Fig. 1
Transcriptional targeting of AAV vectors in the rat striatum. Exclusively neuronal transgene expression is shown after AAV-5-hSYN-EGFP transduction (left panel; shown is an overlay of green = EGFP fluorescence with red = NeuN immunoreactivity); exclusively astrocytic transgene expression is shown after AAV-5-GFAP-EGFP transduction (right panel; shown is an overlay of green = EGFP fluorescence with red = GFAP immunoreactivity). Both vectors were injected into the brain at 6 × 10e9 vg. Animals were sacrificed at 1 month after vector application and tissue sections were stained with anti-NeuN (neuronal marker) or anti-GFAP (astrocytic marker). The sketches show the transduced cell types and shall serve as reminders that striatal neurons project to far remote brain structures over several mm or even cm, making it likely that a secreted transgene like a neurotrophic factor is delivered to these potentially off-target sites, while astrocytes have a much more restricted area of influence. Identical results as shown here for AAV-5 were obtained with AAV vectors of the hybrid serotype 1/2 and serotype 6
Transcriptional control elements are the third level of targeting therapeutic transgene expression to specific brain cell populations, and in terms of efficacy and feasibility appear to be the easiest to achieve. However, as illustrated in Fig. 1, targeting transgene expression by means of cell-type specific promoters or binding sites for micro-RNAs by no means alters the transduction properties of the viral capsid itself. Thus, detection of transgene expression only in neurons does not mean that the recombinant virus would not bind to glial cell surface receptors and enter astrocytes with equal efficacy, but only that in these cells the neuron-specific promoter is expressing with negligible activity. Although with the neuron- and astrocyte-specific promoters described in this chapter we never detected transgene expression in microglia, this does not prove absence of microglial transduction and potential antigen-processing and presentation. Indeed, at least some microglial transgene expression was suggested to occur after injection of AAV -2 and AAV-5 vectors into the brain which drove expression from the macrophage-specific F4/80 promoter [8].
2 A History of Our Attempts to Achieve Neuron – and Astrocyte-Specific Transgene Expression in the Brain
The chapters in this series are thought to be “protocols” for design of optimized viral vectors, with potential use in gene therapeutic applications. However, there is no simplified protocol, which will allow the reader to generate transcriptionally targeted viral vectors specifically for certain neuronal or glial brain cell populations, simply because the predictive powers of algorithms suggesting binding of cell-type-specific transcription factors to DNA sequences are quite limited. In addition, promoter elements defining cell specificity may reside far upstream from transcription initiation, making it necessary to use relatively bulky promoter constructs. While this is not a major problem for high-capacity (up to 30 kbp) adenoviral vectors, and may work with the cloning capacity of lentiviral vectors (up to 8 kbp), it represents a major hurdle for the AAV vectors with maximum capacities around 5 kbp. Thus, the only protocol to propose here is to simply go for trial and error: clone the respective promoter sequence into your vector of choice, and evaluate cell-type specificity over a wider range of titers, and, in case of AAVs, serotypes with different transduction and tissue spreading efficacies, and in different brain areas. Once suitable specificity has been achieved with a respective DNA sequence and AAV serotype , one can serially shorten the promoter sequence to make it more applicable especially for vectors with limited genome capacity, or combine the promoter with de-targeting miR-binding sites to further increase cell-type specificity (see description of transcriptional control elements in Subheading 3). However, how little one can trust even promoter sequences functionally proven in transgenic mice when transferring them to viral vectors will be illustrated by our work described below, aiming to transcriptionally target AAVs to astrocytes. Although the text will be somehow anecdotal, the author hopes that along the line of our experiments several important principles of transcriptionally targeting will become evident.
We started our attempts to target viral vectors specifically to neurons more than a decade ago, when still using first-generation adenoviral vectors of the human serotype 5 (Ad5). The capsid of these vectors had a relatively strong glial tropism, i.e., use of ubiquitous promoters such as the popular CMV promoter resulted in mainly astroglial transgene expression. For certain experiments we could still use this type of vector to achieve specific transduction of distinct neuronal populations, by making use of the retrograde transport of Ad5 capsids in nerve fibers: application of Ad5 with CMV promoter at the transected optic nerve resulted in transgene expression specifically in retinal ganglion neurons, which project into the optic nerve [9] (see Subheading 1). However, this vector was not suitable for neuron-specific transgene expression elsewhere in the brain .
