The Modulatory Functions of the Brain Stem
Ascending Monoaminergic and Cholinergic Projections from the Brain Stem Maintain Arousal
Monoaminergic and Cholinergic Neurons Share Many Properties and Functions
Many Monoaminergic and Cholinergic Neurons Are Linked to the Sleep-Wake Cycle
Monoamines Regulate Many Brain Functions Other Than Arousal
Cognitive Performance Is Optimized by Ascending Projections from Monoaminergic Neurons
Monoamines Are Involved in Autonomic Regulation and Breathing
Pain and Anti-nociceptive Pathways Are Modulated by Monoamines
Postscript: Evaluation of the Comatose Patient
AS WE LEARNED IN THE PREVIOUS CHAPTER, the brain stem can respond independently to the environment with stereotypic actions. We have also seen, in discussing the sensory and motor systems, that the brain stem is the conduit for all ascending and descending pathways between the forebrain, spinal cord, and peripheral nervous system. In this chapter we examine still a third role of the brain stem, as the modulatory center that orchestrates the activity of the rest of the central nervous system, ensuring that its activity is optimized.
This modulatory function is mediated by several small groups of neurons in the brain stem. These neurons project widely, using as neurotransmitters acetylcholine and the monoamines (norepinephrine, epinephrine, serotonin, dopamine, and histamine). Many of the monoaminergic groups modify pain and help regulate the autonomic nervous system to maintain internal homeostasis. Some are essential for controlling the level of behavioral arousal; and together they influence attention, mood, and memory. Because they are involved in the pathophysiology of many human diseases and are targets of many commonly used drugs, these monoaminergic and cholinergic neurons are also important for clinical care.
Behaviors we regard as uniquely human, such as memory, language, and compassion, depend heavily on modulation of forebrain function by the ascending cholinergic and monoaminergic systems. This dependence is seen clinically in the links between Alzheimer’s disease and the acetylcholine system, schizophrenia and the dopaminergic system, and alleviation of depression with drugs that affect serotonergic and noradrenergic synapses. Thus, although the brain stem is phylogenetically primitive, the modulatory systems that project from this region enable and modulate many of the higher-order behaviors that we regard as most human.
Ascending Monoaminergic and Cholinergic Projections from the Brain Stem Maintain Arousal
Some neurons in the brain stem that project to the forebrain control wakefulness and sleep. In the mid-1930s Frederic Bremer found that transection of the cat’s brain stem at the midbrain level produced a continuous sleep-like state, whereas transections that separated the medulla from the spinal cord did not (Figure 46–1). These experiments demonstrate that the portion of the brain stem from the midbrain to the medulla keeps the forebrain awake.
Figure 46-1 Ascending projections from the rostral brain stem are necessary for maintaining arousal of the cortex.
A. Transection of the brain stem in the lower medulla of a cat causes low-amplitude, fast electroencephalogram (EEG) activity characteristic of wakefulness. Animals appear awake and respond to sensory input from cranial nerves, but the muscles innervated by the spinal cord, including the diaphragm (which is required for breathing), are paralyzed.
B. Transection of the brain stem of a cat between the superior and inferior colliculi in the midbrain causes a sleep-like state from which the animal cannot be aroused. The EEG shows periods of slow delta waves (1–3 Hz) alternating with spindles (bursts of 8–11 Hz activity that wax and wane over a few seconds and are generated by the intact thalamus).
C. In humans injury to the rostral pons, the midbrain, or the thalamus and hypothalamus (shaded areas) causes impairment of consciousness. As shown here, the EEG of a drowsy patient consists of theta activity (4–7 Hz waves) and some larger delta waves. As the patient becomes less arousable, the amount of delta activity increases. Spindles similar to the cat appear as humans enter slow wave sleep but may not occur in coma if the thalamus is impaired.
Consistent with this view, experiments in 1949 by Guiseppi Moruzzi and Horace Magoun found that damage to the brain stem reticular formation in cats led to loss of wakefulness. Conversely, stimulating the reticular formation immediately converted the electroencephalogram (EEG) of a sleeping cat to a waking EEG. Transection of the brain stem at the level of the midpons or lower did not cause loss of wakefulness, indicating that the critical structures that need to be connected to the forebrain for wakefulness are located in the rostral pons and caudal midbrain.
Moruzzi and Magoun proposed that this part of the brain stem provides a general activation energy for the entire brain and therefore called it the reticular activating system. Today this system is more accurately called the ascending arousal system, because we know it is not confined to the reticular formation. As we shall learn in Chapter 51, sleep and waking are regulated by interactions between this ascending arousal system and sleep-promoting regions in other parts of the brain. Damage to the ascending arousal system or its projections in the thalamus and hypothalamus leads to coma (Figure 46–1C, and see the postscript, “Evaluation of the Comatose Patient,” at the end of this chapter).
