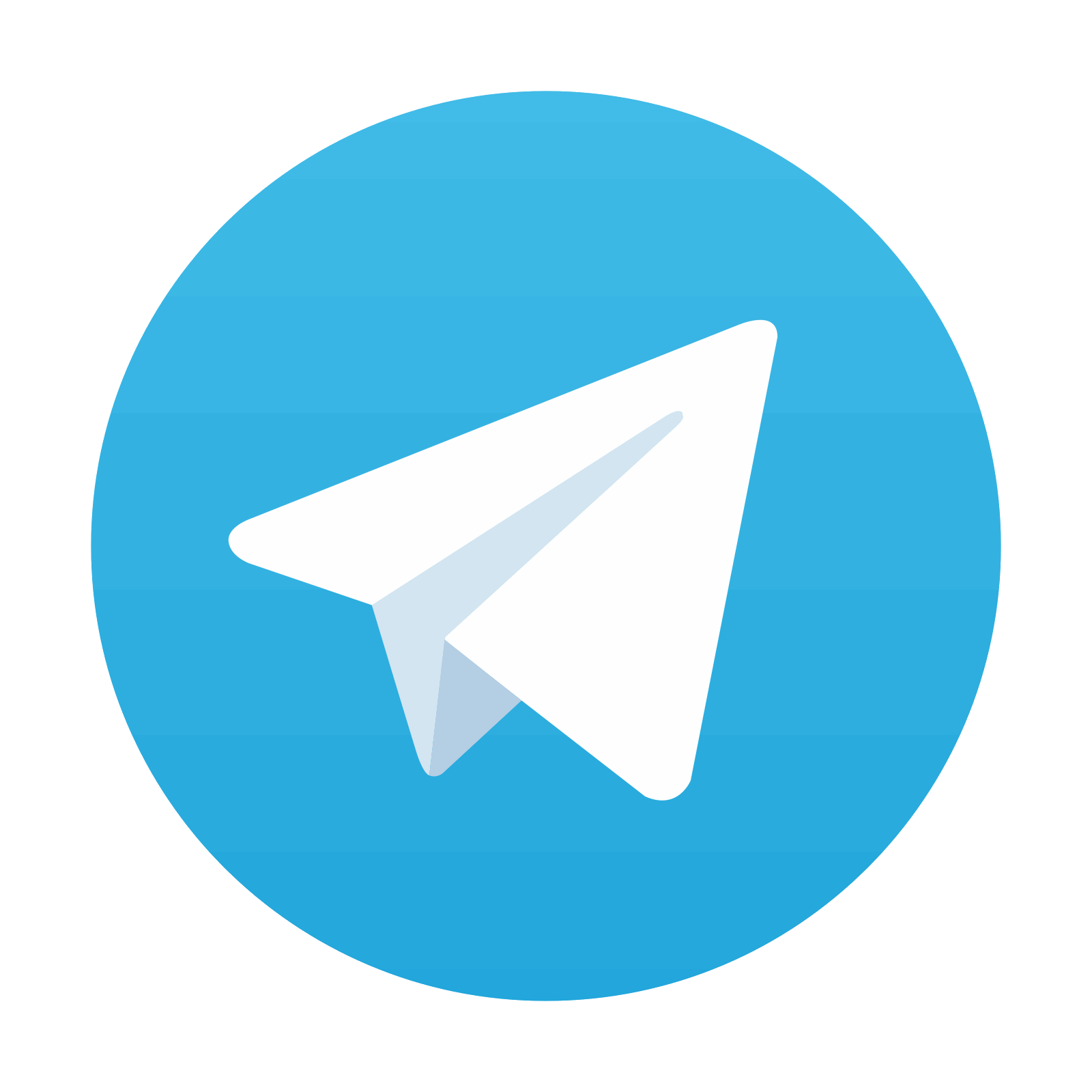
Stay updated, free articles. Join our Telegram channel

Full access? Get Clinical Tree
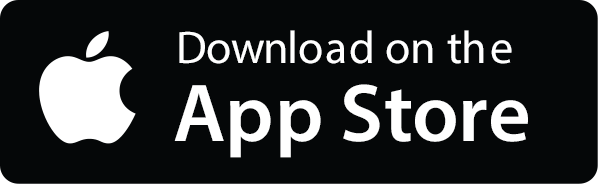
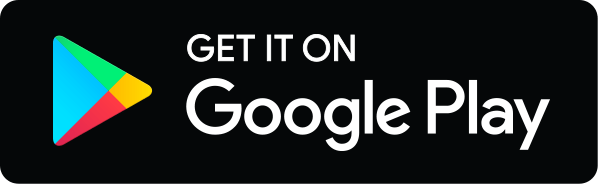