Thus, we tested a variety of promoters in Ad5 vectors for their neuron specificity against the human CMV promoter: the human synapsin 1 gene promoter (hSYN; 470 bp), the rat neuron-specific enolase promoter (NSE; 1800 bp), the rat tubulin alpha-1 gene promoter (Ta1; 450 bp), and the human U1 snRNA gene promoter (U1; 400 bp) [10]. For a first assessment of any promoter fragment a good in vitro test system is important, allowing to quickly proceed through multiple constructs. We used primary neuron/glia co-cultures for this purpose, and found that the hSYN promoter offered the best overall performance in terms of promoter size, neuronal expression level, and rapid onset of transgene expression. The favorable properties of the hSYN promoter were then confirmed in the rat brain , where we for the first time achieved long-lasting and virtually 100 % neuron-specific transgene expression with a first-generation adenoviral vector [11]. These results were extended by Glover et al. [12] who showed that the hSYN promoter remained absolutely neuron specific even at largely increased expression levels, with high-titer vectors and incorporation of the mRNA stabilizing woodchuck hepatitis virus posttranscriptional regulatory element (WPRE [13]), resulting in greatly increased protein expression rate.
However, first-generation Ad5 vectors provoked inflammatory responses in the rodent brain when used at higher titers. Thus, we moved on to adeno-associated viral (AAV ) vectors [14, 15]. Here again, we aimed to confirm that the hSYN promoter is an adequate pan-neuronal promoter. As tissue-specific promoters are frequently claimed to have only weak expression levels, we compared the hSYN promoter to the very strong mouse CMV (mCMV) promoter, under the assumption that the proposed neuronal tropism of the AAV-2 capsid would allow expression from both promoters in neurons only. In cultured primary neuron/glia co-cultures we found that the mCMV promoter resulted in exclusively astrocyte-specific expression, while the hSYN promoter restricted expression exclusively to neurons. Thus, a promoter with high activity in glia was able to “de-target” a so-called neuronotrophic AAV quite efficiently. Secondly, in terms of transgene expression levels, the mCMV promoter was a bit ahead of the hSYN promoter, but the latter kept up to equal expression level after only a few days in culture. Thus, a cell-type-specific promoter is by no means necessarily a weak promoter, although this may depend on enhancing elements as the WPRE. When testing both hSYN and mCMV promoter in AAV-2 vectors in different brain areas we elucidated another important principle: results obtained under primary cell culture conditions are not inevitably the same in the adult brain. In the retina, hSYN expressed exclusively in neurons, and mCMV very weakly in Müller glia; in the cortex hSYN expressed exclusively in neurons, while mCMV expressed very weakly in neurons but moderately in astrocytes; in the thalamus, both hSYN and mCMV expressed strongly and exclusively in neurons. Lessons to be learned from such experimentation was that (1) the hSYN promoter under all conditions demonstrated exclusively neuron-specific expression, but that (2) a promoter like the mCMV may have very different expression characteristics depending on use in cultured brain cells or in vivo, and even depending on brain area under investigation.
Since astroglia-specific expression was only partially achievable so far, we next exploited astrocyte-specific promoters proven to work as such in transgenic animals. Both the full-length 2.2 kbp glial fibrillary acidic protein (GFAP) promoter and its much shorter variant, the GfaABC1D promoter, drove transgene expression exclusively in astrocytes in transgenic mice [16]. With the full-length GFAP promoter we indeed achieved a virtually exclusive astroglial expression both in neuron/glia co-cultures and in the rodent brain [17]. These experiments were conducted with several AAV serotypes (hybrid-1/2, 5, and 6) and for all these vectors resulted in the same outcome: transgene expression was exclusively neuronal under control of the hSYN promoter, and exclusively astrocytic under the control of the GFAP promoter (see Fig. 1). Thus, for AAV serotypes with quite different tissue transduction properties (AAV-6: high-level transduction, restricted area; AAV-1/2: high-level transduction, widespread; AAV-5: low-level transduction, widespread) a highly efficient and mutually exclusive transcriptional targeting was proven. An important lesson to learn from these experiments is: if a viral vector is described to result in transduction of a certain cellular population in a given tissue, it may very well be that a different promoter would result in a completely different outcome. It is important to remember that transcriptional targeting and targeting by modification of capsid tropism are two completely independent approaches.