The ascending arousal system was originally viewed as a heterogeneous collection of neurons distributed diffusely throughout the brain stem reticular formation, which had widespread projections. This view changed radically in the early 1960s when Nils-Åke Hillarp and his students in Sweden used histofluorescence to discover that the neurons in this brain stem system fall into chemically distinct groups based on their monoamine neurotransmitter content. In a brilliant series of histochemical experiments they defined the location and projections of these neurons based on differences in the fluorescence signals produced by the different monoamines: norepinephrine, dopamine, and serotonin (Figure 46–2).
Figure 46-2 Locations and projections of monoaminergic and cholinergic neurons in the rat brain. (3V, third ventricle; AC, anterior commissure; AP, area postrema; AQ, Sylvian aqueduct; ARC, arcuate nucleus; BM, nucleus basalis of Meynert; BP, brachium pontis; CD, caudate; CP, cerebral peduncle; DBh, horizontal limb of the diagonal band; DR, dorsal raphe; FX, fornix; IC, inferior colliculus; LC, locus ceruleus; LDT, laterodorsal tegmental nucleus; MCP, middle cerebellar peduncle; MGN, medial geniculate nucleus; MR, median raphe; MS, medial septum; MTT, mammillothalamic tract; NTS, nucleus tractus solitarius; OC, optic chiasm; PPT, pedunculopontine tegmental nucleus; PUT, putamen; Pyr, pyramidal tract; RM, raphe magnus; SC, superior colliculus; SCP, superior cerebellar peduncle; SN, substantia nigra; STN, spinal trigeminal nucleus; TMN, tuberomammillary nucleus; VTA, ventral tegmental area.)
A. Noradrenergic neurons (A groups) and adrenergic neurons (C groups) are located in the medulla and pons (shaded). The A2 and C2 groups in the dorsal medulla are part of the nucleus of the solitary tract. The A1 and C1 groups in the ventral medulla are located near the nucleus ambiguus. Both groups project to the hypothalamus; some C1 neurons project to sympathetic preganglionic neurons in the spinal cord and control cardiovascular and endocrine functions. The A5, A6 (locus ceruleus), and A7 cell groups in the pons project to the spinal cord and modulate autonomic reflexes and pain sensation. The locus ceruleus also projects rostrally to the forebrain and plays an important role in arousal and attention.
B. All histaminergic neurons are located in the posterior lateral hypothalamus, mostly within the tuberomammillary nucleus. These neurons project to virtually every part of the neuraxis and play a major role in arousal.
C. Serotonergic neurons (B groups) are found within the medulla, pons, and midbrain, mostly near the midline in the raphe nuclei. Those within the medulla (the B1–B4 groups corresponding to the raphe magnus, raphe obscurus, and raphe pallidus) project throughout the medulla and spinal cord and modulate afferent pain signals, thermoregulation, cardiovascular control, and breathing. Those within the pons and midbrain (the B5–B9 groups in the raphe pontis, median raphe, and dorsal raphe) project throughout the forebrain and contribute to arousal, mood, and cognition.
D. Cholinergic neurons (Ch groups) are located in the pons, midbrain, and basal forebrain. Those in the pons and midbrain (mesopontine groups) are divided into a ventrolateral cluster (pedunculopontine nucleus) and the dorsomedial cluster (laterodorsal tegmental nucleus). The mesopontine cholinergic neurons project to the brain stem reticular formation and the thalamus. Those in the basal forebrain are found in the medial septum, the nuclei of the vertical and horizontal limbs of the diagonal band, and the nucleus basalis of Meynert. These neurons project throughout the cerebral cortex, hippocampus, and amygdala. Both groups play an important role in arousal, and the basal forebrain groups are also involved in attention.
E. Dopaminergic neurons are located in the midbrain and hypothalamus. The dopaminergic cell groups were originally included with the noradrenergic lettering system and are still labeled as A groups. The A9 cell group is located in the substantia nigra pars compacta. The A8 group is in a region of the midbrain tegmentum, dorsally adjacent to the substantia nigra. These two groups of neurons project to the striatum and play an important role in initiation of movement. The A10 group is located in the ventral tegmental area just medial to the substantia nigra. These cells project to the frontal and temporal cortex and limbic structures of the basal forebrain and play a role in emotion and memory. The A11 and A13 cell groups in the zona incerta of the hypothalamus project to the lower brain stem and spinal cord and regulate sympathetic preganglionic neurons. The A12, A14, and A15 cell groups are components of the neuroendocrine system. Some of them inhibit release of prolactin into the hypophysial portal circulation, and others control gonadotrophin secretion. Dopaminergic neurons are also found in the olfactory bulb (A16) and the retina (A17).
