FIGURE 23.1 The motor unit. An alpha motor neuron in the anterior horn gives rise to an axon, which branches and arborizes in the periphery to innervate scattered muscle fibers within a muscle fascicle.
THE MOTOR UNIT
Alpha motor neurons reside in the anterior horn of the spinal cord and the brainstem motor nuclei. The axon traverses the anterior root and the peripheral nerve en route to the muscle. The peripheral nerve enters the muscle at the motor point and divides into intramuscular branches. These arborize within a muscle fascicle and terminate as fine twigs, which end as axon boutons. Terminal boutons abut the motor end plates of individual muscle fibers across a synaptic cleft, forming NMJs. Each muscle fiber has only a single end plate.
Terminal axonal twigs ramify in the muscle and innervate widely dispersed muscle fibers. A motor unit may have anywhere from a handful of muscle fibers to more than a thousand. The innervation ratio refers to the number of muscle fibers in a motor unit. A low innervation ratio means few muscle fibers are innervated by a single axon and is characteristic of muscles under precise and finely graded voluntary control, such as extraocular or laryngeal muscles. A muscle performing a gross motor movement may have several hundred muscle fibers per motor unit. The gastrocnemius has about 2,000. The innervation ratios for the brachial biceps, tibialis anterior, and deltoid are 209, 329, and 239 fibers, respectively. The anatomical scatter of fibers belonging to the same motor unit may vary from muscle to muscle. Electrophysiologic motor unit counting techniques estimate that an intrinsic hand muscle has about 100 motor units. Beyond the age of 60 years, there is a decline in the number of functioning motor units.
Motor units vary by size within a muscle. Smaller motoneurons have smaller motor unit territories. To produce a smoothly graded muscle contraction, motor units are recruited, more or less, in order of increasing size. Small motoneurons are first recruited, and increasing force of contraction calls forth activity from increasingly larger motoneurons: the size principle.
Motor units are classified as type 1 or type 2. Muscles were broadly divided into red muscle and white muscle, dark meat and light meat, long before the basis for the difference was understood. The red or dark color is now known to result from the presence of the instruments for oxidative metabolism: myoglobin, mitochondria, and a vascular network for delivery of oxygen to the metabolizing muscle cells. Histochemical stains help separate the different types of muscle fibers and often allow the appreciation of structural detail and abnormalities not seen with routine hematoxylin and eosin stains. The myosin ATPase (adenosine triphosphatase) histochemical stain identifies two distinct populations of muscle fibers, referred to as type 1 and type 2, which correspond to type 1 and 2 motor units. Further adjustment of pH allows separation of type 2 muscle fibers into types 2A and 2B (Box 23.1; Figure 23.1). An average muscle contains about 40% type 1 fibers and 60% type 2 fibers. However, this ratio varies with the anatomical location and function of the muscle, and similar muscles may vary among individuals. All fibers in a particular motor unit are of the same type, and there is good correlation between the mechanical properties and other attributes of a motor unit and the histochemical reactions of its muscle fibers. The fiber type mix of a muscle is determined by its innervation and ultimately by its function. In needle electromyography, the summated electrical activity of all the muscle fibers of a motor unit—a motor unit action potential—is recorded by a needle electrode inserted into the muscle.
Type 1 and Type 2 Muscle Fibers
The myosin ATPase (adenosine triphosphatase) stain identifies the two distinct populations of type 1 and type 2 muscle fibers; the differences are summarized in Table 23.1. Cross sections reveal a random admixture of the two fiber types creating a checkerboard pattern. The type 2 fibers stain darkly and the type 1 fibers lightly at pH 9.4; the staining characteristics reverse at pH 4.3. Preincubation at pH 4.6 identifies two kinds of type 2 fibers: type 2A and type 2B. The 2B fibers are the classic fast twitch, fatigue-sensitive glycolytic fibers, while 2A fibers have characteristics intermediate between type 1 and type 2B, with some oxidative capability, slower twitch, and more fatigue resistance than the 2B fibers. Pathologic processes may cause characteristic abnormalities of fiber type distribution or proportion, or produce changes primarily in one particular fiber type.
TABLE 23.1 Attributes and Characteristics of Type 1 and Type 2 Muscle Fibers
ATPase, adenosine triphosphatase.
Type 1 muscle fibers are rich in oxidative enzymes and mitochondria but sparse in glycogen; they are designed for sustained, long-duration contraction under aerobic conditions. Red, or dark, meat is high in type 1 fibers. Type 2 fibers are rich in glycogen and glycolytic enzymes but sparse in oxidative enzymes, mitochondria, and lipid. They are designed for brief, intense bursts of activity under anaerobic conditions. White meat is high in type 2 fibers. The mnemonic “one, slow, red ox” helps recall the essentials: type 1 fibers, slow muscle, red meat, oxidative metabolism.
