Chapter 13 Treatment of dystonia
Despite the paucity of knowledge about the cause and pathogenesis of dystonic disorders, the symptomatic treatment of dystonia has markedly improved, particularly since the introduction of botulinum toxin. In most cases of dystonia, the treatment is merely symptomatic, designed to improve posture and function and to relieve associated pain. In rare patients, however, dystonia can be so severe that it can produce not only abnormal postures and disabling dystonic movements, sometimes compromising respiration, but also muscle breakdown and life-threatening hyperthermia, rhabdomyolysis, and myoglobinuria. In such cases of dystonic storm or status dystonicus, proper therapeutic intervention can be life-saving (Jankovic and Penn, 1982; Vaamonde et al., 1994; Manji et al., 1998; Dalvi et al., 1998; Jankovic, 2006, 2009a).
The assessment of various therapeutic interventions in dystonia is problematic for the following reasons: (1) Dystonia and its effects on function are difficult to quantitate; therefore, most trials utilize crude clinical rating scales, many of which have not been properly evaluated or validated; (2) dystonia is a syndrome with different etiologies, anatomic distributions, and heterogeneous clinical manifestations producing variable disability; (3) some patients, perhaps up to 15%, may have spontaneous, albeit transient, remissions; (4) many studies have used dosages that may have been insufficient or too short a duration to provide benefit; (5) the vast majority of therapeutic trials in dystonia are not double-blind, placebo-controlled; and (6) most studies, even those that have been otherwise well designed and controlled, have utilized small sample sizes, which makes the results difficult to interpret, particularly in view of a large placebo effect demonstrated in dystonia (Lindeboom et al., 1996). A variety of instruments have been used to assess the response in patients with cervical dystonia; the most frequently used is the Toronto Western Spasmodic Torticollis Rating Scale (TWSTRS) (Consky and Lang, 1994; Comella et al., 1997). TWSTRS (range: 0–87) consists of three subscales: severity (range: 0–35), disability (range: 0–23), and pain (range: 0–20). In addition, visual analog scale, global assessment of change, and pain analog assessments have been used in various clinical trials. Various scales, such as the Fahn–Marsden scale and the Unified Dystonia Rating Scale, have also been used to assess patients with generalized dystonia (Burke et al., 1985a; Ondo et al., 1998; Comella et al., 2003). Another scale designed to capture the burden of dystonia on patients is the Cervical Dystonia Impact Profile scale (Cano et al., 2004). The Jankovic Rating Scale was initially used in the original study of botulinum toxin (BTX) in patients with cranial dystonia which led to the approval of BTX by the Food and Drug Administration (Jankovic and Orman, 1987) and in subsequent studies (Roggenkamper et al., 2006) to assess the severity and frequency of involuntary eyelid contractions in patients with blepharospasm (Jankovic et al., 2009). Subsequent studies have refined the clinical rating scales and included quality-of-life scales (Lindeboom et al., 1996). Health-related quality-of-life instruments, such as the Craniocervical Dystonia Questionnaire (CDQ-24), are increasingly used to assess the function and the impact of treatment in patients with cranial, cervical, and other dystonias on activities of daily living and other measures of quality of life (Muller et al., 2004; Reimer et al., 2005; Hall et al., 2006).
The selection of a particular choice of therapy is guided largely by personal clinical experience and by empirical trials (Greene et al., 1988; Greene, 1995; Jankovic, 1997; Brin, 1998; Jankovic, 2004a, 2006; Albanese et al., 2006; Tinter and Jankovic, 2007) (Table 13.1). An evidence-based review concluded that except for BTX in cervical dystonia and high-dose trihexyphenidyl in young patients with segmental and generalized dystonia (level A, class I–II), none of the methods of pharmacologic intervention have been confirmed as being effective according to evidence-based criteria (Balash and Giladi, 2004). The patient’s age, the anatomic distribution of dystonia, and the potential risk of adverse effects are also important determinants of choice of therapy. The identification of a specific cause of dystonia, such as drug-induced dystonias or Wilson disease (Svetel et al., 2001), may lead to a treatment that is targeted to the particular etiology. It is therefore prudent to search for identifiable causes of dystonia, particularly when some atypical features are present. The diagnostic approach to patients with dystonia is based on being aware of the multiple causes of torsion dystonia, which was covered in Chapter 12 and in other reviews (Jankovic, 2007).
Table 13.1 Medical therapy of dystonia
Physical and supportive therapy
Before reviewing pharmacologic and surgical therapy of dystonia, it is important to emphasize the role of patient education and supportive care, which are integral components of a comprehensive approach to patients with dystonia. Various paramedical treatments reported to be useful in patients with primary dystonia have been systematically reviewed (Delnooz et al., 2009). Physical therapy and well-fitted braces are designed primarily to improve posture and to prevent contractures. Although braces are often poorly tolerated, particularly by children, they may be used in some cases as a substitute for a sensory trick. For example, some patients with cervical dystonia are able to construct neck–head braces that seem to provide sensory input by touching certain portions of the neck or head in a fashion similar to the patient’s own sensory trick, thus enabling the patient to maintain a desirable head position. Various hand devices have been developed in an attempt to help patients with writer’s cramp to use their hands more effectively and comfortably (Ranawaya and Lang, 1991; Tas et al., 2001). In one small study of five professional musicians with focal dystonia, Candia and colleagues (1999) reported success with immobilization by splints of one or more of the digits other than the dystonic finger followed by intensive repetitive exercises of the dystonic finger. It is not clear, however, whether this therapy provides lasting benefits. In one study of eight patients with idiopathic occupational focal dystonia of the upper limb, immobilization with a splint for 4–5 weeks resulted in a significant improvement at a 24-week follow-up visit, based on the Arm Dystonia Disability Scale (0 = normal; 3 = marked difficulty in playing) and the Tubiana and Champagne Score (0 = unable to play; 5 = returns to concert performances); the improvement was considered marked in four and moderate in three, but the initial improvement disappeared in one (Priori et al., 2001). The splint was applied for 24 hours every day except for 10 minutes once a week when it was removed for brief local hygiene. Immediately on full removal of the splint, all patients reported marked clumsiness and weakness, which resolved in 4 weeks. There was also some local subcutaneous and joint edema and pain in the immobilized joint, and nail growth stopped; none of the patients developed contractures. While the mechanisms of action of immobilization are unknown, the authors have postulated that removing all motor and sensory input to a limb might allow the cortical map to “reset” to the previous normal topography. One major concern about immobilization of a limb, particularly a dystonic limb, is that such immobilization can actually increase the risk of exacerbating or even precipitating dystonia, as has been well demonstrated in dystonia following casting or other peripheral causes of dystonia (Jankovic, 2001a). In one study of 21 patients with writer’s cramp, after 4 weeks of immbolization, “retraining” for 8 weeks using drawing and writing exercises was associated with significant improvement relative to baseline as assessed by the Writer’s Cramp Rating Scale (Zeuner et al., 2008). A variation of the immobilization therapy, constraint-induced movement therapy, has been used successfully in rehabilitation of patients after stroke and other brain insults, and the observed benefit has been attributed to cortical reorganization (Taub et al., 1999; Levy et al., 2001; Taub and Morris, 2001; Taub et al., 2002; Wolf et al., 2002).
