Touch
Active and Passive Touch Evoke Similar Responses in Mechanoreceptors
The Hand Has Four Types of Mechanoreceptors
Both Slowly and Rapidly Adapting Fibers Are Important for Grip Control
Tactile Information Is Processed in the Central Touch System
Cortical Receptive Fields Integrate Information from Neighboring Receptors
Neurons in the Somatosensory Cortex Are Organized into Functionally Specialized Columns
Touch Information Becomes Increasingly Abstract in Successive Central Synapses
Cognitive Touch Is Mediated by Neurons in the Secondary Somatosensory Cortex
Active Touch Engages Sensorimotor Circuits in the Posterior Parietal Cortex
Lesions in Somatosensory Areas of the Brain Produce Specific Tactile Deficits
IN THIS CHAPTER ON THE SENSE OF TOUCH, we focus on the hand because of its importance in the sensory appreciation of object properties and its role in skilled motor tasks. The hand is one of evolution’s great creations. The fine manipulative capacity provided by our fingers is possible because of their fine sensory capacity; if we lose tactile sensation in our fingers we lose manual dexterity.
When we become skilled in the use of a tool, such as a scalpel or a pair of scissors, we feel conditions at the working surface of the tool as though our fingers were there because two groups of mechanoreceptors monitor the vibrations and forces produced by those distant conditions. When we scan our fingers across a surface we feel its form and texture because another group of mechanoreceptors has high spatial and temporal acuity. A blind person uses this capacity to read Braille at a hundred words per minute. When we grip and manipulate an object we do so delicately, with only as much force as is required, because yet another group of mechanoreceptors continually monitors slip and adjusts our grip appropriately.
We are also able to recognize objects placed in the hand from touch alone. When we are handed a baseball we recognize it instantly without having to look at it because of its shape, size, weight, density, and texture. We do not have to think about the information provided by each finger to deduce that the object must be a baseball; the information flows to memory and instantly matches previously stored representations of baseballs. Even if we have never previously handled a baseball, we perceive it as a single object, not as a collection of discrete features. The somatosensory pathways of the brain have the daunting task of integrating information from thousands of sensors in each hand and transforming it to a form suitable for cognition.
Sensory information is extracted for the purpose of motor control as well as cognition, and different kinds of information are extracted for those purposes. We can, for example, shift our attention from the baseball’s shape to its location in the hand to readjust our grip for an effective throw. This selective attention to different aspects of the sensory information is brought about by cortical mechanisms.
Active and Passive Touch Evoke Similar Responses in Mechanoreceptors
Touch is defined as direct contact between two physical bodies. In neuroscience touch refers to the special sense by which contact with the body is perceived consciously. Touch can be active, as when you move your hand or some other part of the body against another surface, or passive, as when someone or something else touches you.
Active and passive modes of tactile stimulation excite the same population of receptors in the skin and evoke similar responses in afferent fibers. They differ somewhat in cognitive features that reflect attention and behavioral goals during the period of stimulation. Passive touch is used for naming objects or describing sensations; active touch is used when the hand manipulates objects. The sensory and motor components of touch are intimately connected anatomically in the brain and are important functionally in guiding motor behavior.
The distinction between active and passive touch is important clinically when patients have deficits in hand use. Motor deficits such as weakness, stiffness, or clumsiness may result from sensory loss, which is why passive sensory testing is important in the neurological examination (see Appendix B). Common neurological tests for touch include measurements of detection thresholds, vibration sense, two-point or texture discrimination, and the ability to recognize form through touch (stereognosis). The neural mechanisms underlying these tests are discussed in this chapter. Other common tests of somatosensory function—tendon reflexes, pinprick, and thermal tests—are discussed in other chapters.
Nearly all of these neurological tests involve passive touch; the clinician stimulates the body in a controlled and reproducible fashion. The tests measure the sensitivity and function of various receptors for touch. Deviations from the expected values may help diagnose the sensory deficits or lesions that underlie sensory dysfunction.
