The Somatosensory System: Receptors and Central Pathways
The Primary Sensory Neurons of the Somatosensory System Are Clustered in the Dorsal Root Ganglia
Peripheral Somatosensory Nerve Fibers Conduct Action Potentials at Different Rates
Many Specialized Receptors Are Employed by the Somatosensory System
Visceral Sensations Represent the Status of Various Internal Organs
Somatosensory Information Enters the Central Nervous System Through Cranial and Spinal Nerves
Somatosensory Information Flows from the Spinal Cord to the Thalamus Through Parallel Pathways
The Dorsal Column-Medial Lemniscal System Relays Tactile and Proprioceptive Information
The Spinothalamic System Conveys Noxious, Thermal, and Visceral Information
The Thalamus Has a Number of Specialized Somatosensory Regions
The Ventral Posterior Nucleus Relays Tactile and Proprioceptive Information
Noxious, Thermal, and Visceral Information Is Processed in Several Thalamic Nuclei
WE BEGIN THE STUDY OF THE INDIVIDUAL sensory systems with the somatosensory system (Greek soma, the body), the system in which sensory coding was first studied electrophysiologically. Somatic information is provided by receptors distributed throughout the body. One of the earliest investigators of the bodily senses, Charles Sherrington, noted that the somatosensory system serves three major functions: proprioception, exteroception, and interoception.
Proprioception is the sense of oneself (Latin proprius, one’s own). Receptors in skeletal muscle, joint capsules, and the skin enable us to have conscious awareness of the posture and movements of our own body, particularly the four limbs and the head. Although one can move parts of the body without sensory feedback from proprioceptors, the movements are often clumsy, poorly coordinated, and inadequately adapted to complex tasks, particularly if visual guidance is absent.
Exteroception is the sense of direct interaction with the external world as it impacts on the body. The principal mode of exteroception is the sense of touch, which includes sensations of contact, pressure, stroking, motion, and vibration, and is used to identify objects. Some touch involves an active motor component—stroking, tapping, grasping, or pressing—whereby a part of the body is moved against another surface or organism. The sensory and motor components of touch are intimately connected anatomically in the brain and are important in guiding behavior.
Exteroception also includes the thermal senses of heat and cold. Thermal sensations are important controllers of behavior and homeostatic mechanisms needed to maintain the body temperature near 37°C (98.6°F). Finally, exteroception includes the sense of pain, or nociception, a response to external events that damage or harm the body. Nociception is a prime motivator of actions necessary for survival, such as withdrawal or combat.
The third component of somatic sensation, interoception, is the sense of the function of the major organ systems of the body and its internal state. Although most of the events recorded by receptors in the viscera do not become conscious sensations, the information conveyed by these receptors is crucial for regulating autonomic functions, particularly in the cardiovascular, respiratory, digestive, and renal systems. Interoceptors are primarily chemoreceptors that monitor organ function through such indicators as blood gases and pH.
Abnormal function in major organ systems resulting from disease or trauma can evoke conscious sensations of pain. Much of our knowledge of the neural mechanisms of pain is derived from studies of cutaneous nociceptors because the mechanisms are easier to study in cutaneous nerves than in visceral nerves. Nevertheless, the neural mechanisms underlying visceral pain are similar to those for pain arising from the surface of the body.
This diverse group of sensory functions may seem an unlikely combination to form a sensory system. We treat all of the somatic senses in one introductory chapter because they are mediated by one class of sensory neurons, the dorsal root ganglion neurons. Individual neurons in a dorsal root ganglion respond selectively to specific types of stimuli because of morphological and molecular specialization of their peripheral terminals.
In this chapter we consider the principles common to all dorsal root ganglion neurons and those that distinguish their individual sensory function. We begin with a description of the peripheral nerves and their organization, followed by a survey of the receptors responsible for each of the major bodily senses. We then consider their sensory pathways in the spinal cord and brain stem. The chapter concludes with a discussion of the central processing centers for each submodality in the thalamus. The physiological function of touch, pain, proprioception, and autonomic regulation are described in more detail in separate chapters.