Another technique, using a repetitive task during regional anesthesia of a weak arm in patients following stroke, was also associated with improved hand function (Muellbacher et al., 2002). Some patients find various muscle relaxation techniques and sensory feedback therapy useful adjuncts to medical or surgical treatments. Since some patients with dystonia have impaired sensory perception, it has been postulated that sensory training may relieve dystonia. In a study of 10 patients with focal hand dystonia, Zeuner and colleagues (2002) showed that reading Braille for 30–60 minutes daily for 8 weeks improved spatial acuity and dystonia that was sustained for up to 1 year in some patients (Zeuner and Hallett, 2003). Sensory training to restore sensory representation of the hand along with mirror imagery and mental practice techniques has also been reported to be useful in the treatment of focal hand dystonia (Byl and McKenzie, 2000; Byl et al., 2003).
Siebner and colleagues (1999) showed that using repetitive transcranial magnetic stimulation (rTMS) delivered at low frequencies (≤1 Hz) for 20 minutes can temporarily (8 of 16 patients reported improvement that lasted longer than 3 hours) improve handwriting impaired by dystonic writer’s cramp, presumably by increasing inhibition (and thus reducing excitability) of the underlying cortex. Despite some encouraging results from open-label pilot studies it remains to be seen whether rTMS or transcranial direct current stimulation (tDCS) will enter the mainstream of therapeutics of dystonia (Wu et al., 2008).
Other stimulation techniques are also being studied in patients with dystonia. Long-term neck muscle vibration of the contracting muscle might have a therapeutic value in patients with cervical dystonia. This is suggested by transient (minutes) improvement in head position in one patient who was treated for 15 minutes with muscle vibration (Karnath et al., 2000). Transcutaneous electrical stimulation has been found to improve dystonic writer’s cramp for at least 3 weeks in a double-blind, placebo-controlled trial (Tinazzi et al., 2005). Also, acoustic and galvanic vestibular stimulation was reported beneficial in one patient with cervical dystonia (Rosengren and Colebatch, 2006). Such observation is consistent with the notion that proprioceptive sensory input affects cervical dystonia.
Dopaminergic therapy
Pharmacologic treatment of dystonia is based largely on an empirical rationale rather than a scientific one. Unlike Parkinson disease, in which therapy with levodopa replacement is based on the finding of depletion of dopamine in the brains of parkinsonian animals and humans, our knowledge of biochemical alterations in idiopathic dystonia is very limited. One exception is dopa-responsive dystonia (DRD), in which the biochemical and genetic mechanisms have been elucidated by molecular DNA and biochemical studies in patients (Ichinose et al., 1994) and by studies of postmortem brains (Furukawa et al., 1999). Decreased neuromelanin in the substantia nigra with otherwise normal nigral cell count and morphology and normal tyrosine hydroxylase immunoreactivity were found in one brain of a patient with classic DRD (Rajput et al., 1994). There was a marked reduction in dopamine in the substantia nigra and the striatum. These findings suggested that in DRD, the primary abnormality was a defect in dopamine synthesis. This proposal is supported by the finding of a mutation in the GTP cyclohydrolase I gene on chromosome 14q that indirectly regulates the production of tetrahydrobiopterin, a cofactor for tyrosine hydroxylase, the rate-limiting enzyme in the synthesis of dopamine (Ichinose et al., 1994; Tanaka et al., 1995; Steinberger et al., 2000). Another form of DRD has been described in four cases belonging to two unrelated families of dopa-responsive cervical dystonia, presenting between 9 and 15 years of age and similar to classic DRD manifesting diurnal variation but no levodopa-induced dyskinesia (Schneider et al., 2006).
DRD usually presents in childhood with dystonia, mild parkinsonian features, and pseudopyramidal signs (hypertonicity and hyperreflexia) predominantly involving the legs. Many patients have a family history of dystonia or Parkinson disease. At least half of the patients have diurnal fluctuations, with marked progression of their symptoms toward the end of the day and a relief after sleep. Many patients with this form of dystonia are initially misdiagnosed as having cerebral palsy. Some patients with DRD are not diagnosed until adulthood, and family members of patients with typical DRD may present with adult-onset levodopa-responsive parkinsonism (Harwood et al., 1994). The take-home message from these reports is that a therapeutic trial of levodopa should be considered in all patients with childhood-onset dystonia, whether they have classic features of DRD or not.
Most patients with DRD improve dramatically even with small doses of levodopa (100 mg of levodopa with 25 mg of decarboxylase inhibitor), but some might require doses of levodopa as high as 1000 mg per day. In contrast to patients with juvenile Parkinson disease (Ishikawa and Miyatake, 1995), DRD patients usually do not develop levodopa-induced fluctuations or dyskinesias. If no clinically evident improvement is noted after 3 months of therapy, the diagnosis of DRD is probably an error, and levodopa can be discontinued. In addition to levodopa, patients with DRD also improve with dopamine agonists, anticholinergic drugs, and carbamazepine (Nygaard et al., 1991). In contrast to patients with DRD, patients with idiopathic or other types of dystonia rarely improve with dopaminergic therapy (Lang, 1988). While dopaminergic therapy is remarkably effective in treating DRD, this strategy is not useful in the treatment of primary dystonia. Apomorphine, however, may ameliorate dystonia, perhaps by decreasing dopamine as well as serotonin release (Zuddas and Cianchetti, 1996).
