Fig. 1
Pathways transmitting activation from hypothalamic orexin neurons to cardiorespiratory outputs. a One method often used to activate orexin neurons involves local microinjections of GABAA receptor antagonists, such as bicuculline or gabazine, in anesthetized rodents. Compared to the anesthetized baseline state, such injections powerfully disinhibit orexin neurons, as indicated by massive expression and accumulation in their nuclei of c-Fos, a protein product of an early gene. The approach has been introduced prior to the discovery of orexin neurons as the means of producing cardiorespiratory, motor and electrocortical activation similar to that observed during a systemic defense response (e.g., Shekhar and DiMicco 1987). Posterior hypothalamic bicuculline or gabazine microinjections activate orexin neurons, as well as other local neurons that also may have widespread projections (Lu et al. 2007). In panel a′ (left), many cells located in the perifornical region of the posterior hypothalamus have c-Fos-stained nuclei following microinjection of 20 nl of 1 mM bicuculline in an urethane-anesthetized rat. Panel b′ shows an expanded frame marked in panel a′. Arrowheads point to selected c-Fos-stained nuclei (black) in orexin-positive neurons (brown) and arrows point to c-Fos-stained nuclei in non-orexin neurons (cell body not labeled). Unpublished data from the study of Lu et al. (2007) f—fornix. b Major descending projections from hypothalamic orexin neurons to the most relevant for cardiorespiratory regulation locations and cell groups in the brainstem and spinal cord. The scheme includes direct orexin cell projections to major targets, such as pontomedullary noradrenergic neurons (locus coeruleus—LC, A7, Sub-coeruleus region—Sub-C, A5, A2/C2 and A1/C1 groups) and medullary serotonergic neurons of the obscurus, pallidus and parapyramidal regions, as well as spinal and cranial motoneurons and preganglionic sympathetic neurons. Among the latter are those that control brown adipose tissue (BAT) and activate heat production and those responsible for vasoconstriction. Notably, spinal and cranial motoneurons and preganglionic sympathetic neurons can be activated directly by orexin-containing axon terminals and indirectly through aminergic cells that are themselves activated by orexin projections. Accordingly, orexinergic activation acts, in part, through enhancement of aminergic activation. Orexin neurons also send axons to numerous other pontomedullary regions with well-established roles in cardiorespiratory regulation. These include the pontine parabrachial region where the noradrenergic A7 group is located, the medullary retrotrapezoid region containing central chemosensory neurons, and other ventrolateral (V-L) medullary neurons that are part of the central respiratory rhythm and sympathetic tone generators. PPT & LDT—cholinergic neurons of the pedunculopontine tegmental and laterodorsal tegmental nuclei
2 Endogenous Respiratory Effects of Orexins
Baseline ventilation in orexin knock out (KO) mice does not differ from that in the control, wild-type mice but KO animals exhibit reduced ventilatory response when breathing is stimulated by increased level of inspired CO2. In rats, pharmacological activation of orexin and other adjacent neurons in the posterior, lateral hypothalamus activates hypoglossal motoneurons that in obstructive sleep apnea (OSA) patients need to be activated to protect the airway against collapse during sleep. However, while genetic association studies show increased incidence of sleep apnea in patients with narcolepsy-cataplexy, it is the hypoxic ventilatory response, rather than the ventilatory sensitivity to CO2, that is attenuated in narcolepsy-cataplexy patients. There are several possible explanations for these not fully concordant findings.
2.1 Orexin Contribution to Central CO2 Sensitivity
Stimulation of breathing by elevated inspiratory CO2 increases expression of the early gene c-Fos in orexin and other adjacent hypothalamic neurons in both anesthetized (Johnson et al. 2012) and freely behaving mice (Sunanaga et al. 2009). This indicates increased firing rates of these neurons. Since hypercapnic activation of breathing is associated with activation of multiple primary and accessory respiratory muscles and elicits anxiety caused by the feeling of dyspnea, the increased c-Fos expression does not necessarily indicate a primary respiratory role of orexin neurons. Rather, their activation may be secondary to those other aspects of experimental stimulation of breathing by hypercapnia. Nevertheless, it is evident from the scheme in Fig. 1b that orexin cell activation that occurs during hypercapnic states can facilitate generation of enhanced ventilatory effort.