These findings were later expanded in studies that used antibodies against the synthetic enzymes for the monoamine neurotransmitters (which also allowed visualization of histamine and epinephrine) and acetylcholine. The connectivity of these cell groups is remarkable. Whereas most nuclei in the central nervous system project to specific regions or to a limited set of nuclei, the monoaminergic and cholinergic neurons of the ascending arousal system have widespread projections, some to virtually every part of the central nervous system.
A major part of the ascending arousal system consists of monoaminergic and cholinergic neurons in the brain stem. These neurons are found primarily in four regions (Figure 46–3A):
Figure 46-3 Major cell groups in the ascending arousal system in the rat brain.
A. Neurons using the neurotransmitters norepinephrine, serotonin, dopamine, histamine, and acetylcholine have widespread forebrain projections, but there are important differences in the distributions. They all contribute to arousal, but effects on other brain functions vary. Other groups of neurons that play an important role in arousal include the glutamatergic parabrachial nucleus and hypothalamic neurons that secrete orexin and melanin-concentrating hormone.
B. Stimulation of noradrenergic neurons in the locus ceruleus (LC) induces arousal. The cholinergic agonist bethanechol was microinjected directly into the locus ceruleus of a lightly anesthetized (halothane) rat. Arousal, which shows up in the EEG as low voltage, fast activity (arrow), coincides in time with the bethanechol-induced increase in firing of the locus ceruleus neurons. (EEG, electroencephalogram; ILT, intralaminar thalamic nuclei; RT, reticular nucleus of the thalamus.) (Reproduced, with permission, from Berridge and Foote 1991.)
1. The locus ceruleus, which contains noradrenergic neurons
2. The dorsal and median raphe nuclei, which contain primarily serotonergic, but also some dopaminergic neurons
3. The pedunculopontine and laterodorsal tegmental nuclei, which contain cholinergic neurons
4. The tuberomammillary nucleus, which contains histaminergic neurons
The monoaminergic pathways from the locus ceruleus, and dorsal and median raphe nuclei, are thought to regulate sleep and waking. Other neurons in the parabrachial nucleus in the rostral pons and caudal midbrain, which largely use glutamate as a neurotransmitter, are also thought to contribute to the regulation of wakefulness and sleep. In addition to these reticular neurons, neurons in the lateral hypothalamus that contain the peptide neurotransmitters orexin and melanin-concentrating hormone are important for sleep and waking. Finally, cholinergic and GABA-ergic (γ-aminobutyric acid) neurons in the basal forebrain contribute to arousal through diffuse cortical projections.
Stimulation of noradrenergic neurons in the locus ceruleus or histaminergic cells in the tuberomammillary nucleus causes increases in EEG arousal (Figure 46–3B), indicating that these systems play an important role in cortical and behavioral arousal. However, lesions restricted to one biogenic amine cell group do not cause profound loss of wakefulness, suggesting that the various cell groups probably have overlapping and at least partly redundant roles in sleep/wake regulation. As we will see, the monoaminergic pathways alter specific cellular properties of postsynaptic neurons in the thalamus and cerebral cortex, enhancing alertness and interaction with environmental stimuli.
At the junction of the midbrain and diencephalon the projections from the ascending arousal system split into two major branches, dorsal and ventral. The dorsal branch terminates in the thalamus. The ventral branch travels through the lateral hypothalamic area and is joined by ascending projections from the hypothalamus and the basal forebrain before terminating throughout the cerebral cortex. Lesions that disrupt either of these two branches impair consciousness.
Monoaminergic and Cholinergic Neurons Share Many Properties and Functions
Monoamines are biochemical compounds with an aromatic ring that are synthesized from aromatic amino acids. They include the catecholamines (epinephrine, norepinephrine, and dopamine), serotonin, and histamine. Neurons that use monamines as neurotransmitters share many cellular properties. For example, most continue to fire spontaneous action potentials in a highly regular pattern when isolated from their synaptic inputs in brain slice preparations. Their action potentials typically are followed by a slow membrane depolarization that leads to the next spike (Figure 46–4A).
Figure 46-4 Monoaminergic neurons have common properties.