After having achieved a strict astrocytic transgene expression, which was exploited to restrict the delivery of neurotrophic factors to defined brain areas by avoiding off-target delivery through long-range neuronal projections, we next sought to gain temporal control over transgene expression. We thus put together a regulated gene transfer system, which is based on the mifepristone-regulated GeneSwitch (GS) [18, 19]. Such regulated transgene expression systems (as described in detail elsewhere in this issue) require a second expression cassette to be expressed together with the therapeutic transgene of choice, the latter under control of the regulated promoter. Thus, space restrictions are likely to encounter when using small viruses as the AAVs, and thus we aimed to replace the bulky full-length GFAP promoter with a variant already cut down to less than 700 bp, the so-called GfaABC1D promoter [16]. By this means it would be possible to construct a single vector genome for both astrocyte-specific GS expression and regulated GDNF expression. Unfortunately, however, despite the fact that the GfaABC1D promoter restricted transgene expression exclusively to astrocytes in transgenic mice, and despite promising results in neuron/glia co-culture, this promoter was not able to restrict transgene expression to astrocytes in AAV vectors (expression was about 70 % neuronal and 30 % astroglial [19]). Thus, the predictive power of both transgenic animals and cell culture experiments was not sufficient to foresee the characteristics of this promoter in the AAV-5 and AAV-6 gene transfer vectors investigated. Addition of neuron-specific mir124-binding sites into the 3′-untranslated region of an expression cassette driven by the GfaABC1D promoter drastically diminished neuronal expression, but also impacted on expression levels in astrocytes (Taschenberger and Kügler, unpublished), rendering this de-targeting approach almost useless under the conditions applied, although it worked out very well in the context of lentiviral vectors [20].
3 Setscrews for Transcriptional Control
To summarize the transcriptional targeting approaches described above in a more general format the principal “screws” that can be important for cell-type-specific transgene expression are summarized in Fig. 2. Shown is the prototype genome of a recombinant AAV vector, but the basic principles are the same for lentiviral vectors. It should be taken into account, however, that AAVs mainly persist as epigenomic concatamers, while LVs integrate into the host cell genome, often at sites of active transcription. Thus, influences from neighboring genomic sequences cannot be ruled out, and results obtained for LVs must be experimentally confirmed with AAVs and vice versa.


Fig. 2
A prototype AAV vector genome containing the elements useful for transcriptional targeting
The potential impact of each vector genome element on transcriptional targeting is described below.
“ITR”: the inverted terminal repeats flanking the recombinant vector genome are necessary for packaging the genome into the viral capsid . These sequences demonstrate relatively weak but readily detectable promoter activity on their own, allowing to construct “promoter-less” genomes for incorporation of very large transgenes.