Other evidence from rodents further supports a role of orexin in ventilatory activation specifically related to hypercapnia and central acidification. In arterially perfused brainstem-spinal cord juvenile rat preparation, respiratory rate response to hypercapnia is attenuated by bath application of orexin-1 receptor antagonist (Corcoran et al. 2010). This suggests that any endogenous orexin that might be present in the brainstem of this in vitro preparation acts at the brainstem level to facilitate transmission of hypercapnic stimulus to the central respiratory rhythm generating neurons. Such an action and interaction may occur in the ventrolateral medullary retrotrapezoid nucleus neurons which are known central receptors of brain acidification (Lazarenko et al. 2011). However, an alternative explanation for these findings would be that relevant orexin receptors are constitutively active and pH-sensitive in the conditions of these experiments.
A more detailed investigation of the sleep-wake state-dependent role of orexins in modulation of ventilatory sensitivity revealed that orexins facilitate the ventilatory response to CO2 only during wakefulness (Nakamura et al. 2007). More specifically, the periods of wakefulness occurring during the dark phase when rodents are more active were associated with most pronounced endogenous orexinergic facilitation of hypercapnic ventilatory response and the ventrolateral medullary retrotrapezoid region was found to be an important site where such a state-dependent facilitation takes place (Dias et al. 2009). Orexin KO mice also had more frequent sleep-related central apneas (not associated with sighs) than wild-type mice, whereas hypoxic ventilatory response did not differ between the groups (Nakamura et al. 2007). Among these findings, the state-dependence of the effect of orexin on hypercapnic ventilatory response is consistent with the known state-dependence of orexin cell activity—activity present in wakefulness but absent, or nearly absent during sleep. However, given the absence of evidence for endogenous contribution of orexin to basal ventilation at rest, it would not be appropriate to conclude from the studies in which hypercapnia was used to stimulate breathing that a decrease in ventilation and the concomitant small increase in central CO2 level that normally occur at the onset of SWS are due to silencing of orexin neurons. The increased expression of central apneas in orexin KO mice is more puzzling because orexin cells are typically silent in SWS in normal animals. Therefore, it is not clear how genetically induced absence of orexin and absence of orexin effects due to cell silencing would differentially contribute to the occurrence of central apneas during sleep. It is likely that a developmental reconfiguration of the respiratory system in orexin KO mice results in a more frequent expression of apneas in this mouse strain.
Importantly, when tested in vitro, orexin neurons have intrinsic pH chemosensitivity similar to noradrenergic neurons of the locus coeruleus and other neurons located in the pontomedullary reticular formation, and the level of highest pH sensitivity is around 7.2 (Williams et al. 2007). Combined with the known efferent projections of orexin neurons to aminergic neurons, as well as sympathetic and respiratory neurons, of the brainstem (Fig. 1b), this observation is supportive of a facilitatory role of orexins in ventilatory response to hypercapnia. Equally plausible, however, is the possibility that the primary role of hypercapnic activation of orexin neurons is to mediate the increased arousal and anxiety elicited by the hypercarbia-induced feeling of dyspnea (Williams et al. 2007). This function would be mediated by multiple suprapontine and ascending connections of orexin neurons that are outside of the scope of this discussion.
2.2 Orexin Effects on the Brainstem Respiratory Network and Motoneurons Innervating the Upper Airway Muscles
Orexin neurons send axonal projections to both cranial and spinal motoneurons. The role of these connections may be to facilitate motor activation in synchrony with the states of active wakefulness when orexin cells are more active than in other states. From the perspective of this review, it is particularly interesting whether endogenous orexinergic tone activates respiratory motoneurons, such as those innervating the diaphragm and intercostal muscles of the respiratory pump, and those that control the accessory respiratory muscles of the upper airway, such as hypoglossal (XII cranial nerve), facial (V) or laryngeal (X). Activation of upper airway motoneurons is especially important in OSA patients. In contrast to healthy persons, these patients require elevated levels of upper airway muscle activity in order to maintain the airway open. When this activity declines, as it typically does at sleep onset, the airway collapses (usually in the pharyngeal region), and the patient needs to awaken to resume breathing. Given the elevated activity level and prominent sleep-wake state-dependence of upper airway muscle activity in OSA patients, it is possible that endogenous orexinergic tone contributes to activation of upper airway motoneurons during wakefulness.