The Hand Has Four Types of Mechanoreceptors
The softness and compliance of the skin plays a major role in the sense of touch. When an object contacts the hand, the skin conforms to its contours, forming a mirror image of the object surface. The resultant displacement and indentation of the skin stretches the tissue, thereby stimulating the sensory endings of mechanoreceptors at or near the region of contact.
These receptors are highly sensitive and are continually active as we manipulate objects and explore the world with our hands. They provide information to the brain about the position of the stimulus on the skin, its shape and surface texture, the amount of force applied at the contact point, and how these features change over time when the hand or the object moves.
Tactile sensations in the human hand arise from four kinds of mechanoreceptors: Meissner corpuscles, Merkel cells, Pacinian corpuscles, and Ruffini endings (Figure 23–1). The sense of touch can be understood as the combined result of the information provided by these four systems acting in concert.
Figure 23-1 Four mechanoreceptors are responsible for the sense of touch. A cross section of the glabrous skin shows the principal receptors for touch. All of these receptors are innervated by large-diameter myelinated fibers. The Meissner corpuscles and Merkel cells lie in the superficial layers of the skin at the base of the epidermis, 0.5 to 1.0 mm below the skin surface. The Meissner corpuscles border the edges of each papillary ridge, whereas the Merkel cells form dense bands surrounding the sweat gland ducts along the center of the ridges. The RA1 and SA1 fibers that innervate these receptors branch at their terminals so that each fiber innervates several nearby receptor organs. The Pacinian and Ruffini corpuscles lie within the dermis (2–3 mm thick) and deeper tissues. The RA2 and SA2 fibers that innervate these receptors each innervate only one receptor organ. (RA1, rapidly adapting type 1; RA2, rapidly adapting type 2; SA1, slowly adapting type 1; SA2, slowly adapting type 2.)
Each receptor responds in a distinctive manner depending on its morphology, innervation pattern, and depth in the skin. Receptors are innervated by either slowly adapting or rapidly adapting axons. Slowly adapting (SA) fibers respond to steady skin indentation with a sustained discharge, whereas rapidly adapting (RA) fibers stop firing as soon as the indentation is stationary. Sustained mechanical sensations from the hand must accordingly arise from the SA fibers. The sensation of motion across the skin is signaled by RA fibers. The receptors are further subdivided into two types based on size and location in the skin; each type includes both rapidly and slowly adapting fibers.
Thus tactile sensation in the hand is mediated by four functional units: rapidly adapting type 1 (RA1), slowly adapting type 1 (SA1), rapidly adapting type 2 (RA2), and slowly adapting type 2 (SA2). Each unit consists of an afferent fiber, the fiber’s distal branches, and the receptor organ(s) that surround the axon terminals (Table 23–1).
Type 1 fibers terminate in clusters of small receptors in the superficial layers of the skin at the margin between the dermis and epidermis (Figure 23–1). RA1 fibers are the most numerous tactile afferents in the hand, reaching a density of approximately 2 per mm2 at the fingertip in man and monkey. The RA1 receptor organ, the Meissner corpuscle, is a globular, fluid-filled structure that encloses a set of flattened, lamellar cells originating from the myelin sheath (see Figure 21–6). The lamellae are coupled mechanically to the edge of the papillary ridge by collagen fibers, a relationship that confers fine mechanical sensitivity to frictional forces as the hand is moved across surfaces (Box 23–1). An RA1 axon typically innervates 10 to 20 Meissner corpuscles, integrating information from several adjacent papillary ridges. Each Meissner corpuscle is innervated by 2 to 5 RA1 axons (Figure 23–3A).
Figure 23-3 Innervation pattern of Meissner corpuscles and Merkel cells in hairy and glabrous skin.
A. A confocal transverse section of a papillary ridge in the human glabrous skin shows the innervation of mechanoreceptors in the fingertip. Meissner corpuscles are located just below the epidermis (blue) at the apex of the ridge, and are innervated by two or more rapidly adapting type 1 (RA1) fibers. The fibers lose their myelin sheaths (orange) when entering the receptor capsule, exposing broad terminal bulbs at which sensory transduction occurs (green). Individual slowly adapting type 1 (SA1) fibers innervate groups of Merkel cells clustered at the base of the intermediate ridge, providing localized signals of pressure applied to that ridge. Scale bar = 50 µm. (Adapted, with permission, from Nolano et al. 2003.)