The Primary Sensory Neurons of the Somatosensory System Are Clustered in the Dorsal Root Ganglia
Somatosensory information from the skin, muscles, joint capsules, and viscera is conveyed by dorsal root ganglion neurons innervating the limbs and trunk or by trigeminal sensory neurons that innervate cranial structures (the face, lips, oral cavity, conjunctiva, and dura mater). These sensory neurons perform two major functions: the transduction and encoding of stimuli into electrical signals and the transmission of those signals to the central nervous system.
The cell body of a dorsal root ganglion neuron lies in a ganglion on the dorsal root of a spinal or cranial nerve. Dorsal root ganglion neurons originate from the neural crest and are intimately associated with the nearby segment of the spinal cord.
Dorsal root ganglion neurons are a type of bipolar cell, called pseudo-unipolar cells. The axon of a dorsal root ganglion neuron has two branches, one projecting to the periphery and one projecting to the central nervous system (Figure 22-1). The peripheral terminals of different neurons innervate the skin, muscle, joint capsules, or viscera and contain receptors specialized for particular kinds of stimuli. They differ in receptor morphology and stimulus selectivity. The central branches terminate in the spinal cord or brain stem, forming the first synapses in somatosensory pathways. Thus the axon of each dorsal root ganglion cell serves as a single transmission line with one polarity between the receptor terminal and the central nervous system. This axon is called the primary afferent fiber.
Figure 22-1 The dorsal root ganglion neuron is the primary sensory receptor cell of the somatosensory system. The neuron cell body is located in a dorsal root ganglion adjacent to the spinal cord. The axon has two branches, one projecting to the periphery, where its specialized terminal contains receptors for a particular form of stimulus energy, and one projecting to the spinal cord or brain stem, where the afferent signals are processed.
Individual primary afferent fibers innervating a particular region of the body, such as the thumb or fingers, are grouped together into bundles or fascicles of axons forming the peripheral nerves. They are guided during development to a specific location in the body by various trophic factors. The peripheral nerves also include motor axons innervating nearby muscles, blood vessels, glands, or viscera.
Damage to peripheral nerves or their targets in the brain may produce sensory deficits in more than one somatosensory submodality. Knowledge of where somatosensory modalities overlap morphologically, and where they diverge, facilitates diagnosis of neurological disorders and malfunction.
Peripheral Somatosensory Nerve Fibers Conduct Action Potentials at Different Rates
The diverse modalities of somatic sensation are mediated by peripheral nerve fibers that differ in diameter and conduction velocity. Mechanoreceptors for touch and proprioception are innervated by dorsal root ganglion neurons with large-diameter, myelinated axons that conduct action potentials rapidly. Thermal receptors, nociceptors, and other chemoreceptors have small-diameter axons that are either unmyelinated or thinly myelinated; these nerves conduct impulses more slowly. The difference in conduction velocity allows signals of touch and proprioception to reach the spinal cord and higher brain centers sooner than noxious or thermal signals.
Large-diameter fibers conduct action potentials more rapidly because the internal resistance to current flow along the axon is low, and the nodes of Ranvier are widely spaced along its length (see Chapter 6). The conduction velocity of large myelinated fibers (in meters per second) is approximately six times the axon diameter (in micrometers), whereas thinly myelinated fibers conduct at five times the axon diameter. For unmyelinated fibers, the factor for converting axon diameter to conduction velocity is 1.5 to 2.5.
Peripheral nerve fibers are classified into functional groups based on properties related to axon diameter and myelination, conduction velocity, and whether they are sensory or motor. The first classification scheme was devised in 1894 by Charles Sherrington, who measured the diameter of myelin-stained axons in sensory nerves, and subsequently codified by David Lloyd (Table 22–1). They found two or three overlapping groups of axonal diameters (Figure 22-2). It was later discovered that in muscle nerves these anatomical groupings are functionally important. Group I axons innervate muscle-spindle receptors and Golgi tendon organs, which signal muscle length and contractile force. Group II fibers innervate secondary spindle endings and receptors in joint capsules; these receptors also mediate proprioception. Group III fibers, the smallest myelinated muscle afferents, and the unmyelinated group IV afferents signal disorders in muscles and joints that can be sensed as painful.
Table 22-1 Classification of Sensory Fibers in Peripheral Nerves1
Figure 22-2 Peripheral nerves innervating skeletal muscle and the skin contain several types of sensory nerve fibers. The graphs illustrate the distribution of four groups of sensory nerve fibers innervating skeletal muscle and the skin. Each group has a characteristic axon diameter and conduction velocity. Light blue lines are the sum of fibers in each group in the zones of overlap. The conduction velocity of myelinated peripheral nerve fibers is approximately six times the fiber diameter. (Adapted, with permission, from Boyd and Davey 1968.)