We and others have previously determined that norepinephrine- and serotonin-containing brainstem neurons provide a major component of the wakefulness related drive to XII motoneurons (Chan et al. 2006; Fenik et al. 2005). The relevance of this is that XII motoneurons innervate the muscles of the tongue which in OSA patients protect the airway from collapse by causing an anterior shift of the tongue which widens the lumen of the upper airway (Remmers et al. 1978; Sauerland and Harper 1976). As the scheme in Fig. 1b indicates, the wake-related activation of upper airway motoneurons may occur through their direct activation by axonal projections from hypothalamic orexin neurons and indirectly through orexinergic activation of brainstem aminergic neurons. To investigate these pathways, we used urethane-anesthetized rats in which orexin neurons are activated by local hypothalamic injections of GABAA receptor antagonists (bicuculline or gabazine). In the first study of this series, we determined that disinhibition of perifornical hypothalamic neurons, including the ones that express orexins (Fig. 1a), powerfully activated XII motoneurons and increased respiratory rate parallel to activation of cortical and hippocampal activity in a manner analogous to that in an aroused state (Lu et al. 2007). We then determined that a major component of the activation seen in XII motoneurons was preserved when noradrenergic and serotonergic excitatory effects on XII motoneurons were blocked at the level of the XII nucleus by local microinjections of a combination of α1-adrenergic and serotonergic antagonists (Fenik et al. 2009). This suggested that a major activation of XII motoneurons in this model is mediated by direct projections from the perifornical hypothalamus to the XII motor nucleus, including the projections originating in orexin neurons (Fig. 1b). To test this, we compared the effects of hypothalamic gabazine injections on XII nerve activity before and after local microinjections of a dual orexin receptor antagonist, Almorexant (Brisbare-Roch et al. 2007), into the XII nucleus. Contrary to our expectation, Almorexant injected into the XII nucleus did not attenuate hypothalamic activation of XII motoneurons (Stettner and Kubin 2013). On the other hand, Almorexant injections placed into the same posterior hypothalamic site prior to gabazine injections delayed and attenuated the XII nerve and respiratory rate increases (as well as heart rate increases) (Stettner and Kubin 2013). This suggested that local, intrahypothalamic interactions mediated by orexin play an important, albeit only a partial, role in shaping of the response elicited by disinhibition of cells in the perifornical hypothalamus. A similar result was also obtained from the same animal model by others. Specifically, as in our study with local microinjections of Almorexant into the perifornical hypothalamus, systemic administrations of moderate doses of Almorexant (15 mg/kg) attenuated, but did not abolish, tachypneic and sympathoexcitatory effects of hypothalamic microinjections of bicuculline (Iigaya et al. 2012).
The following two points are worth deriving from the studies of the characteristic pattern of activation elicited by disinhibition of posterior hypothalamic neurons. First, there is to date no evidence that any component of the complex response elicited in this model can be entirely abolished by orexin antagonists regardless of whether they are administered locally or systemically. Rather, responses are either attenuated or unaffected. Second, local microinjections of the dual orexin receptor antagonist, Almorexant, into the perifornical region were sufficient to significantly attenuate both respiratory and cardiovascular effects of perifornical gabazine. Together, these findings suggest that the effects elicited by disinhibition of perifornical region neurons originate in orexin-containing and other neurons with overlapping projections and somewhat redundant functions. It also appears that orexin is an important excitatory peptide within the posterior lateral hypothalamus, and that its local role is to synchronize activation of orexin-containing and other local neurons who jointly ensure proper cardiorespiratory and behavioral activation, as needed by different environmental challenges. Accordingly, we would postulate that the perifornical hypothalamus may contain additional types of wake- and arousal-active neurons that interact with orexin neurons and generate complementary signals to those emanating from orexin neurons. A search for such neurons may prove to be a rewarding endeavor.