B. A higher-magnification micrograph portrays antibody-labeled Merkel cells (red) innervated by an SA1 fiber (green). Each fiber extends multiple branches parallel to the surface of the skin that allow it to integrate tactile information from multiple receptor cells in a small zone of skin. The diameter of each Merkel cell is approximately 10 µm. (Adapted, with permission, from Snider 1998.)
SA1 fibers are also widely distributed in the skin, particularly in the fingertips. The SA1 receptor organs, the Merkel cells, consist of small epithelial cells that surround the terminal branches of an axon. Each Merkel cell encloses a semirigid structure that transmits compressive strain to the sensory nerve ending. Because there are synapse-like junctions between the Merkel cells and the SA1 axon terminals, it has been proposed that the mechanosensitive ion channels reside in the Merkel cells rather than in the nerve endings. Merkel cells are densely clustered in the center of each papillary ridge in glabrous skin (Figure 23–3A), placing them in an excellent position to detect deformation of the overlying skin, either from pressure above or lateral stretch. In hairy skin Merkel cells are localized in small clusters called touch domes (Figure 23–3B).
Box 23–1 Fingerprint Structure Enhances Touch Sensitivity in the Hand
The histological structure of glabrous skin—the smooth, hairless skin of the palm and fingertips—plays a crucial role in the hand’s sensitivity to touch. The fingerprints are formed by a regular array of parallel ridges in the epidermis, the papillary ridges (Figure 23–2).
Figure 23-2 The skin of the finger.
A. Scanning electron micrograph of the fingerprints in the human index finger. The glabrous skin of the hand is structured as arrays of papillary ridges and intervening sulci (limiting ridges) that recur at regular intervals. Globules of sweat exude from ducts at the center of the papillary ridges, forming a grid-like pattern along each ridge. The Merkel cells are located in dense clusters along the center of the papillary ridges between the ducts (see Figure 23–1). (Adapted, with permission, from Quilliam 1978.)
B. Histological section of the glabrous skin cut parallel to the skin surface. The cholinesterase-stained Meissner corpuscles form regularly spaced chains along both sides of each papillary ridge. Thus Meissner corpuscles and Merkel cells form alternating bands of RA1 and slowly adapting type 1 (SA1) touch receptors that span each fingerprint ridge. (Adapted, with permission, from Bolanowski and Pawson 2003.)
Each ridge is bordered by epidermal folds—the limiting ridges—that are visible as thin lines on the fingers and palm border. The limiting ridges increase the stiffness and rigidity of the skin, protecting it from damage when contacting objects or when walking barefooted.
The fingerprints give the glabrous skin a corrugated, rough structure that increases friction, allowing us to grasp objects without slippage. Frictional forces are augmented further when these ridges contact the textured surfaces of objects. Smooth surfaces slide easily underneath the fingers and thus require greater grip force to maintain stability in the hand; the screw caps on bottles are often ridged to make them easy to turn. Frictional forces between the limiting ridges and objects also amplify surface features when we palpate them, allowing us to detect small irregularities such as the grain of wood.
The regular spacing of the papillary ridges—and the precise localization of specific receptors within this grid—allow us to repeatedly scan surfaces with back and forth hand movements while preserving a constant spatial alignment of adjacent surface features.
Type 2 fibers innervate the skin sparsely and terminate in single large receptors in the deeper layers of the dermis or in the subcutaneous tissue (Figure 23–1). The receptors are larger and less numerous than the receptor organs of the type 1 fibers. The large size of these receptors allows them to sense mechanical displacement at some distance from the sensory nerve endings.