Antidopaminergic therapy
Although dopamine receptor-blocking drugs were used extensively in the past, most clinical trials have produced mixed results with these drugs. Because of the poor response and the possibility of undesirable side effects, particularly sedation, parkinsonism, and tardive dyskinesia, the use of these drugs in the treatment of dystonia should be discouraged (Jankovic, 1995b). Clozapine, an atypical neuroleptic, has been reported in a small open trial to be moderately effective in the treatment of segmental and generalized dystonia, but its usefulness was limited by potential side effects (Karp et al., 1999). Although antidopaminergic drugs have been reported to be beneficial in the treatment of dystonia, the potential clinical benefit is usually limited by the development of side effects. Dopamine-depleting drugs, however, such as tetrabenazine, have been found useful in some patients with dystonia, particularly those with tardive dystonia (Jankovic and Orman, 1988; Jankovic and Beach, 1997). Tetrabenazine, a vesicular monoamine transporter 2 (VMAT2) inhibitor, has the advantage over other antidopaminergic drugs in that it does not cause tardive dyskinesia, although it may cause transient acute dystonic reaction (Burke et al., 1985b; Jankovic and Beach, 1997; Kenney and Jankovic, 2006). Tetrabenazine is not readily available in the United States, but it is dispensed by prescription under the trade name Nitoman or Xenazine 25 in other countries, including the United Kingdom. It is possible that some of the new atypical neuroleptic drugs will be useful not only as antipsychotics but also in the treatment of hyperkinetic movement disorders. Risperidone, a D2 dopamine receptor-blocking drug with a high affinity for 5-HT2 receptors, has been reported to be useful in a 4-week trial of five patients with various forms of dystonia (Zuddas and Cianchetti, 1996). Clozapine, a D4 dopamine receptor blocker with relatively low affinity for the D2 receptors and high affinity for the 5-HT2A receptors, has been reported to ameliorate the symptoms of tardive dystonia (Trugman et al., 1994). The treatment of tardive dystonia and other tardive syndromes is discussed in Chapter 19 and in other reviews (Jankovic, 1995b).
Anticholinergic therapy
High-dosage anticholinergic medications, such as trihexyphenidyl, for dystonia were introduced by Fahn (1983) and confirmed by several groups (Marsden et al., 1984; Greene et al., 1988; Jabbari et al., 1989; Hoon et al., 2001), including a double-blind study (Burke et al., 1986). Although helpful in all types of dystonias, the supremacy of botulinum toxin therapy for focal dystonias has relegated anticholinergic therapy to be most useful in the treatment of generalized and segmental dystonia. In the experience of Greene and colleagues (1988), patients with blepharospasm, generalized dystonia, tonic (in contrast to clonic) dystonia, and onset of dystonia at age younger than 19 years seemed to respond better to anticholinergic drugs than other subgroups, but this difference did not reach statistical significance. Except for short duration of symptoms before onset of therapy, there was no other variable, such as gender or severity, that reliably predicted a favorable response. Treating patients within the first 5 years of disease onset was statistically significantly more successful than delaying treatment in both children and adults regardless of severity (Greene et al., 1988). Thus, starting treatment early is important.
This therapy is generally well tolerated when the dose is increased slowly. We recommend starting with a 5 mg preparation, half-tablet at bedtime, and adding a half-tablet a week, advancing up to 10 mg in four divided doses by the end of 4 weeks. Because there is a lag between reaching a dose and seeing a benefit, we hold this dose for a month, and then continue to increase at the same rate over the next 4 weeks until the dose is 20 mg in four divided doses. We continue to increase until there is adequate benefit or adverse effects that limit higher doses. Some patients require up to 60–140 mg/day, but may experience dose-related drowsiness, confusion, or memory difficulty that limit the dose. Children usually tolerate the very high dosages, whereas adults do not. In one study of 20 cognitively intact patients with dystonia, only 12 of whom could tolerate 15–74 mg of daily trihexyphenidyl, drug-induced impairments of recall and slowing of mentation were noted, particularly in the older patients (Taylor et al., 1991). Diphenhydramine, a histamine H1 antagonist with anticholinergic properties, has been reported to have an antidystonic effect in three of five patients (Truong et al., 1995). However, the drug was not effective in 10 other patients with cervical dystonia, and it was associated with sedation and other anticholinergic side effects in most patients. Pyridostigmine, a peripherally acting anticholinesterase, and eye drops of pilocarpine (a muscarinic agonist) often ameliorate many of the peripheral side effects, such as urinary retention and blurred vision. Pilocarpine (Salagen) 5 mg four times per day, Cevimeline (Evoxac) 30 mg three times per day, and synthetic saliva (Glandosane, Salagen, Salivart, Salix) have been found effective in the treatment of dry mouth.
Other pharmacologic therapies
Many patients with dystonia require a combination of several medications and treatments (Jankovic, 2004b, 2006). High-dosage oral baclofen appears to be the next most effective agent for dystonia, particularly in combination with high-dosage anticholinergic treatment. Baclofen is a GABAb autoreceptor agonist that is used to treat spasticity. It has been found to produce substantial and sustained improvement in 29% of children at a mean dose of 92 mg/day (range: 40–180 mg/day) (Greene, 1992; Greene and Fahn, 1992a). Although baclofen was initially effective in 28 of 60 (47%) of adults with cranial dystonia, only 18% continued baclofen at a mean dose of 105 mg/day after a mean of 30.6 months (Fahn et al., 1985a).
Benzodiazepines (diazepam, lorazepam, or clonazepam) may provide additional benefit for patients whose response to anticholinergic drugs is unsatisfactory. Clonazepam might be particularly useful in patients with myoclonic dystonia. Muscle relaxants that are useful in the treatment of dystonia include cyclobenzaprine (Flexeril, 30–40 mg/day), metaxalone (Skelaxin, 800 mg two to three times per day), carisoprodol (Soma), methocarbamol (Robaxin oral and patch), orphenadrine (Norflex), and chlorzoxazone (Parafonforte). Structurally and pharmacologically similar to amitriptyline, cyclobenzaprine has been found at doses of 30–40 mg/day to be superior to placebo but equal to diazepam. Sodium oxybate, a salt of gamma-hydroxybutyrate, has been also reported to be effective in the myoclonus–dystonia syndrome, similar to alcohol (Frucht et al., 2005).