Cutaneous nerves contain two sets of myelinated fibers: Group II fibers innervate cutaneous mechanoreceptors that respond to touch, and group III fibers mediate thermal and noxious stimuli. Unmyelinated group IV cutaneous afferents, like those in muscle, also mediate thermal and noxious stimuli.
Another method for classifying peripheral nerve fibers is based on electrical stimulation of whole nerves. In this widely used diagnostic technique nerve conduction velocities are measured between pairs of stimulating and recording electrodes placed on the skin above a peripheral nerve. When studying conduction in the median or ulnar nerve, for example, the stimulation electrode might be placed on the wrist and the recording electrode on the upper arm. Brief electrical pulses applied through the stimulating electrode evoke action potentials in the nerve that are recorded a short time later in the arm. The recorded signal represents the summed action potentials of all of the nerve fibers excited by the stimulus pulse and is called the compound action potential. It increases in amplitude as more nerve fibers are stimulated; the summed activity is roughly proportional to the total number of active nerve fibers.
Electrical stimuli of increasing strength evoke action potentials in the largest axons first, for they have the lowest electrical resistance, and then in progressively smaller axons. The earliest signal recorded in the compound action potential occurs in fibers with conduction velocities greater than 90 m/s. Called the Aα wave (Figure 22-3), this signal reflects the action potentials generated in group I fibers and in motor neurons innervating skeletal muscle. The sensation is barely perceived by the subject in the region innervated.
Figure 22-3 Conduction velocities of peripheral nerves are measured clinically from compound action potentials. Electrical stimulation of a peripheral nerve at varying intensities activates different types of nerve fibers. The action potentials of all the nerves stimulated by a particular amount of current are summed to create the compound action potential. The distinct conduction velocities of different classes of sensory and motor axons produce multiple deflections. (Adapted, with permission, from Erlanger and Gasser 1938.)
A second signal, the Aβ wave, appears as more large fibers are recruited. This component corresponds to group II fibers in skin or muscle nerves and becomes larger as the shock intensity is increased. At higher voltages, when axons in the smaller AΔ range are recruited, the stimulus becomes painful, resembling an electric shock produced by static electricity. Voltages sufficient to activate unmyelinated C fibers evoke sensations of burning pain. Stimulation of motor neurons innervating muscle spindles evoke an intermediate wavelet called the Aγ wave, but this is usually difficult to discern because the conduction velocities of these motor neurons overlap those of Aβ and AΔ sensory axons.
The clinician takes advantage of the known distribution of the conduction velocities of afferent fibers in peripheral nerves to diagnose diseases that result in sensory-fiber degeneration or motor neuron loss. In certain conditions the loss of axons is selective; in the neuropathy characteristic of diabetes, for example, the large-diameter sensory fibers degenerate. Such a selective loss is reflected in a reduction in the appropriate peak of the compound action potential, a slowing of nerve conduction, and a corresponding diminution of sensory capacity. Similarly, in multiple sclerosis the myelin sheath of large-diameter fibers in the central nervous system degenerates, producing slowing of nerve conduction or failure of impulse transmission.
Rapid conduction in a peripheral nerve axon solves two problems. First, it provides the prompt sensory feedback required for motor control. The greatest velocities are in the afferent fibers from muscle that signal muscle length, contraction velocity, and force. Any delay in the feedback signal would cause instability, which probably explains why the fibers innervating spindles have the greatest diameters among peripheral axons.
Conduction-delay dispersion poses a second significant problem because conduction velocity varies among afferent fibers. Synchronous firing of a set of neurons becomes desynchronized by the time the action potentials reach the postsynaptic cells by an amount that is proportional to the mean conduction delay and the variation in conduction velocities. If the delay is great, either because the pathway is long or because the conduction velocities are low, the desynchronization can be substantial.