A. The baseline electrophysiological properties of all monoaminergic neurons are similar. The slow, regular firing pattern of a noradrenergic neuron in the locus ceruleus is shown at the upper left. Serotonergic and histaminergic neurons are similar. The upper right shows the average impulse activity of noradrenergic cells in the locus ceruleus in a behaving rat shown during different stages of sleep and waking. Histaminergic and serotonergic neurons exhibit a similar pattern of activity over the sleep-wake cycle. The bottom left shows the average activity of presumptive cholinergic neurons in the basal forebrain. Note decreased activity from waking to slow sleep (NR1 and NR2) but increased activity during REM sleep. The lower right shows that firing of neurons in the locus ceruleus follows a circadian rhythm. Histograms show the distributions of firing rates of the neurons during dark and light periods of the circadian cycle. Lesions of the dorsomedial nucleus of the hypothalamus eliminate the difference in locus ceruleus firing rates during the dark and light periods. (REM, rapid eye movement sleep; SWS, slow-wave sleep; W, wakefulness; AW, active wakefulness; QW, quiet wakefulness; NR1, lighter stages of non-REM sleep; NR2, deeper stages of non-REM sleep.) (Upper right reproduced, with permission, from Aston-Jones and Bloom 1981; lower left modified, with permission, from Szymusiak et al. 2000; lower right reproduced, with permission, from Aston-Jones et al. 2001.)
B. At conventional synapses the presynaptic terminal is closely aligned with the postsynaptic membrane (left). In contrast, the en passant terminal diffusely releases a transmitter that can affect several synapses within the immediate area (right). Acetylcholine and each of the monoamines can be released using this so-called paracrine mechanism.
The spontaneous regular firing pattern of monoaminergic neurons is regulated by intrinsic pacemaker currents. Tonic firing in vivo may be important for ensuring the continuous delivery of monoamines to targets. For example, the basal ganglia depend on continuous exposure to dopamine from the neurons of the substantia nigra to facilitate motor responses.
The properties of monoaminergic neurons are suited to their unique and widespread modulatory roles in brain function. Indeed, some axon terminals of monoaminergic cells do not even form conventional synaptic connections, instead releasing neurotransmitter diffusely to many targets at once (Figure 46–4B). Most monoamine neurotransmission occurs by means of metabotropic synaptic actions through G protein-coupled receptors. Many monoaminergic neurons co-release neuropeptides, which have slow effects through other G protein-coupled receptors. Thus, although some monoamine responses are mediated through fast synaptic mechanisms (see Chapter 10), many involve slower metabotropic and neuromodulatory actions as well (see Chapter 11).
Some cholinergic neurons in the brain stem share certain of these properties. For example, cholinergic neurons in the pedunculopontine and laterodorsal tegmental nuclei have widespread projections, providing the bulk of the cholinergic input to the thalamus, particularly to its relay and reticular nuclei. Many effects of cholinergic neurons also are mediated by G protein-coupled muscarinic receptors.
Many Monoaminergic and Cholinergic Neurons Are Linked to the Sleep-Wake Cycle
Most central neurons fire maximally during waking, decrease firing in a phase of sleep called slow-wave sleep, and then increase firing again during a phase of sleep called rapid eye movement (REM) sleep, the phase of the sleep cycle in which dreams are particularly likely to occur (see Chapter 51). Motor neurons are an exception; they have their lowest firing frequency during REM sleep because they are actively inhibited to prevent the acting out of dreams.
Noradrenergic, serotonergic, and histaminergic neurons are unlike most central neurons and more like motor neurons. They are maximally active during waking, their firing rates progressively decrease with increasing depth of non-REM sleep, and they stop almost completely during REM sleep (Figure 46–4A). Dopaminergic neurons within the dorsal raphe region also are most active during waking, but it has not yet been possible to distinguish their activity patterns during non-REM and REM sleep from those of the serotonergic neurons with which they intermingle.
In contrast to monoaminergic neurons, the firing rates of cholinergic neurons in the pedunculopontine and laterodorsal tegmental nuclei decrease during non-REM sleep but increase during REM sleep (Figure 46–4A). These and other properties indicate that the role of acetylcholine in controlling arousal is different from that of the monoamines.
The sleep-wake cycle has a strong circadian rhythm. The pacemaker for this rhythm is the suprachiasmatic nucleus in the hypothalamus (see Chapter 51). The suprachiasmatic nucleus regulates the circadian rhythm of sleep and waking primarily through connections with other hypothalamic areas, such as the dorsomedial nucleus. The dorsomedial nucleus in turn contacts both sleep- and wake-promoting cell groups in the hypothalamus and brain stem. As a result, the ascending arousal system entrains to the circadian rhythm (Figure 46–4A).