Narayan and colleagues (1991) first suggested that intrathecal baclofen (ITB) might be effective in the treatment of dystonia in 1991 in a report of an 18-year-old man with severe cervical and truncal dystonic spasms who was refractory to all forms of oral therapy and to large doses of paraspinal BTX injections. Muscle-paralyzing agents were necessary to relieve these spasms, which compromised his respiration. Within a few hours after the institution of ITB infusion, the patient’s dystonia markedly improved, and he was able to be discharged from the intensive care unit within 1–2 days. The subsequent experience with intrathecal infusions has been quite encouraging, and studies are currently in progress to further evaluate this form of therapy in patients with dystonia and other motor disorders (Penn et al., 1995; Dressler et al., 1997; van Hilten et al., 1999). In some patients who were treated with intrathecal baclofen for spasticity, the benefits persisted even after the infusion was stopped (Dressnandt and Conrad, 1996). Ford and colleagues (1996) reviewed the experience with ITB in 25 patients and concluded that this form of therapy may be “more effective when dystonia is associated with spasticity or pain.” ITB could have a role in selected patients with dystonic storm (Dalvi et al., 1998) and in secondary dystonias associated with pain and spasticity (Ford et al., 1998a). For example, Albright and colleagues (1998) found improvement in dystonia scores in 10 of 12 patients with cerebral palsy using an average daily dose of ITB of 575 µg; the improvement was sustained in 6 patients. In a subsequent study involving 86 patients aged 3–42 years (mean: 13 years) with generalized dystonia (secondary to cerebral palsy in 71% of patients), external infusion or bolus-dose screening was positive in approximately 90% of patients (Albright et al., 2001). Programmable pumps were implanted in 77 patients. Infusion began at 200 µg/day and increased by 10–20% per day until the best dose was achieved. The median duration of ITB therapy was 26 months. The mean dose increased over time, from 395 µg at 3 months to 610 µg at 24 months to 960 µg at 36 months. Patients and caregivers rated quality of life and ease of care as having improved in approximately 85% of patients. Seven patients, including four with cerebral palsy, lost their response to ITB during the study, usually during the first year. The most common side effects were increased constipation (19%), decreased neck and trunk control, and drowsiness. Surgical and device complications occurred in 38% of patients, including infections and catheter breakage and disconnection. Complication rates decreased over time. The authors conclude, “In our opinion, ITB is the treatment of choice for severe, generalized secondary dystonia after oral medications have been shown to be ineffective.” One potentially serious complication of ITB is life-threatening intermittent catheter leakage, which might not be detectable by standard noninvasive methods (Bardutzky et al., 2003).
In a long-term (6 years) follow-up of ITB in 14 patients, 5 patients were found to have improvement in their rating scale scores, although only 2 patients had sustained “clear clinical benefit” (Walker et al., 2000). Hou and colleagues (2001) provided a follow-up on long-term effects of ITB in 10 patients (2 males and 8 females; mean age: 43.2 years; range: 21–66 years) with severe segmental or generalized dystonia who responded unsatisfactorily to oral medications and BTX injections. Three patients had peripherally induced dystonia, all with reflex sympathetic dystrophy, two were idiopathic (one with reflex sympathetic dystrophy), two suffered brain injury, two had static encephalopathy, and one had an unknown neurodegenerative disorder. Anatomically, three patients suffered segmental dystonia involving the neck, arms, or abdominal muscles. Seven others had generalized dystonia. The average duration of dystonia prior to implantation was 11 years (range: 1.7–35 years). All patients received test doses of bolus ITB before implantations. Two had relatively poor initial response to the test dose, but still received implantations for continuous infusion. Neurologic assessments were conducted immediately after the implantations, 1 month later, and every 3 months thereafter. The average duration of follow-up was 4.7 years (range: 0.7–11.1 years). Only one patient abandoned the pump after 22 months. Initially, five improved markedly, two improved moderately, two improved mildly, and one had no improvement. Both patients who failed to respond to the initial test of ITB improved on continuous infusion with pump. In four patients, the improvement lessened over time, although the patients were still better than they had been preoperatively. All 10 patients needed to continue oral medications, and 8 continued BTX injections at similar doses. Hou and colleagues (2001) concluded that patients with secondary (spastic) dystonia involving primarily legs and trunk appeared to be the best candidates for continuous ITB infusion. Continuous ITB has been found to be safe and effective in other series of patients with reflex sympathetic dystrophy and dystonia (van Hilten et al., 2000). It is not yet clear whether ITB can induce lasting remissions in patients with dystonia. The American Academy for Cerebral Palsy and Developmental Medicine has published a systematic review of the use of ITB for spastic and dystonic cerebral palsy (Butler et al., 2000). The limited published data show that ITB reduced spasticity and dystonia, particularly in the lower extremities.
There are other medications that are used in the treatment of dystonia. For example, slow-release morphine sulfate has been shown to improve not only pain but also dystonic movement in some patients with primary and tardive dystonia (Berg et al., 2001). Besides clonazepam, gamma-hydroxybutyrate, used in the treatment of alcohol abuse, has been found to be beneficial in the treatment of myoclonus–dystonia syndrome (Priori et al., 2000). It is not known whether acamprosate, another drug that is used in the treatment of alcohol abuse, is useful in the treatment of myoclonus–dystonia. Anticonvulsants, such as levetiracetam (Keppra) and zonisamide (Zonegran), have been reported to be effective in the treatment of cortical myoclonus, but it is not clear whether these drugs play a role in the treatment of dystonia. An open-label trial in 10 patients with segmental and generalized dystonia showed no evidence of efficacy (Hering et al., 2007).
Peripheral deafferentiation with anesthetic was previously reported to improve tremor (Pozos and Iaizo, 1992), but this approach might also be useful in the treatment of focal dystonia, such as writer’s cramp (Kaji et al., 1995a) or oromandibular dystonia (Yoshida et al., 1998) that is unresponsive to other pharmacologic therapy. An injection of 5–10 mL of 0.5% lidocaine into the target muscle improved focal dystonia for up to 24 hours. This short effect can be extended for up to several weeks if ethanol is injected simultaneously. The observation that blocking muscle spindle afferents reduces dystonia suggests that somatosensory input is important in the pathogenesis of dystonia (Hallett, 1995; Kaji et al., 1995b). Mexiletine, an oral derivative of lidocaine, has been found to be effective in the treatment of cervical dystonia at doses ranging from 450 to 1200 mg/day (Ohara et al., 1998). Two-thirds of the patients, however, experienced adverse effects, including heartburn, drowsiness, ataxia, and tremor. On the basis of a review and a rating of videotapes by a “blind” rater, Lucetti and colleagues (2000) reported a significant improvement in six patients with cervical dystonia who had been treated with mexiletine.
Local electromyograph (EMG)-guided injection of phenol is currently being investigated as a potential treatment of cervical dystonia, but the results have not been very encouraging because of pain associated with the procedure and unpredictable response (Ruiz and Bernardos, 2000; Massey, 2002). Chemomyectomy with muscle-necrotizing drugs, such as doxorubicin, has been tried in some patients with blepharospasm and hemifacial spasm (Wirtschafter, 1991), but because of severe local irritation, it is doubtful that this approach will be adopted into clinical practice.