As a general rule, conduction velocity throughout the nervous system is correlated with the need to maintain synchrony. The disparity in conduction times from a fingertip to the spinal cord can be calculated from the conduction velocities in Table 22–1 and by considering that the distance is approximately 0.8 m. In C fibers delays range from 0.8 s to 1.8 s, the mean delay is about 1.3 s, and the spike arrival times are distributed over about 1 s; thus events occurring more often than once per second are smeared together. In Aα fibers delays range from 7.5 ms to 12.5 ms, the mean delay is 10 ms, and the arrival times vary by only 5 ms; events can therefore occur as often as 200 per second without smearing. The comparable limits for AΔ and Aβ fibers are approximately 8 Hz and 80 Hz. A blind person can scan his or her fingers across Braille dot patterns at remarkable rates of up to 100 mm/s with little loss of information because tactile information is conveyed by Aβ afferents, which maintain synchrony to within 12 ms.
Many Specialized Receptors Are Employed by the Somatosensory System
The particular receptor class expressed in the nerve terminal of a sensory neuron determines the type of stimulus detected by the neuron. The peripheral axons of the sensory neurons that mediate touch and proprioception terminate in a nonneural capsule. They sense mechanical stimuli that indent or otherwise physically deform the receptive surface. In contrast the peripheral axons of neurons that detect noxious, thermal, or chemical events have unsheathed endings with multiple branches.
When a somatic receptor is activated by an appropriate stimulus, the terminal of the sensory neuron is typically depolarized. Stimuli of sufficient strength produce action potentials that are transmitted along the peripheral branch of the neuron’s axon and into the central branch that terminates in the spinal cord or brain stem.
A variety of morphologically specialized receptors underlie the various somatosensory submodalities. For example, the median nerve that innervates the skin of the hand and some of the muscles controlling the hand contains tens of thousands of nerve fibers that can be classified into 30 functional types. Of these, 22 types are afferent fibers (sensory axons conducting impulses toward the spinal cord), and eight types are efferent fibers (motor axons conducting impulses away from the spinal cord to skeletal muscle, blood vessels, and sweat glands). The afferent fibers convey signals from eight kinds of cutaneous mechanoreceptors that are sensitive to different kinds of skin deformation; five kinds of proprioceptors that signal information about muscle force, muscle length, and joint angle; four thermoreceptors that report the temperatures of objects touching the skin; four nociceptors that signal potentially injurious stimuli; and at least one kind of itch receptor. The major receptor groups within each submodality are listed in Table 22–2.
Mechanoreceptors Mediate Touch and Proprioception
Mechanoreceptors sense physical deformation of the tissue in which they reside. Mechanical distension, such as pressure on the skin or stretch of muscles, is transduced into electrical energy by the physical action of the stimulus on cation channels in the membrane. Mechanical stimulation deforms the receptor protein, thus opening stretch-sensitive ion channels and increasing Na+, and Ca2+ conductances that depolarize the receptor neuron. Removal of the stimulus relieves mechanical stress on the receptor and allows stretch-sensitive channels to close.
Some mechanoreceptor ion channels are activated directly by forces applied to the tissue, permitting rapid activation and inactivation. For example, Pacinian corpuscle receptors in the skin can respond to vibration at frequencies as high as 500 Hz, firing one impulse for each vibratory cycle. This means that the receptor is capable of firing an impulse every 2 ms for sustained periods.
Various mechanisms for direct activation of mechanoreceptor ion channels have been proposed. Some mechanoreceptors appear to respond to forces conveyed through tension in the lipids of the plasma membrane (Figure 22–4A). This may be the mechanism for detection of cellular swelling, which plays an important role in osmoregulation.
Figure 22-4 Ion channels in mechanoreceptor nerve terminals are activated by mechanical stimuli that stretch or deform the cell membrane. Mechanical displacement leads to channel opening, permitting the influx of cations. (Modified, with permission, from Lin and Corey 2005.)
A. Channels can be directly activated by forces conveyed through lipid tension in the cell membrane, such as osmotic swelling.
B. Forces conveyed through structural proteins linked to the ion channel can also directly activate channels. The linking proteins may be either extracellular (attached to the surrounding tissue) or intracellular (bound to the cytoskeleton) or both.
C. Channels can be indirectly activated by forces conveyed to a force sensor (a separate protein) in the membrane. An internal second messenger carries the sensory signal from the mechanosensitive protein to the channel.