2.3 Association Between Orexin Dysfunction and OSA in Humans
OSA patients have reduced plasma levels of orexin (Busquets et al. 2004; Nishijima et al. 2003) which are then increased following treatment with nasal continuous positive airway pressure (nCPAP) (Sakurai et al. 2005). A plausible explanation of these findings is that the excessive sleepiness characteristically affecting OSA patients results in lower levels of orexin cell activity and, consequently, reduced plasma orexin levels. Once sleep is normalized by treatment with nCPAP, patients are less sleepy, more alert, and orexin cell activity levels rebounds. However, gene association studies also reveal a significant relationship between the genetic predisposition for narcolepsy-cataplexy and OSA. Specifically, a distinct polymorphism of prepro-orexin gene has been associated with OSA (Ahmed et al. 2012; Chen et al. 2012).
Interestingly, in contrast to findings in rats, narcolepsy-cataplexy patients with identified human leucocyte antigen (HLA)-DQB1*0602 allele that predisposes to narcolepsy-cataplexy do not exhibit reduced ventilatory sensitivity to hypercapnia. Instead, they have depressed ventilatory response to hypoxia (Han et al. 2010). The discrepancy between the impairment of hypoxic ventilatory response in humans versus hypercapnic ventilatory response in mice in orexin deficiency is consistent with a complex and indirect, rather than a strictly deterministic, impact of orexins on ventilatory regulation.
3 Endogenous Cardiovascular Effects of Orexins
There are reports of both increased and decreased arterial blood pressure in orexin-deficient rodents when compared to their wild-type controls. The picture is complicated because, under physiologic conditions, blood pressure exhibits predictable and clinically significant dips on transition from wakefulness to SWS and then a relative increase, as well as increased variability, on transition from SWS to REM sleep. This pattern also appears to be affected by orexin deficiency. On the other hand, it is well established that arterial hypertension elicited by stress depends on increased activation of orexin neurons. Similarly, the spontaneous neurogenic hypertension depends on hyperactivity of orexin nerons. In both situations, there is also evidence of possible upregulation of orexin receptors and increased orexinergic innervation at brainstem sites relevant for the control of arterial blood pressure.
3.1 Baseline Blood Pressure Levels in Orexin Deficiency
In contrast to the absence of any effect of genetic elimination of orexin on baseline ventilation, it has been initially reported that orexin KO mice have significantly lower baseline blood pressure than their wild-type counterparts (Kayaba et al. 2003). For the systolic blood pressure, the magnitude of the difference was about 25 mmHg in anesthetized animals, and about 15 mmHg in chronically instrumented, behaving mice. A similar difference was also reported for the orexin/ataxin-3 mice (Zhang et al. 2006). A subsequent study using the orexin/ataxin-3 rats which included a more detailed analysis of blood pressure and heart rate separately during different phases of the circadian cycle and during transitions between different sleep-wake states also reported that hypocretin neuron-ablated rats had reduced blood pressure during wakefulness and all sleep stages, whereas heart rate measurements did not reveal any differences. Notably, blood pressure changes accompanying transitions between sleep-wake state were small, less than 4 mmHg, and not different between the orexin/ataxin-3 rats and their wild type controls (Schwimmer et al. 2010).
However, data from a series of reports from another group were not concordant with the finding of a reduced blood pressure in orexin deficient rodents. Instead, both orexin KO mice and orexin/ataxin-3 mice were reported to have higher blood pressure during SWS and REM sleep than the control mice of the same genetic background, whereas during wakefulness blood pressure did not differ among the three strains (Bastianini et al. 2011). As a result, blood pressure decrease (dip) that typically occurs on transition from wakefulness to non-REM sleep was attenuated in the orexin-deficient animals. In another study, neither the average blood pressure levels nor the blood pressure dips with entry into non-REM sleep differed between the orexin/ataxin-3 mice and control animals (Lo Martire et al. 2012). A third study from the same group devoted to the analysis of dynamic fluctuations of blood pressure and heart rate in different sleep-wake states in orexin deficient mice also concluded that the absence of orexin or orexin-containing neurons imparts only small and subtle changes on the central neural control of baseline blood pressure and heart rate across wake-sleep states (Silvani et al. 2012).