In another functional and physiologic scheme, motor units are classified into three different types: fast twitch, fatigue sensitive (FF); slow twitch, fatigue resistant (S); and intermediate (FR). Type FF units are fast twitch and fatigue sensitive, type 2B histochemically, rich in glycogen but poor in oxidative enzymes, and designed for brief, phasic activity. Type S units are slow twitch, fatigue resistant, type 1 histochemically, low in glycolytic but high in oxidative enzymes, and designed for sustained, tonic activity. Type FR is intermediate, fast twitch but more fatigue resistant than type FF, type 2A histochemically, high in glycolytic, and intermediate in oxidative enzyme activity.
Myotomes
A myotome consists of all the muscles innervated by a specific nerve root. Most skeletal muscles receive innervation from two or more roots, and there is inherent variability in the myotomal patterns among individuals. Early anatomists reported myotome innervation from detailed dissections, and some errors have been perpetuated through the years. Many different innervation charts are available, and most vary in some details. Some misinformation remains, such as the inclusion of C6-C7 innervation to the thenar muscles, which does not fit clinical reality. The issue has been compounded by observations during intraoperative recordings that indicate contributions from unexpected sources to leg muscles and anomalous innervation so frequent as to be the rule rather than the exception. Liveson and Ma present charts derived from seven different sources, separating “new myotomes” derived from electromyographic data.
Microanatomy of the Peripheral Nerve
Peripheral nerves are composed of myriad axons, ensheathed by myelin of varying thickness and supported by Schwann cells, all imbedded in a matrix of connective tissue. Nerves are divided into discrete internal fascicular compartments by perineurium. The blood nerve barrier is a physiologic partition, created by the perineurium and the endothelium of intrafascicular capillaries. It regulates the nerve microenvironment and acts as a diffusion barrier. The extreme terminal ends of nerve fibers are not protected by perineurium and have no effective blood-nerve barrier, a detail of probable importance in the pathogenesis of some peripheral neuropathies. Within each fascicle, endoneurium separates individual axons and their Schwann cells (Figure 23.2). Fascicles are bound together into nerve trunks by the epineurium, loose areolar connective tissue that also contains blood vessels, lymphatics, and the nervi nervorum. The epineurium also serves an important cushioning role. The interfascicular epineurium lies between fascicles; the epifascicular epineurium circumferentially envelops the entire nerve.
FIGURE 23.2 The normal peripheral nerve is composed of connective tissue and neural tissue components. The nerve fibers may be myelinated or unmyelinated. (Modified from Mackinnon SE, Dellon AL. Surgery of the Peripheral Nerve. New York: Thieme, 1988, with permission.)
Fascicles bifurcate, join with adjacent fascicles, redivide, and recombine to create a complex internal fascicular network (Figure 23.3). Nerves can be classified into monofascicular, oligofascicular, and polyfascicular types. A polyfascicular pattern is common in regions subject to mechanical stress and where there is heavy fiber exchange, such as the brachial plexus. Plexiform fascicular exchange is most prominent proximally, and a constant fascicular pattern is present for only a short distance in proximal regions of a nerve. Fascicles innervating a particular muscle or sensory zone become more discrete and constant in position as they approach the target organ. This complex intraneural topography has important clinical, surgical, and electrophysiologic implications.
FIGURE 23.3 An illustration of the internal topography of the median nerve at different levels. The complexity of the internal fascicular anatomy is apparent. The degree of plexus formation between fascicles decreases in the distal portion of the nerve as the bundles approach their target muscles. (Modified from Mackinnon SE, Dellon AL. Surgery of the Peripheral Nerve. New York: Thieme, 1988, with permission.)
Axons are divided into three major size groups: large myelinated, small myelinated, and unmyelinated. Large myelinated axons have diameters in the 6-to 12-μm range, small myelinated axons are 2 to 6 μm, and unmyelinated axons are 0.2 to 2.0 μm. Small myelinated fibers are about three times more numerous than large myelinated axons. The myelin sheath adds additional thickness. Conduction is most efficient when the ratio of the axon diameter to total fiber diameter is 0.5 to 0.7.
Ultrastructurally, axons contain cytoskeletal elements, neurofilaments, and neurotubules, which are synthesized in the cell body and move slowly down the axon at a rate of 3 mm/day. Neurotubules consist of polymerized dimers of tubulin protein forming longitudinally oriented hollow tubes about 20 nm in diameter and 1 mm long, linked by cross bridges to the neurofilaments. Neurofilaments are smaller organelles that maintain axonal structure. Neurotubules are responsible for fast antegrade and retrograde axonal transport (see Physiology of the Peripheral Nerve, p. 309).