Attacks of kinesigenic paroxysmal dystonia may be controlled with anticonvulsants (e.g., carbamazepine, phenytoin) (Table 13.2). The nonkinesigenic forms of paroxysmal dystonia are less responsive to pharmacologic therapy, although clonazepam and acetazolamide may be beneficial. Treatment of paroxysmal dyskinesias is covered in Chapter 22 and in other reviews (Fahn, 1994; Demirkiran and Jankovic, 1995). Table 13.3 lists the common medications and procedures utilized for dystonia.
Table 13.3 Treatment of dystonia
Focal dystonias |
Blepharospasm |
Oromandibular dystonia |
Spasmodic dysphonia |
Cervical dystonia |
Task-specific dystonias (e.g., writer’s cramp) |
Segmental and generalized dystonias |
In addition to conventional forms of therapy described previously, many patients with dystonia seek complementary or alternative forms of therapy. In one survey of 180 members of the German Dystonia Group, 131 (73%) patients used some form of alternative treatments, such as acupuncture (56%), relaxation techniques (44%), homeopathy (27%), and massages (26%) (Junker et al., 2004).
The involuntary muscle contraction can lead to “status dystonicus” or “dystonic storm” resulting in breakdown of the muscle and a life-threatening myoglobinuria (Opal et al., 2002; Mariotti et al., 2007). In a review of 37 cases of status dystonicus or dystonic storm, the following treatment approach has been used with some success: early admission to the intensive care unit, monitor for evidence of myoglobinuria, avoid respiratory and renal compromise, sedation with intravenous midazolam (initially up to 10 µg/kg/min and subsequently at 30–100 µg/kg/hour), possible barbiturate anesthesia combined with endotracheal intubation and mechanical ventilation, continuous intrathecal baclofen, bilateral pallidotomy, or globus pallidus interna (GPi) deep brain stimulation (DBS) (Mariotti et al., 2007; Jech et al., 2009). Although administration of propofol has been also suggested, we would caution against it as this drug has been demonstrated to cause or exacerbate levodopa-related dyskinesia (Krauss et al., 1996).
Botulinum toxin
The introduction of BTX into clinical practice in the late 1980s revolutionized treatment of dystonia. The most potent biologic toxin, BTX has become a powerful therapeutic tool in the treatment of a variety of neurologic, ophthalmic, and other disorders that are manifested by abnormal, excessive, or inappropriate muscle contractions (Jankovic and Brin, 1991; Jankovic and Hallett, 1994; Brin et al., 2002; Thant and Tan, 2003; Jankovic, 2004a). In December 1989, after extensive laboratory and clinical testing, the Food and Drug Administration approved this biologic (BTX-A or Botox) as a therapeutic agent in patients with strabismus, blepharospasm, and other facial nerve disorders, including hemifacial spasm. In December 2000, the Food and Drug Administration approved Botox and BTX-B (Myobloc) as treatments for cervical dystonia. Although its widest application is still in the treatment of disorders manifested by abnormal, excessive, or inappropriate muscle contractions, the use of BTX is rapidly expanding to include treatment of a variety of ophthalmologic, gastrointestinal, urologic, orthopedic, dermatologic, secretory, painful, and cosmetic disorders (Tintner and Jankovic, 2001b; Jankovic and Brin, 2002; Thant and Tan, 2003; Jankovic, 2004a) (Fig. 13.1; Tables 13.4 and 13.5).
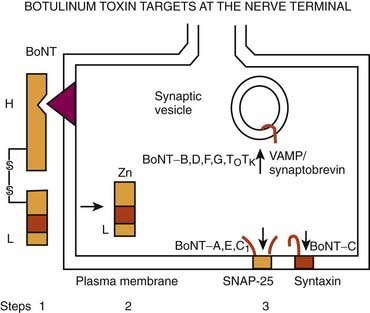
Figure 13.1 Mechanism of action of botulinum toxin.
Redrawn from Jankovic J. Botulinum toxin in clinical practice. J Neurol Neurosurg Psychiatry 2004;75:951–957.
Table 13.4 Clinical applications of botulinum toxin
Focal dystonia |
Other involuntary movements |
Inappropriate contractions |
Other potential applications |
Few therapeutic agents have been better understood in terms of their mechanism of action before their clinical application or have had greater impact on patients’ functioning than BTX. The therapeutic value of BTX is due to its ability to cause chemodenervation and to produce local paralysis when injected into a muscle. There are seven immunologically distinct toxins that share structurally homologous subunits. Synthesized as single-chain polypeptides (molecular weight of 150 kDa), these toxin molecules have relatively little potency until they are cleaved by trypsin or bacterial enzymes into a heavy chain (100 kDa) and a light chain (50 kDa). The 150 kDa protein, the active portion of the molecule, complexes with one or more nontoxin proteins that support its structure and protect it from degradation. Type A is the only serotype that forms the 900 kDa complex. Types A, B, C1, and hemagglutinin-positive D form the 500 kDa complex and 300 kDa complex; types E, F, and hemagglutinin-negative D form only the 300 kDa complex (Melling et al., 1998). The three-dimensional structure of the BTX complex is known (Hanson and Stevens, 2002). When linked by a disulfide bond, these dichains exert their paralytic action by preventing the release of acetylcholine. BTX therefore does not affect the synthesis or storage of acetylcholine, but it interferes with the release of acetylcholine from the presynaptic terminal. This is a three-step process that involves binding to the acceptors on presynaptic membrane (heavy chain), internalization (endocytosis), and an enzymatic action (light chain). BTX serotypes bind to different acceptors, which contain both protein components and gangliosides with more than one neuraminic acid residue. BTX-A has been found to enter neurons by binding to the synaptic vesicle protein SV2 (isoform C) which acts as the BTX-A receptor (Dong et al., 2006; Mahrhold et al., 2006). The neural membrane proteins synaptotagmin I and II act as receptors for BTX-B and for BTX-G (Mahrhold et al., 2006). While the heavy chain of the toxin binds to the presynaptic cholinergic terminal, the light chain acts as a zinc-dependent protease that selectively cleaves proteins that are critical for fusion of the presynaptic vesicle with the presynaptic membrane. Thus, the light chains of BTX-A and BTX-E cleave SNAP-25 (synaptosome-associated protein), a protein that is needed for synaptic vesicle targeting and fusion with the presynaptic membrane. The light chains of BTX-B, BTX-D, and BTX-F prevent the quantal release of acetylcholine by proteolytically cleaving synaptobrevin-2, also known as VAMP (vesicle-associated membrane protein), an integral protein of the synaptic vesicle membrane. BTX-C cleaves syntaxin, another plasma membrane-associated protein. A three-dimensional study showed that syntaxin 1a forms a complex with neuronal-Sec1, forming a recognition site for the arriving vesicle through a Rab protein, and the subsequent formation of the syntaxin–VAMP–SNAP-25 complex promotes membrane fusion (Misura et al., 2000) (Fig. 13.1).