Monoaminergic and Cholinergic Neurons Maintain Arousal by Modulating Neurons in the Thalamus and Cortex
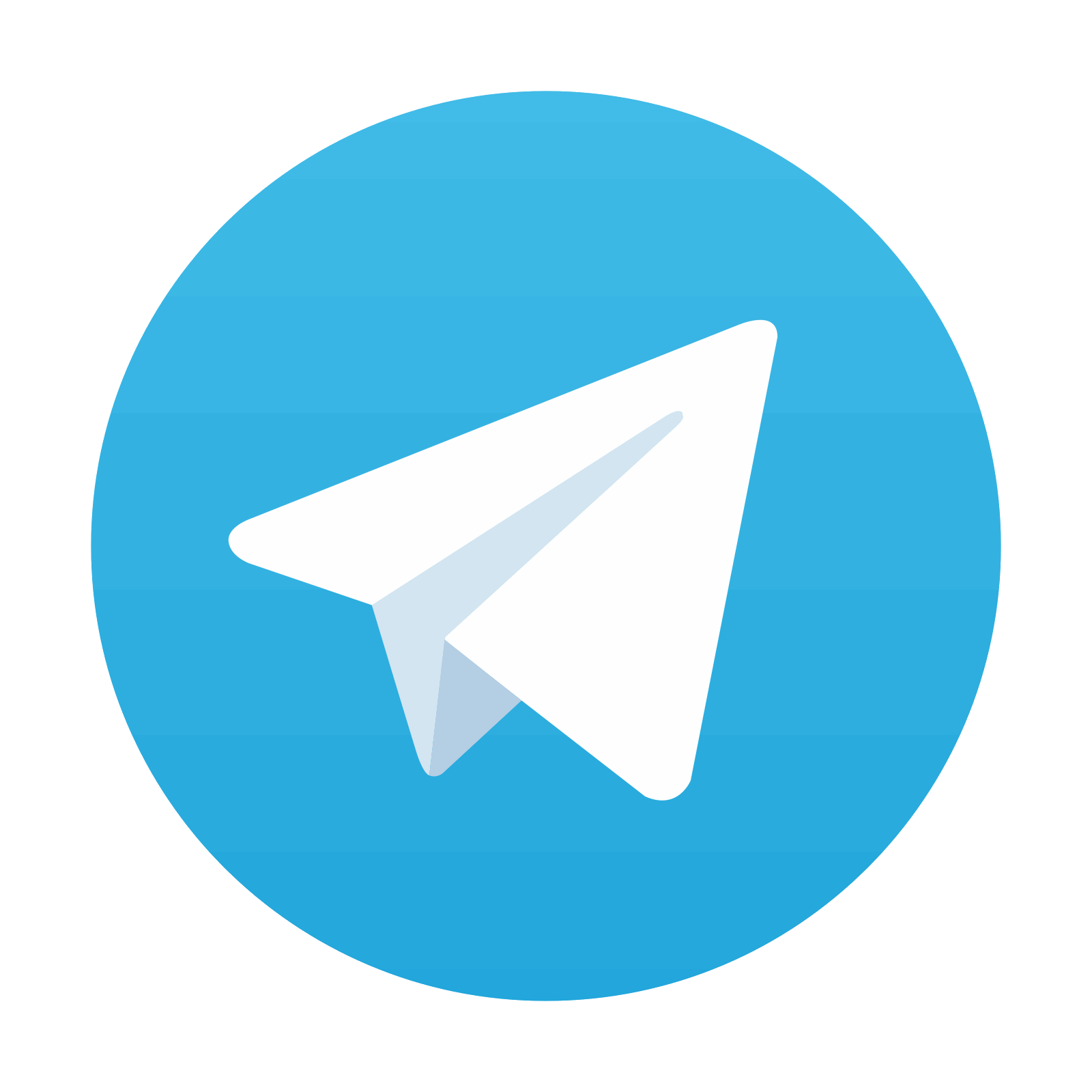
Stay updated, free articles. Join our Telegram channel

Full access? Get Clinical Tree
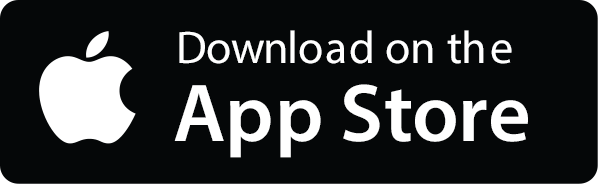
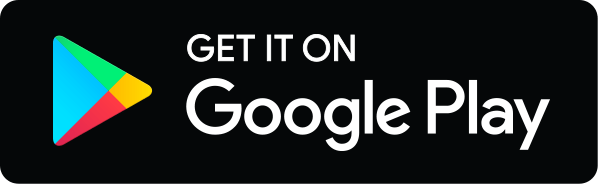