Data from human narcolepsy-cataplexy patients revealed that daytime blood pressure levels were similar in patients and control subjects, but the patients had a non-dipping night time blood pressure pattern (Grimaldi et al. 2012). Circadian rhythms of heart rate and blood pressure did not differ between patients and control subjects but systolic blood pressure during night time REM sleep was elevated and the average heart rate was significantly higher in the patient group across the entire 24 h period. The authors noted that the nocturnal blood pressure and heart rate patterns could be different in patients due to the characteristic for narcolepsy-cataplexy increase of sleep fragmentation and increased prevalence of arousals-causing periodic limb movements. Ultimately, a blunted night time blood pressure decrease in the patient group was significantly associated only with periodic limb movements. In another study, no differences were detected in mean systolic blood pressure or heart rate within different sleep-wake states between narcolepsy-cataplexy patients and control subjects, and only subtle differences were found in indices of blood pressure and heart rate variability, suggesting altered vago-sympathetic control and reduced bararoceptor reflex sensitivity in narcolepsy-cataplexy (Silvani et al. 2013). Similar to these studies, in a study focused exclusively of the heart rate, narcolepsy-cataplexy patients had a reduced transient heart rate acceleration associated with arousals (Sorensen et al. 2013). Notably, heart rate response was more attenuated in the patients with directly verified reduced plasma orexin levels and patients with cataplexy when compared to narcoleptic patients who had normal orexin levels or patients without cataplexy. Thus, orexin deficiency was associated with a significant attenuation of dynamic heart rate changes but, contrary to the generalization offered by the authors of the study, there was no evidence for a tonic contribution of the orexin system to modulation of cardiovascular function at rest.
The overall impression from the studies of cardiovascular baseline parameters in orexin deficiency is that the absence of orexin effects, has little if any effects.
3.2 Role of Orexins in Spontaneous Arterial Hypertension
Two studies have recently reported that elevated blood pressure in a commonly used rodent model of spontaneous neurogenic hypertension, the spontaneousy hypertensive rat (SHR), is dependent on orexinergic hyperactivity (Lee et al. 2013; Li et al. 2013). In the study of Li et al. (2013), the dual orexin receptor antagonist, Almorexant (250–300 mg/kg in oral gavage) profoundly reduced the mean arterial blood pressure by over 40 mmHg during the dark (active) period and by nearly 30 mmHg during the light (rest) period, whereas in the control rat strain, the normotensive Wistar-Kyoto rat, the effects of Almorexant were not significant. In the study of Lee et al. (2013), intracerebroventricular administration of orexin-2 receptor antagonist similarly reduced blood pressure and reduced heart rate in SHR, but not in Wistar-Kyoto rats, and local microinjections of the same antagonist into the ventrolateral medulla had stronger hypotensive effect in SHR than in the control rats. In contrast, orexin-1 receptor antagonist had no effect. Additionally, both studies revealed various biochemical markers consistent with upregulation of orexinergic transmission in the rostral ventrolateral medulla.