BTX-A has been studied most intensely and used most widely, but the clinical applications of other types of toxins, including BTX-B and BTX-F, are also expanding (Greene and Fahn, 1992b; Mezaki et al., 1995; Sheean and Lees, 1995; Figgit and Noble, 2002; Jankovic, 2004a). BTX-A is harvested from a culture medium after fermentation of a high-toxin-producing strain of Clostridium botulinum that lyses and liberates the toxin into the culture (Schantz and Johnson, 1992). The toxin is then extracted, precipitated, purified, and finally crystallized with ammonium sulfate. The crystalline toxin is diluted from milligram to nanogram concentrations, freeze-dried, and dispensed as a white powder in small vials containing 100 mouse units (U) of the toxin. When isolated from bacterial cultures, BTX is noncovalently associated with nontoxic macromolecules, such as hemagglutinin. These nontoxic proteins enhance toxicity by protecting the neurotoxin from proteolytic enzymes in the gut, but they apparently have no effect on the potency of the toxin if injected parenterally. Although the efficacy and duration of benefits of BTX-B or Myobloc, formerly NeuroBloc (Elan), are thought to be generally comparable to those of BTX-A (Botox and Dysport), no head-to-head comparisons between the various products have been performed. An in-vivo study using injections into the extensor digitorum brevis in healthy volunteers suggested that the muscle paralysis from BTX-B was not as complete or long-lasting as that from BTX-A (Sloop et al., 1997). Whether M-wave amplitude is a reliable measure of clinical response and whether the doses of BTX-A (7.5–10 U) and BTX-B (320–480 U), with a B/A ratio of about 45 : 1, are comparable are debatable. The apparently longer duration of action of BTX-A compared to that of BTX-B could possibly be explained by the observation that VAMP that is cleaved by BTX-B cannot form stable SNARE (soluble N-ethlymaleimide sensitive factor attachment protein receptor or SNAP receptor or neuronal synaptosome-associated proteins) complex and it turns over to form new VAMP, whereas SNAP-25, the substrate for BTX-A, forms a truncated SNAP-25A, which prevents degradation of SNARE, as a result of which the inhibition of exocytosis persists for 40–60 days. This correlates well with the reappearance of the original terminals (de Paiva et al., 1999). De Paiva and colleagues (1999) noted that a single intramuscular injection of BTX-A into the sternomastoid muscles of mice caused the formation of functional neuronal sprouts that connected with the muscle fiber. The primary BTX-A-intoxicated nerve terminal was incapable of neurotransmitter exocytosis; it produced new sprouts that were capable of exocytosis with subsequent upregulation of adjacent nicotinic receptors on the muscle fiber, thus forming a functional synapse. After a certain period of time, such as 3 months, consistent with return of clinical function of the muscle and wearing-off response from the previous injection, the original BTX-A-intoxicated terminal resumed exocytosis, and the sprouts regressed to return the neuromuscular junction to its original state. Other studies have also shown that the prolonged duration of action of BTX-A is due to persistence of catalytically active enzyme in the muscle cell (Adler et al., 2001). A physiologic study in monkeys showed that BTX-B diffuses less extensively than BTX-A into adjacent and remote muscles (Arezzo et al., 2002). In contrast to Botox, Myobloc is a solution that does not require reconstitution, and it may be stored in a refrigerator rather than a freezer. The commercial preparations are complex proteins that must be dissociated from the active neurotoxin molecule to exert the paralytic effect. This dissociation, which is pH dependent, unmasks the binding site on the heavy chain.
In addition to Botox, Myobloc, and Dysport (Truong et al., 2005), a new formulation of BTX-A, NT 201, has been recently introduced (Benecke et al., 2005). This formulation of BTX-A, which is free of complexing proteins, has been reported to be equivalent, in terms of efficacy and safety, to Botox in healthy volunteers and in patients with blepharospasm (Roggenkamper et al., 2006; Jankovic, 2009b) and cervical dystonia (Benecke et al., 2005). While low antigenicity has been predicted with this new formulation of BTX, no long-term data exist, and in view of the low frequency of blocking antibodies reported with the new formulation of Botox (see later) (Jankovic et al., 2003; Brin et al., 2008; Naumann et al., 2010) (Tables 13.6 and 13.7), it is not clear what role the new NT 201 BTX will play in the future treatment of dystonia. Patients resistant to BTX-A often initially respond to BTX-B, but a recent study showed that continued treatment with Myobloc is associated with high incidence of blocking antibodies (Jankovic et al., 2006).
Table 13.6 Risk factors for development of blocking antibodies (immunoresistance)
Table 13.7 Long-term effects of repeat botulinum toxin injections in cervical dystonia
From: Brin MF, Comella CL, Jankovic J, Lai F, Naumann M; CD-017 BoNTA Study Group. Long-term treatment with botulinum toxin type A in cervical dystonia has low immunogenicity by mouse protection assay. Mov Disord 2008;23:1353–1360.
The primary effect of BTX is to induce paralysis of injected skeletal muscles, especially the most actively contracting muscles. BTX paralyzes not only the extrafusal fibers but also the intrafusal fibers, thus decreasing the activity of Ib muscle afferents (Filippi et al., 1993). This might explain the effect of BTX on reciprocal inhibition. In untreated patients with dystonia, the second phase of reciprocal inhibition is usually decreased. BTX “corrects” the abnormal reciprocal inhibition by increasing the second phase, possibly through its effect on the muscle afferents (Priori et al., 1995). While the effect on intrafusal fibers might contribute in part to the beneficial action of BTX in patients with dystonia, this is not its main action because BTX is effective in facial dystonia, even though the facial muscles do not have spindles.
Measuring variations in fiber diameter and using acetylcholine-esterase staining as indexes of denervation, Borodic and colleagues (1994) showed that BTX diffuses up to 4.5 cm from the site of a single injection (10 U injected in the rabbit longissimus dorsi). Since the size of the denervation field is determined largely by the dose (and volume), multiple injections along the affected muscle rather than a single injection should therefore contain the biologic effects of the toxin in the targeted muscle (Borodic et al., 1992). Blackie and Lees (1990) also showed that frequency of dysphagia could be reduced by 50% when multiple rather than single injections are used.