The RA2 fibers terminate in Pacinian corpuscles located in the subcutaneous tissue (Figure 23–1). Each RA2 axon terminates without branching in a single Pacinian corpuscle, and each Pacinian corpuscle receives but a single RA2 axon. Pacinian corpuscles are large onion-like structures in which successive layers of connective tissue are separated by fluid-filled spaces. These layers surround the unmyelinated RA2 ending and its myelinated axon up to one or more nodes of Ranvier. The capsule amplifies high-frequency vibration, a role that is important for tool use. Estimates of the number of Pacinian corpuscles in the human hand range from 2,400 in the young to 300 in the elderly.
The SA2 fibers innervate Ruffini endings concentrated at the finger and wrist joints and along the skin folds in the palm; they are relatively rare in the fingertips. The Ruffini endings are elongated fusiform structures that enclose collagen fibrils extending from the subcutaneous tissue to folds in the skin at the joints, in the palm, or in the fingernails. The SA2 nerve endings are intertwined between the collagen fibers in the capsule, and are excited by stimuli that stretch the receptor along its long axis.
Receptive Fields Define the Zone of Tactile Sensitivity
Individual mechanoreceptor fibers convey information from a limited area of skin called the receptive field. Tactile receptive fields have been determined in the human hand using microneurography.
Åke Vallbo and Roland Johansson inserted micro-electrodes through the skin into the median or ulnar nerves in the human hand and recorded the responses of individual afferent fibers. They found that in humans, as in other primate species, there are important differences between touch receptors, both in their physiological responses and in the structure of their receptive fields.
Type 1 fibers have small, highly localized receptive fields with multiple spots of high sensitivity that reflect the branching patterns of their axons in the skin (Figure 23–4). Receptive fields on the fingertips are the smallest on the body, averaging 11 mm2 for SA1 fibers and approximately 25 mm2 for RA1 fibers. The fields are small because of the high density of receptors in the fingertips. Receptive fields become progressively larger on the proximal phalanges and the palm, consistent with the lower density of mechanoreceptors in these regions.
Figure 23-4 Receptive fields in the human hand are smallest at the fingertips. Each colored area on the hands indicates the receptive field of an individual sensory nerve fiber. (Adapted, with permission, from Johansson and Vallbo 1983.)
A–B. The receptive fields of receptors in the superficial layers of skin encompass spot-like patches of skin. Those of receptors in the deep layers extend across wide regions of skin (light shading), but responses are strongest in the skin directly over the receptor (dark spots). The arrows indicate the directions of skin stretch that activate SA2 fibers.
C. Pressure sensitivity throughout the receptive field is shown as a contour map. The most sensitive regions are indicated in red and the least sensitive areas in pale pink. The receptive field of an RA1 fiber (above) has many points of high sensitivity, marking the positions of the group of Meissner corpuscles innervated by the fiber. The receptive field of an RA2 fiber (below) has a single point of maximum sensitivity overlying the Pacinian corpuscle. The receptive field of SA1 fibers is similar to that of RA1 fibers. Likewise, the receptive field of SA2 fibers resembles that of RA2 fibers.
In contrast, type 2 fibers innervating the deep layers of skin are connected to only a single Pacinian corpuscle or Ruffini ending. Because these receptors are large they collect information from a broader area of skin. Their receptive fields contain a single “hot spot” where sensitivity to touch is greatest; this point is located directly above the receptor (Figure 23–4).
The receptive fields of type 1 fibers are significantly smaller than most objects that contact the hand. Thus RA1 and SA1 fibers detect small pieces of an object, signaling the properties of only a portion of its surface. As in the visual system, the spatial features of objects are distributed across a population of stimulated receptors with responses that are integrated in the brain to form a unified percept.
Two-Point Discrimination Tests Measure Texture Perception
The ability of humans to resolve spatial details of textured surfaces depends on which part of the body is contacted. Tactile acuity is highest on the fingertips and the lips, where receptive fields are smallest (Figure 23–5A). The separation that defines performance midway between chance and perfect discrimination, the threshold for spatial acuity, is approximately 1 mm on the fingertips of young adults; by the sixth or seventh decade of life it declines on average to approximately 2 mm. When we grasp an object we can discriminate features of its surface separated by as little as 0.5 mm. Humans are able to distinguish horizontal from vertical orientation of gratings with remarkably narrow spacing of the ridges (Figure 23–5B).