Another mechanism for direct activation of mechanoreceptors is linking the channel to the surrounding tissue of the skin or to muscle cell membranes through structural proteins. The extracellular linkage is elastic and often represented as a spring, whereas the intracellular portion of the channel is anchored directly to proteins of the cytoskeleton (Figure 22–4B). Direct channel gating in this model may be produced by forces perpendicular or parallel to the receptor cell membrane that stretch the extracellular linkage protein. This type of direct channel gating may be used by hair cells of the inner ear. Similar mechanical linkages between the skin and cutaneous mechanoreceptors have been postulated.
Likewise, mechanical coupling of sensory nerve terminals to skeletal muscle or tendons is thought to underlie proprioception. Unfortunately, because these receptors are embedded in nonneural tissue and thus difficult to isolate for biochemical analysis, the proteins involved in transduction have not been identified in mammals. Studies of invertebrates suggest that the transduction molecules for mechanosensation in skin and muscle may belong to the degenerin superfamily, which includes ion channels related to vertebrate epithelial Na+ channels.
Some mechanoreceptor ion channels are activated indirectly through second-messenger pathways. In this case the force sensor in the receptor’s cell membrane is a protein distinct from the ion channel (Figure 22-4C). A variety of intracellular messengers signal stimulation of the sensor to the ion channel, causing the channel to open. Unlike direct activation, the indirect pathway is slow to activate and inactivate, often outlasting the stimulus. The great advantage of the second-messenger mechanism of course is that the sensory signal is amplified; the conductance of many ionic channels can be affected by the activation of a single sensor molecule in the receptor cell. These properties are consistent with the responses of pain receptors sensitive to mechanical damage of the skin, such as pinch, or excessive distension of viscera. David Corey and co-workers have suggested that these sensations are mediated by TRPV4 receptors, a class of transient receptor potential (TRP) receptors that are also involved in thermal senses (see below).
The specialized, nonneural end organ that surrounds the nerve terminal of a mechanoreceptor nerve must be deformed in specific ways to excite the nerve. For example, individual receptors may respond selectively to pressure or motion, and may detect the direction of force applied to the skin, joints, or muscle fibers. The end organ can also amplify or modulate the sensitivity of the receptor to mechanical displacement.
The skin has eight types of mechanoreceptors that are responsible for the sense of touch (see Table 22–2). They are described briefly here and in greater detail in Chapter 23. The glabrous skin of the hands and feet contains four kinds of mechanoreceptors: Meissner corpuscles, Merkel cells, Pacinian corpuscles, and Ruffini endings (Figure 22-5). Two of these receptors are classified as slowly adapting (SA) because they continue to fire in response to steady pressure on the skin. The other two receptors are rapidly adapting (RA), responding to motion on the skin but not to steady pressure. They also differ in receptor size and location within the skin.
Figure 22-5 Touch is mediated by four types of mechanoreceptors in the human hand. The terminals of myelinated sensory nerves innervating the hand are surrounded by specialized structures that detect contact on the skin. The receptors differ in morphology, innervation patterns, location in the skin, receptive field size, and physiological responses to touch. (Adapted, with permission, from Johansson and Vallbo 1983.)
A. The superficial and deep layers of the glabrous (hairless) skin of the hand each contain distinct types of mechanoreceptors. The superficial layers contain small receptor cells: Meissner corpuscles and Merkel cells. The sensory nerve fibers innervating these receptors have branching terminals such that each fiber innervates multiple receptors of one type. The deep layers of the skin and subcutaneous tissue contain large receptors: Pacinian corpuscles and Ruffini endings. Each of these receptors is innervated by a single nerve fiber, and each fiber innervates only one receptor. The receptive field of a mechanoreceptor reflects the location and distribution of its terminals in the skin. Touch receptors in the superficial layers of the skin have smaller receptive fields than those in the deep layers. (RA1, rapidly adapting type 1; RA2, rapidly adapting type 2; SA1, slowly adapting type 1; SA2, slowly adapting type 2.)