A small percentage of patients receiving repeated injections develop antibodies against BTX, causing them to be completely resistant to the effects of subsequent BTX injections (Greene et al., 1994; Jankovic and Schwartz, 1995; Jankovic, 2002) (Tables 13.6 and 13.7). In one study, 24 of 559 (4.3%) patients treated for cervical dystonia developed BTX antibodies (Greene et al., 1994). The authors suggested that the true prevalence of antibodies might be more than 7%. In addition to patients with BTX antibodies, they studied 8 patients from a cohort of 76 (10.5%) who stopped responding to BTX treatments. These BTX-resistant patients had a shorter interval between injections, more booster injections, and a higher dose at the non-booster injection compared to nonresistant patients treated during the same period. As a result of this experience, clinicians are warned against using booster injections and are encouraged to extend the interval between treatments as long as possible, certainly at least 1 month, and to use the smallest possible dose. In addition to high dosages, young age is a potential risk factor for the development of immunoresistance to BTX-A (Jankovic and Schwartz, 1995; Hanna and Jankovic, 1998; Hanna et al., 1999). Some of the patients who developed BTX-A antibodies have benefited from injections by immunologically distinct preparations, such as BTX-F and BTX-B (Greene and Fahn, 1992b; Mezaki et al., 1994). After 1–3 years, some patients become antibody negative, and when reinjected with the same type of toxin, they may again experience transient benefit (Sankhla et al., 1998). The original preparation of Botox contained 25 ng of neurotoxin complex protein per 100 units, but in 1997, the Food and Drug Administration approved a new preparation that contains only 5 ng per 100 units, which should have lower antigenicity (Aoki et al., 1999). In fact, in a 3-year follow-up of patients treated with the current Botox, no evidence of blocking antibodies was found, compared to a 9.4% frequency of blocking antibodies in patients treated with the original Botox for the same period of time (Jankovic et al., 2003). The low antigenicity of the current Botox is also supported by the findings from a longitudinal, multicenter study in which de novo subjects were treated with Botox and only 4/326 (1.2%) subjects tested positive for antibodies by the mouse protective assay during the course of up to 15 cycles of treatment (Brin et al., 2008) (Table 13.7).
The preliminary data suggest that BTX-B provides clinical effects similar to those of BTX-A, but the duration of benefits is shorter (Comella et al., 2005) and Myobloc appears to have greater antigenicity than Botox, particularly in patients with prior resistance to BTX-A (Jankovic et al., 2007). To compare autonomic effects of BTX, Tintner and colleagues (2005) randomized patients with cervical dystonia to receive either BTX-A or BTX-B in a double-blind manner. Efficacy and physiologic questionnaire measures of autonomic function were assessed at baseline and 2 weeks after injection. Patients who were treated with BTX-B had significantly less saliva production (P < 0.01) and greater severity of constipation (P = 0.037) than those treated with BTX-A but did not differ with respect to other tests of autonomic function, including changes in blood pressure, heart rate, and ocular function. The autonomic effects of BTX-B were reviewed in several additional reports (Wan et al., 2005).
Many clinical studies have provided evidence not only that BTX is safe and effective, but also that this therapy leads to meaningful improvements in quality of life (Naumann and Jankovic, 2004; Jankovic et al., 2004), and the benefits are long-lasting (Mejia et al., 2005) (Video 13.1). Despite its proven therapeutic value, there are still many unresolved issues and concerns about BTX. These include lack of standardization of biologic activity of the different preparations of BTX, poor understanding of toxin antigenicity, variations in the methods of injection, and inadequate assays for BTX antibodies (Brin et al., 2008). Training guidelines for the use of botulinum toxin have been established (American Academy of Neurology, 1994). Clinicians who are interested in utilizing BTX chemodenervation in their practice must be aware of these concerns and must exercise proper precautions to minimize the potential risks associated with BTX. Most important, they should become thoroughly familiar with the movement disorders that they intend to treat and with the anatomy at the injection site. Possible contraindications to the use of BTX include the presence of myasthenia gravis (Emerson, 1994), Eaton–Lambert syndrome, motor neuron disease, aminoglycoside antibiotics, and pregnancy. Besides occasional complications, usually related to local weakness, a major limitation of BTX therapy is its high cost. Several studies analyzing the cost-effectiveness of BTX treatment, however, have demonstrated that the loss of productivity as a result of untreated dystonia and the cost of medications or surgery more than justify the financial expense of BTX treatments. It is likely that future research will result in the development of new, more effective neuromuscular blocking agents that provide therapeutic chemodenervation with long-term benefits and at lower cost.
Blepharospasm
The effectiveness of BTX in blepharospasm was first demonstrated in double-blind, placebo-controlled trials (Fahn et al., 1985b; Jankovic and Orman, 1987). In a subsequent report of experience with BTX in 477 patients with various dystonias and hemifacial spasm, Jankovic and colleagues (1990) reviewed the results in 90 patients who had been injected with BTX for blepharospasm. Moderate or marked improvement was noted in 94% of the patients. The average latency from the time of the injection to the onset of improvement was 4.2 days; the average duration of maximum benefit was 12.4 weeks, but the total benefit lasted considerably longer: an average of 15.7 weeks. While 41% of all treatment sessions were followed by some side effects (ptosis, blurring of vision or diplopia, tearing, and local hematoma), only 2% of these affected patient’s functioning. Complications usually improved spontaneously in less than 2 weeks. These results are consistent with those of other studies (Elston, 1994). There is no apparent decline in benefit, and the frequency of complications actually decreases after repeat BTX treatments (Jankovic and Schwartz, 1993a).
The efficacy and safety of another type of BTX-A, NT-201 (or Xeomin), in the treatment of blepharospasm was also confirmed by a prospective, double-blind, placebo-controlled, randomized, multicenter study involving 109 patients (mean total dose of Xeomin per treatment visit was 64.8 U), in which the Jankovic Rating Scale severity subscore was significant reduced compared to placebo (P < 0.001). The most commonly reported adverse effects related to Xeomin versus placebo were eyelid ptosis (18.9 vs. 8.8%), dry eye (16.2 vs.11.8%), and dry mouth (16.2 vs. 2.9%) (Jankovic, 2009b; Jankovic et al., 2011). In a multicenter, clinical, fixed-dose, trial Dysport (40, 80, and 120 units/eye) or placebo (in a 3 : 1 randomization ratio) were administered to 119 patients with blepharospasm, 85 of whom completed the 16-week follow-up; several parameters showed robust improvement in Dysport arms compared to placebo (Truong et al., 2008).