Figure 23-5 Tactile acuity in the human hand is highest on the fingertip.
A. The two-point threshold measures the minimum distance at which two stimuli are resolved as distinct. This distance varies for different body regions; it is approximately 2 mm on the fingers, but as much as 10 mm on the palm and 40 mm on the arm, thigh, and back. The two-point perceptual thresholds highlighted in pink match the diameters of the receptive fields of receptors in the pink zones on the body. The greatest discriminative capacity is afforded in the fingertips, lips, and tongue, which have the smallest receptive fields. (Adapted, with permission, from Weinstein 1968.)
B. Spatial acuity is measured in psychophysical experiments by having a blindfolded subject touch a variety of textured surfaces. As shown here, the subject is asked to determine whether the surface of a wheel is smooth or contains a gap, whether the ridges of a grating are oriented across the finger or parallel to its long axis, or which letters appear on raised type used in letterpress printing. The tactile acuity threshold is defined as the groove width, ridge width, or font size that yields 75% correct performance (detectable midway between chance and perfect accuracy). The threshold spacing on the human fingertip is 1.0 mm in each of these tests. (Adapted, with permission, from Johnson and Phillips 1981.)
Tactile acuity is slightly greater in women than in men and varies between fingers but not between hands. The distal pad of the index finger has the keenest sensitivity; spatial acuity declines progressively from the index to the little finger and declines rapidly at locations proximal to the distal finger pads. Tactile spatial resolution is 50% poorer at the distal pad of the little finger and six to eight times coarser on the palm.
Tactile acuity on proximal parts of the body decreases in parallel with the growing size of receptive fields of SA1 and RA1 fibers (Figure 23–5A). When a pair of probes is spaced several millimeters apart on the hand, each of them is perceived as a distinct point because they produce separate dimples in the skin and stimulate nonoverlapping populations of SA1 and RA1 receptors. As the probes are moved closer together, the two sensations become blurred because both probes are contained within the same receptive field. The spatial interactions between tactile stimuli form the basis of neurological tests of two-point discrimination and texture recognition.
Blind individuals use the fine spatial sensitivity of SA1 and RA1 fibers to read Braille. The Braille alphabet represents letters as simple dot patterns that are easy to distinguish by touch (Figure 23–6). A blind person reads Braille by moving the fingers over the dot patterns. This hand movement enhances the sensations produced by the dots.
Figure 23-6 Responses of touch receptors to Braille dots scanned by the fingers. The Braille symbols for the letters A through R were mounted on a drum that was repeatedly rotated against the fingertip of a human subject. Following each revolution the drum was shifted upward so that another portion of the symbols was scanned across the finger. Micro-electrodes placed in the median nerve of this subject recorded the responses of the mechanoreceptive fibers innervating the fingertip. The action potentials discharged by the nerve fibers as the Braille symbols were moved over the receptive field are represented in these records by small dots; each horizontal row of dots represents the responses of the fiber to a single revolution of the drum. The SA1 receptors register the sharpest image of the Braille symbols, representing each Braille dot with a series of action potentials and falling silent when the spaces between Braille symbols provide no stimulation. RA1 receptors provide a blurred image of the Braille symbols because their receptive fields are larger, but the individual dot patterns are still recognizable. Neither RA2 nor SA2 receptors are able to encode the Braille patterns because their receptive fields are larger than the dot spacing. The high firing rate of the RA2 fibers reflects the keen sensitivity of Pacinian corpuscles to vibration. (RA1, rapidly adapting type 1; RA2, rapidly adapting type 2; SA1, slowly adapting type 1; SA2, slowly adapting type 2.) (Reproduced, with permission, from Phillips, Johansson, and Johnson 1992.)