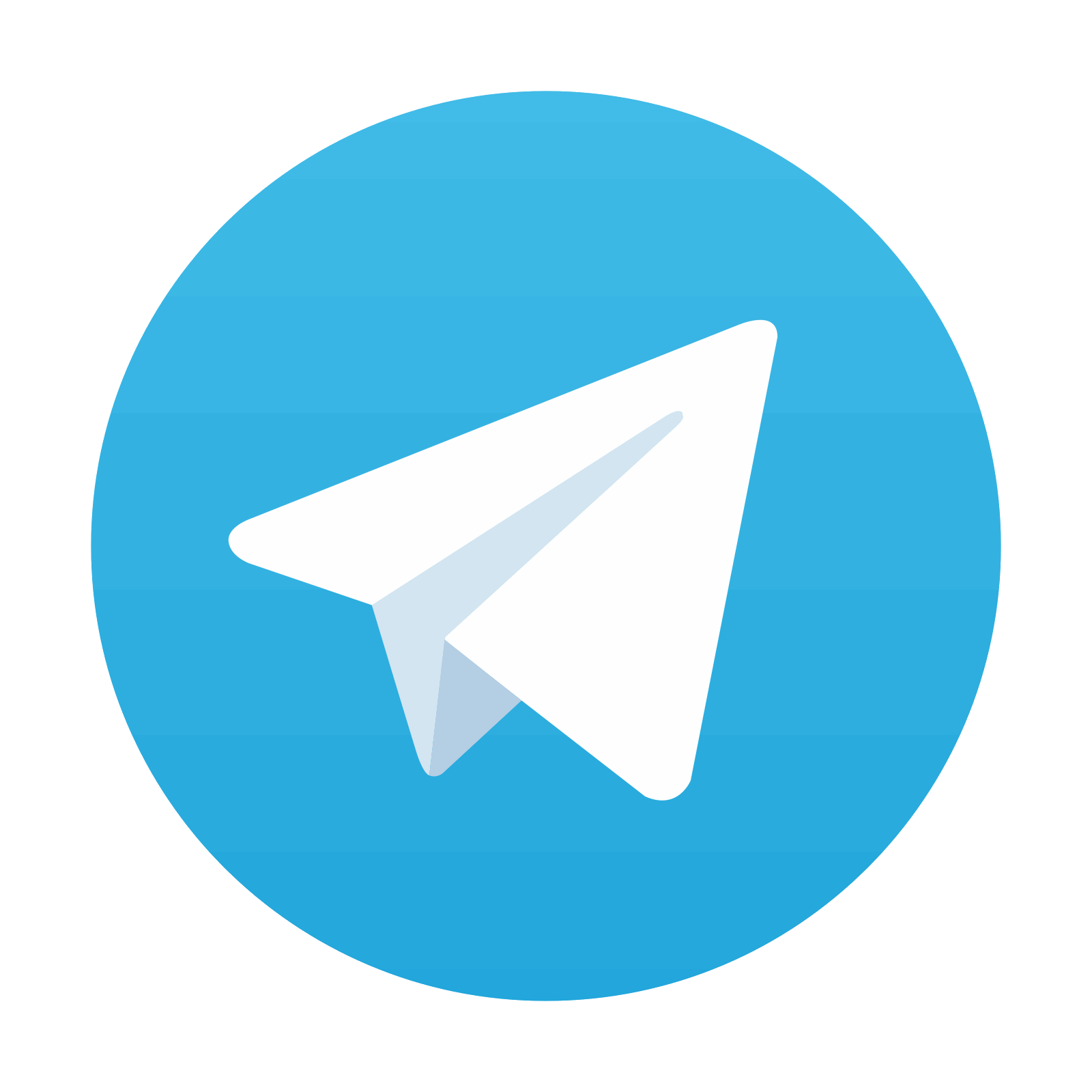
Stay updated, free articles. Join our Telegram channel

Full access? Get Clinical Tree
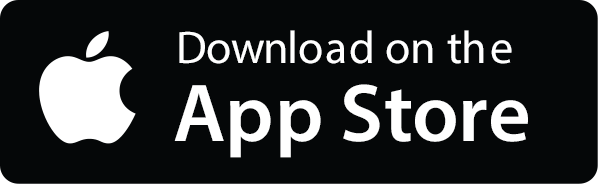
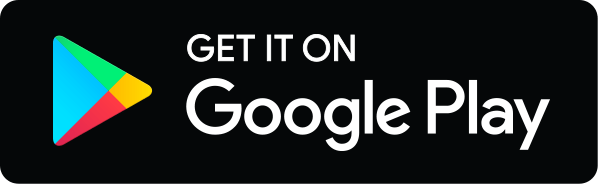