In myelinated axons, a single Schwann cell wraps a single internodal segment in concentric layers of myelin (Figure 23.4). Schmidt-Lanterman incisures are bits of Schwann cell cytoplasm sequestered between layers of myelin. The external plasma membrane of the Schwann cell is continuous with the outermost layer of myelin; the inner membrane of the Schwann cell is immediately adjacent to the outer surface of the axolemma. The external lamina is a condensation of extracellular matrix surrounding the entire external surface of the Schwann cell. The nodes of Ranvier are gaps in the myelin coverage between the territories of adjacent Schwann cells. Internodal length varies with fiber size; it is about 1 mm for large-diameter fibers. For unmyelinated axons, a single Schwann cell, sometimes referred to as a Remak cell, sends out processes to support several adjacent axons, lending to each primarily a cytoplasmic coat and only a minimal investment of myelin. A complex of several unmyelinated axons and their supporting Remak cell is encased by an external lamina.
FIGURE 23.4 Illustration of a single myelinated nerve fiber demonstrates the relationships between the axon, the Schwann cell, and the myelin sheath at different points. (Modified from Mackinnon SE, Dellon AL. Surgery of the Peripheral Nerve. New York: Thieme, 1988, with permission.)
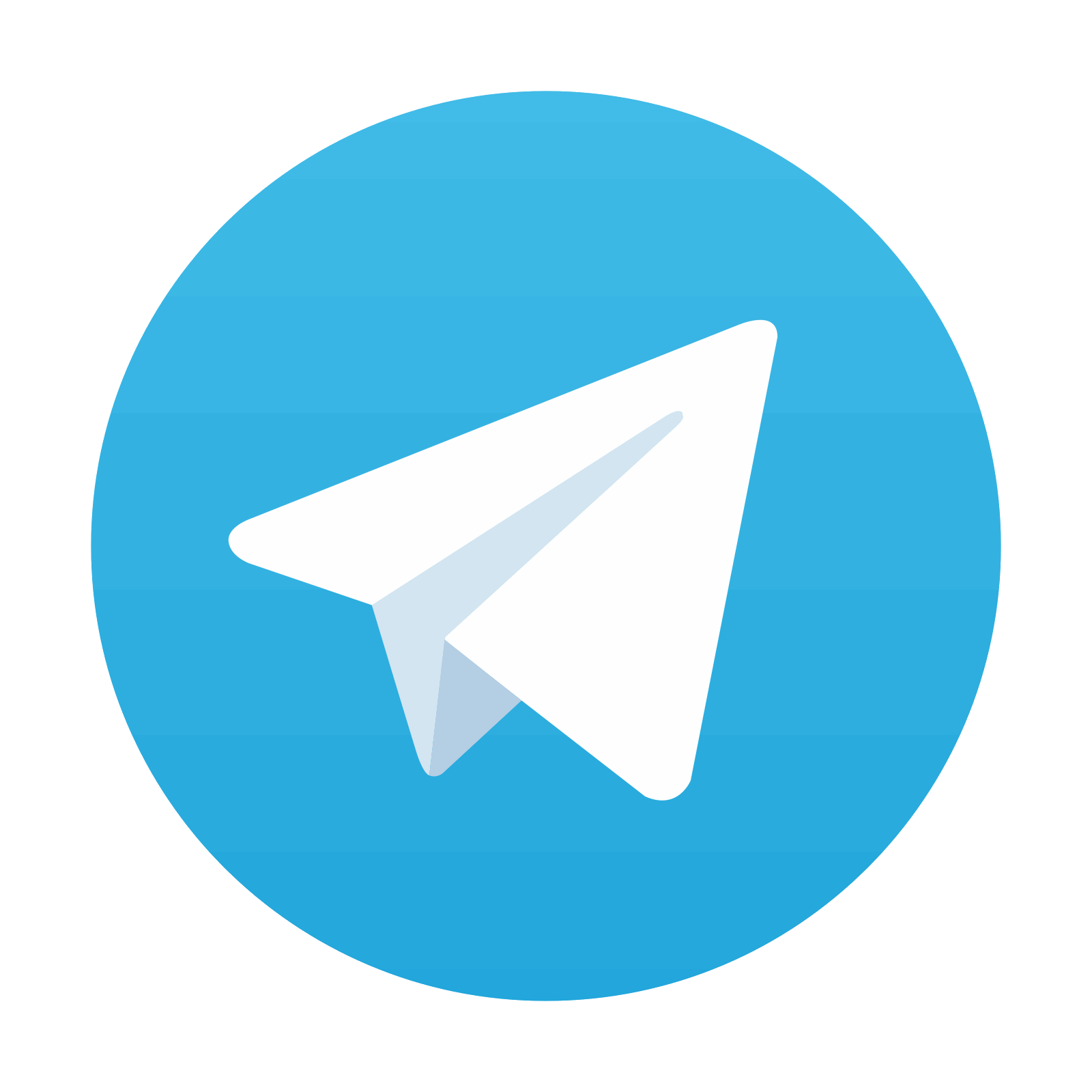