Because the Braille dots are spaced approximately 3 mm apart, a distance greater than the receptive field diameter of an SA1 fiber, each dot stimulates a different set of SA1 fibers. An SA1 fiber fires a burst of action potentials as a dot enters its receptive field and is silent once the dot leaves the field (Figure 23–6). Specific combinations of SA1 fibers that fire synchronously signal the spatial arrangement of the Braille dots. RA1 fibers also discriminate the dot patterns, enhancing the signals provided by SA1 fibers.
Slowly Adapting Fibers Detect Object Pressure and Form
The most important functional feature of the slowly adapting fibers (SA1 and SA2) is their ability to signal skin deformation and pressure. The sensitivity of an SA1 receptor to edges, corners, points, and curvature provides information about object shape, size, surface texture, and compliance. We perceive an object as hard or rigid if it indents the skin, and soft if the skin surface instead deforms the object.
Paradoxically, as an object’s diameter increases, the responses of individual SA1 fibers become weaker and the sensation less distinct. For example, the tip of a pencil pressed 1 mm into the skin feels sharp, unpleasant, and highly localized at the contact point, whereas a 1 mm indentation by the eraser feels blunt and broad. The weakest sensation is evoked by a flat surface pressed against the finger pad (Figure 23–7).
Figure 23-7 Slowly adapting type 1 (SA1) fibers encode the shape and size of objects touching the hand.
A. The area of contact on the skin determines the firing rate and total number of SA1 fibers stimulated. The pink region on the fingertip shows the spread of excitation when probes of different diameters are pressed upon the skin with constant force. The intensity of color is proportional to the firing rates of the stimulated receptors. A small-diameter, sharply pointed probe (left) activates a small population of SA1 receptors. The activated fibers fire intensely because all of the force is concentrated in a small area. A medium-size probe (middle) excites more receptors, but the spread of the force reduces peak firing rates. The probe does not feel as sharp as the small-diameter probe. A large round probe (right) stimulates a large population of SA1 receptors spread across the width of the finger. These fibers fire at low rates because the force is spread over a wide area of skin. The sensation of pressure is diffuse. (Adapted, with permission, from Goodwin, Browning, and Wheat 1995.)
B. The firing rate of an individual SA1 fiber is determined by the probe diameter. When a probe first contacts the skin, the SA1 response is strong regardless of probe diameter. During steady pressure the firing rate is proportional to the curvature of each probe. The highest firing rates are evoked by the smallest probe, while the weakest responses are produced by flat surfaces and gently rounded (large diameter) probes. The tip’s curvature is expressed as the inverse of its spherical radius. (Adapted, with permission, from Srinivasan and LaMotte 1991.)
To understand why these objects evoke different sensations, we need to consider the physical events that occur when the skin is touched. When a pencil tip is pressed against the skin it dimples the surface at the contact point and forms a shallow, sloped basin in the surrounding region (approximately 4 mm in radius). Although the indentation force is concentrated in the center, the surrounding region is also perturbed by local stretch, called tensile strain. SA1 receptors at both the center and the surrounding “hillsides” of skin are stimulated, firing spike trains proportional to the degree of local stretch.
If a second probe is pressed close to the first one, more SA1 fibers are stimulated but the neural response of each fiber is reduced because the force needed to displace the skin is shared between the two probes. Ken Johnson and his colleagues have shown that as more probes are added within the receptive field, the response intensity of each fiber becomes progressively weaker because the displacement forces on the skin are distributed across the entire contact zone. Thus the skin mechanics results in a case of “less is more.” Individual SA1 fibers respond more vigorously to a small object than to a large one because the force needed to indent the skin is concentrated at a small contact point. In this manner each SA1 fiber integrates the local skin indentation profile within its receptive field.
The sensitivity of SA1 receptors to local stretch of the skin also enables them to detect edges, the places where an object’s curvature changes abruptly. SA1 firing rates are many times greater when a finger touches an edge than when it touches a flat surface.
The indentation force of a flat or gently curved surface is distributed symmetrically within the central contact zone, whereas the force applied by an object boundary displaces the skin asymmetrically, beyond the edge as well as at the edge. This asymmetric distribution of force produces enhanced responses from receptive fields located along the edges of an object. As edges are often perceived as sharp, we tend to grasp objects on flat or gently curved surfaces rather than by their edges.