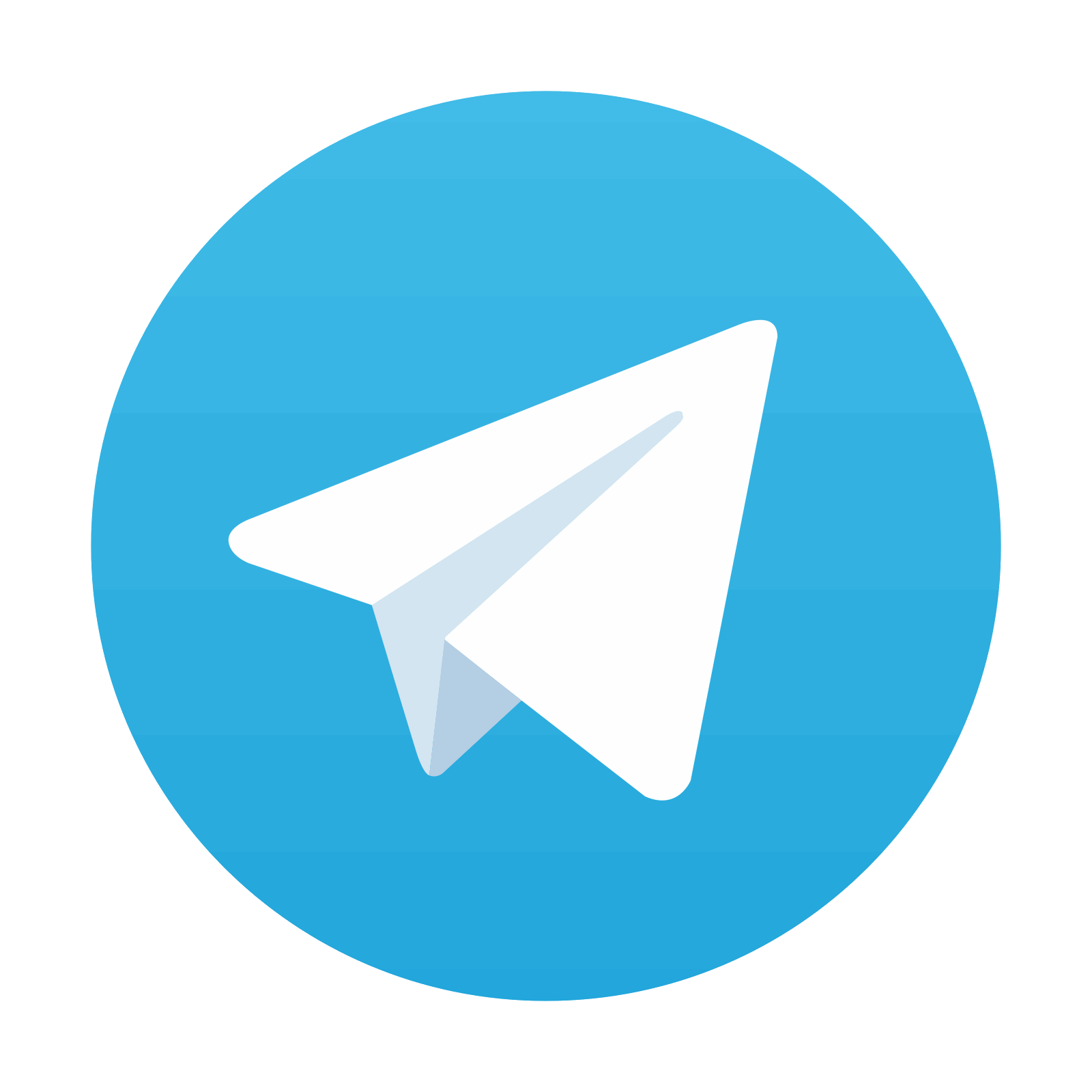
Stay updated, free articles. Join our Telegram channel

Full access? Get Clinical Tree
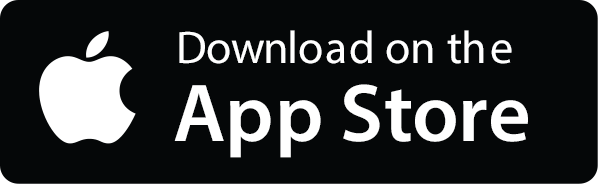
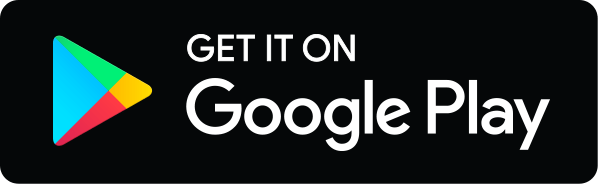