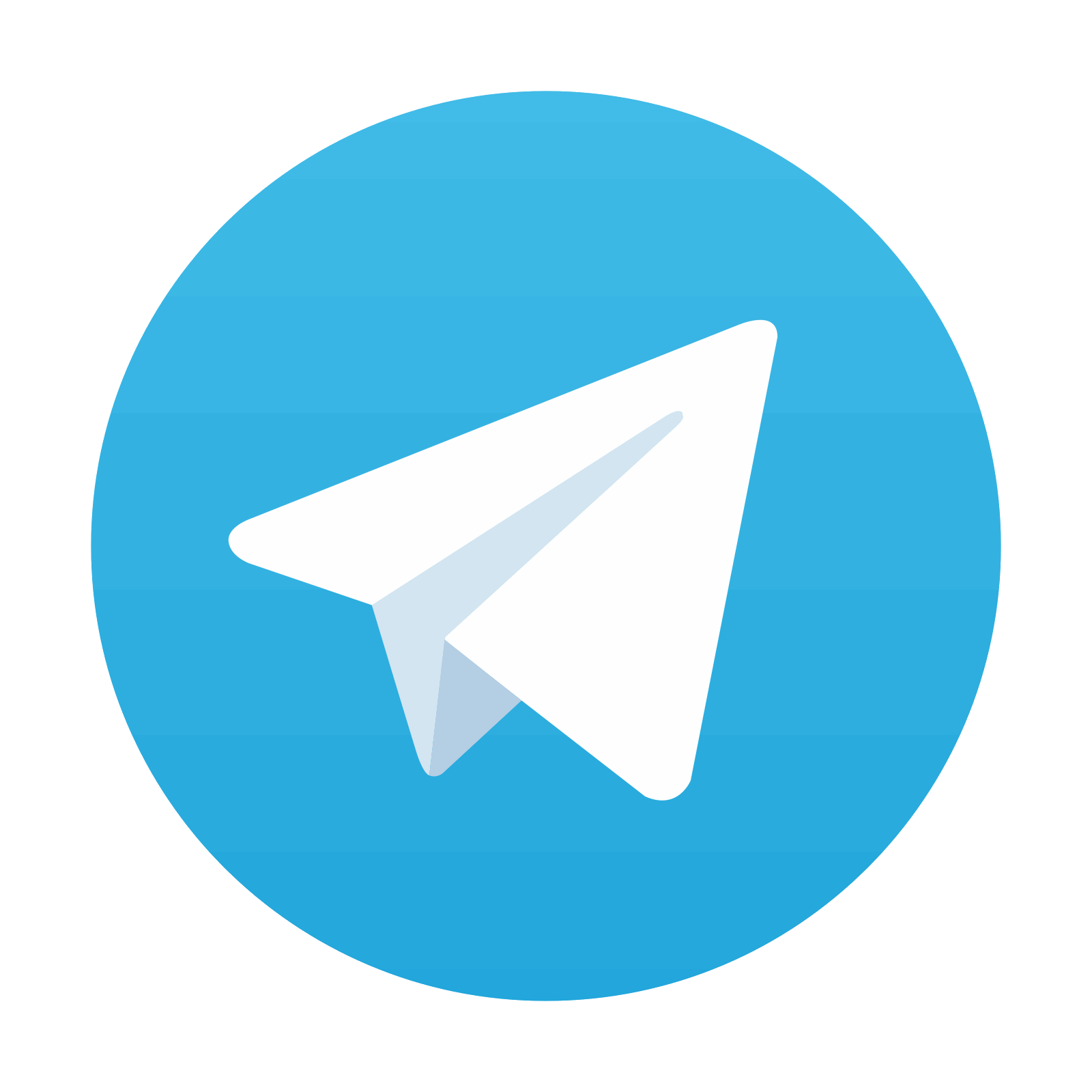
Stay updated, free articles. Join our Telegram channel

Full access? Get Clinical Tree
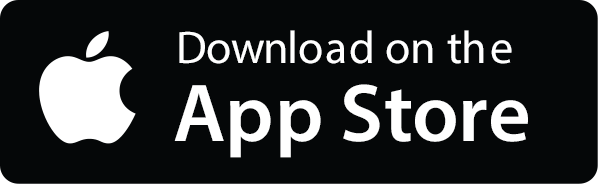
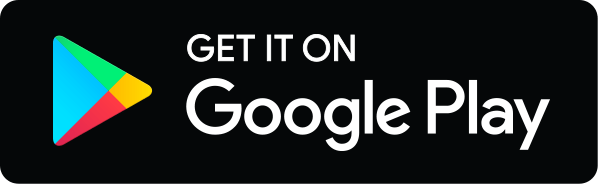