The SA2 fibers that innervate Ruffini endings respond more vigorously to stretch of the skin than to indentation because the receptors are located along the palmar folds or at the finger joints. The SA2 fibers therefore provide information about the shape of large objects grasped with the entire hand, the “power grasp” in which all five fingers press an object against the palm. They also provide information about hand shape and finger movements when the hand is empty. If the fingers are fully extended and abducted we feel the stretch in the palm and proximal phalanges as the glabrous skin is flattened. Similarly, if the fingers are fully flexed, forming a fist, we feel the stretch of the skin on the back of the hand, particularly over the metacarpal-phalangeal and proximal interphalangeal joints.
The SA2 system may play a central role in stereognosis—the recognition of three-dimensional objects using touch only—as well as other perceptual tasks in which skin stretch is a major cue. Benoni Edin has shown that SA2 innervation in the hairy skin plays a substantial role in the perception of hand shape and finger position. The SA2 fibers aid the perception of finger joint angle by detecting skin stretch around the knuckles. The Ruffini endings near these joints are aligned such that different groups of receptors are stimulated as the fingers move in specific directions (Figure 23–4A). In this manner the SA2 system provides a neural representation of skin stretch over the entire hand, a proprioceptive rather than exteroceptive function.
Rapidly Adapting Fibers Detect Motion and Vibration
The RA1 receptor organ, the Meissner corpuscle, detects events that produce low-frequency, low-amplitude skin motion. This includes hand motion over the surface of objects, the detection of microscopic surface features, and low-frequency vibration. RA1 fibers contribute to detection of Braille patterns because they sense the change in skin indentation as individual dots pass over their receptive fields (Figure 23–6). They can detect irregularities and bumps as small as 10 μm. We use the sensitivity of RA1 fibers to motion to adjust grip force when we grasp an object.
The RA2 receptor, the Pacinian corpuscle, is the most sensitive mechanoreceptor in the somatosensory system. It is exquisitely responsive to high-frequency (30–500 Hz) vibratory stimuli, and can detect vibration of 250 Hz in the nanometer range (Figure 23–8). The buzzing sensation experienced when a tuning fork is pressed against the skin in a neurological examination is mediated by the synchronized firing of RA2 units. It is a useful measurement of dynamic sensitivity to touch, particularly in cases of localized nerve damage.
Figure 23-8 Rapidly adapting type 2 (RA2) fibers encode vibration. Vibration is the sensation produced by sinusoidal stimulation of the skin, as by the hum of an electric motor, the strings of a musical instrument, or the tuning fork used in the neurological examination.
A. 1. The Pacinian corpuscle consists of concentric, fluid-filled lamellae of connective tissue that encapsulate the terminal of an RA2 fiber. This structure is uniquely suited to the detection of motion. Sensory transduction in the RA2 fiber occurs in stretch-sensitive cation channels linked to the inner lamellae of the capsule. 2. When steady pressure is applied to the skin the RA2 fiber fires a burst at the start and end of stimulation. In response to sinusoidal stimulation (vibration) the fiber fires at regular intervals such that each action potential signals one cycle of the stimulus. Our perception of vibration as a rhythmically repeating event results from the simultaneous activation of many RA2 units, which fire in synchrony.