In addition to the four different BTX-A preparations available on the market in one or more countries, Botox (Allergan Inc., Irvine, CA, USA), Dysport (Ipsen Ltd, Slough, UK), Xeomin (Merz Pharmaceuticals, Frankfurt am Main, Germany) (Albanese, 2011), and Prosigne (Lanzhou Biological Products, China), Meditoxin (Medy-Tox, Seoul, Korea; also known as Neuronox) was introduced for the treatment of blepharospasm and is currently available in Korea (Yoon et al., 2009). All these types were evaluated in blepharospasm and were found to be comparable with respect to clinical efficacy and adverse effects.
Reasons for the gradual enhancement in efficacy and reduction in the frequency of complications with repeat treatments include greater experience and improvements in the injection technique. For example, one controlled study showed that an injection into the pretarsal rather than preseptal portion of the orbicularis oculi is associated with significantly lower frequency of ptosis (Jankovic, 1996). Ptosis can be prevented also by injecting initially only the lateral and medial portions of the upper lid, thus avoiding the midline levator muscle. We usually initially inject 5 U in each site in the upper lid and 5 U in the lower lid laterally only. This finding has been confirmed by others (Cakmur et al., 2002).
The functional improvement, experienced by the vast majority of patients after BTX injection, is difficult to express numerically. Many patients could not work, drive, watch television, or read prior to the injections. As a result of reduced eyelid and eyebrow spasms, most can now function normally. In addition to the observed functional improvement, there is usually a meaningful amelioration of discomfort, and because of less embarrassment, the patients’ self-esteem also frequently improves. BTX injections are now considered by many to be the treatment of choice for blepharospasm (American Academy of Ophthalmology, 1989; American Academy of Neurology, 1990). In addition to idiopathic blepharospasm, BTX injections have been used effectively in the treatment of blepharospasm induced by drugs (e.g., levodopa in parkinsonian patients or neuroleptics in patients with tardive dystonia), dystonic eyelid and facial tics in patients with Tourette syndrome, and blepharospasm associated with apraxia of eyelid opening (Jankovic, 1994; Aramideh et al., 1995; Lepore et al., 1995; Jankovic, 1995a, 1996; Forget et al., 2002).
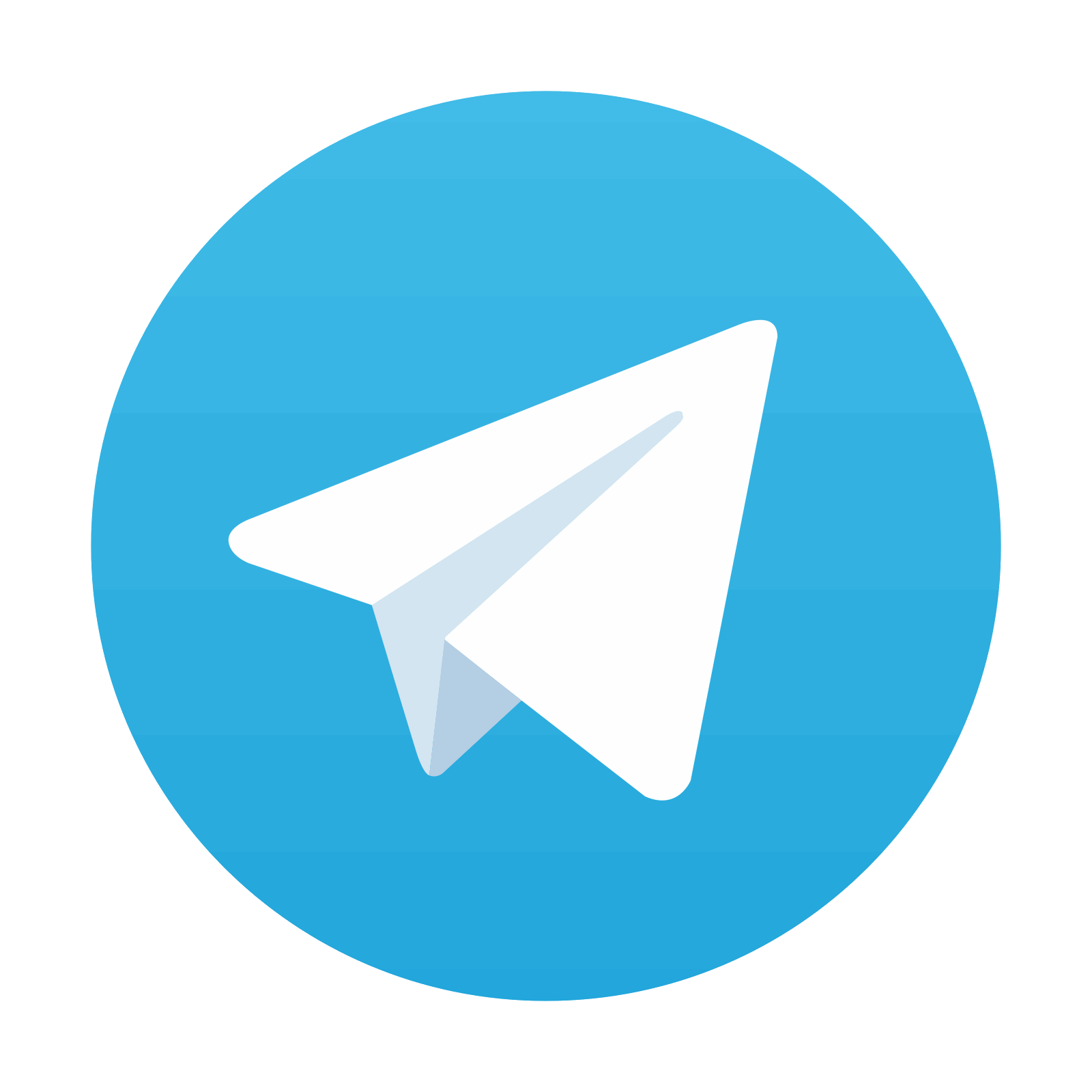
Stay updated, free articles. Join our Telegram channel

Full access? Get Clinical Tree
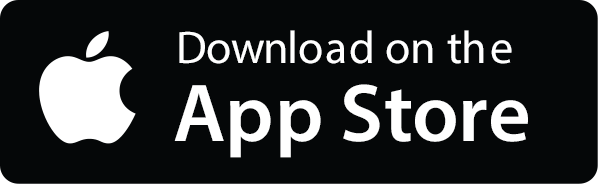
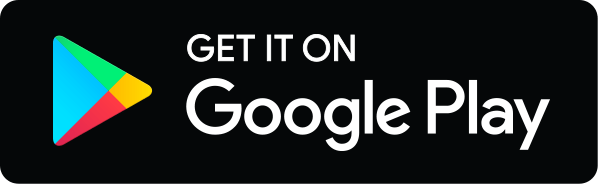