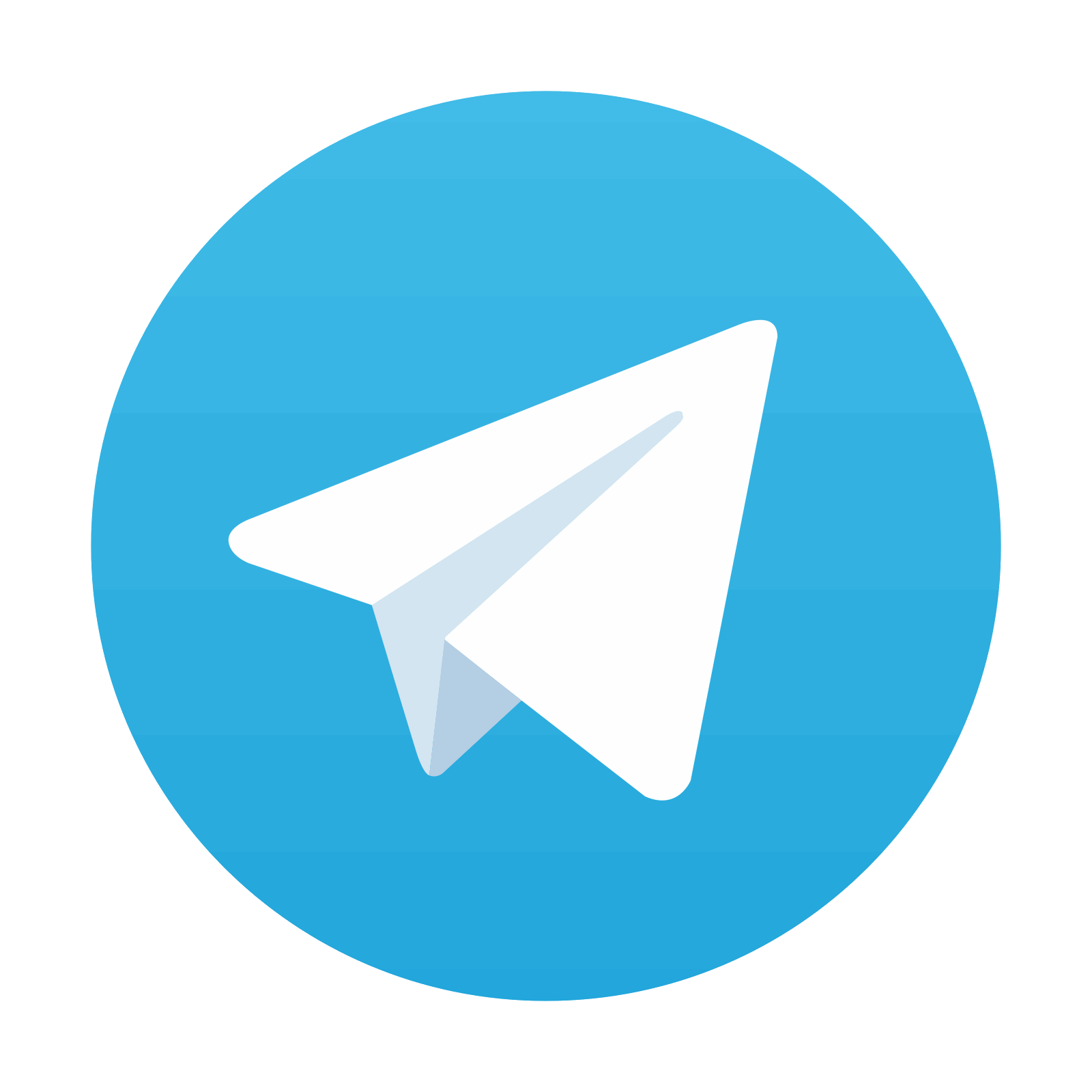
Stay updated, free articles. Join our Telegram channel

Full access? Get Clinical Tree
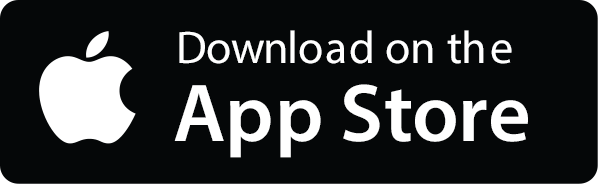
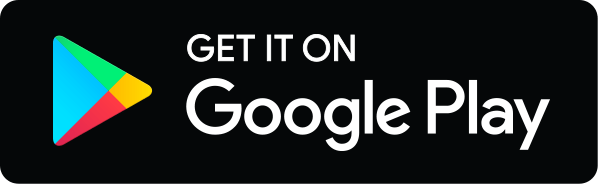