Treatment of Hydrocephalus with Shunts
Since the invention of the first implantable shunt valve by Nulsen and Spitz 60 years ago,1 there have been innumerable innovations and new designs of shunt equipment to treat pediatric hydrocephalus. Shunts have made a dramatic impact on a previously devastating disease; however, despite the rational and seemingly more physiologic designs of these new devices, complications related to ventriculoperitoneal (VP) shunts continue to plague children with hydrocephalus. It is hoped that continued basic and clinical research will lead to advances in the management of pediatric hydrocephalus and improve the quality of life of affected children and their families.
10.1 Epidemiology
The management of hydrocephalus with cerebrospinal fluid (CSF) shunts is the most common neurosurgical problem encountered in the pediatric age group. Based on the Kids’ Inpatient Database, the Hydrocephalus Clinical Research Network estimated 38,200 to 39,900 admissions, 391,000 to 433,000 hospital days, and total hospital charges of $1.4 to $2.0 billion for pediatric hydrocephalus.2 Clearly, the treatment of hydrocephalus with shunts carries significant costs to patients, families, health care centers, and funding organizations.
10.2 Clinical Presentation
The various symptoms associated with hydrocephalus are discussed in Chapter ▶ 7. In this chapter, we discuss the criteria used in the determination of treatment for children with hydrocephalus, specifically in relation to CSF shunt insertion.
First-time shunt insertion is predominantly performed by pediatric neurosurgeons. In the International Society for Pediatric Neurosurgery (ISPN) database, 73% of patients who presented for first-time shunt insertion (774 patients) were 6 months of age or less at time of insertion.4 Furthermore, the median corrected age of patients entered in the Shunt Design Trial (SDT) was 55 days.5 In the endoscopic shunt insertion trial (ESIT), the median corrected age of patients was less than 3 months.6 The clinical characteristics of the patients undergoing first-time shunt insertion who were enrolled in SDT are outlined in ▶ Table 10.1 and ▶ Table 10.2. The most common causes for hydrocephalus were IVH, myelomeningocele, and brain tumors.
Etiology of hydrocephalus | Percentage |
Intraventricular hemorrhage | 24 |
Myelomeningocele | 21 |
Brain tumor | 9 |
Aqueductal stenosis | 7 |
Cerebrospinal fluid infection | 5 |
Head injury | 2 |
Two indicated | 9 |
Other | shun |
Unknown | 12 |
Source: From Kestle JRW, Garton HJL, Drake JM. Treatment of hydrocephalus with shunts. In: Albright L, Pollack I, Adelson D, eds. Principles and Practice of Pediatric Neurosurgery. New York, NY: Thieme Medical Publishers;1999:76.94 Republished with permission. |
Symptoms | Percentage | Signs | Percentage | Preoperative imaging | Percentage |
Irritability | 27 | Increased head circumference | 81 | Magnetic resonance | 23 |
Nausea and vomiting | 19 | Bulging fontanel | 71 | Computed tomography | 57 |
Headache | 18 | Delayed development | 21 | Ultrasound only | 20 |
Lethargy | 18 | Loss of upward gaze | 16 | ||
New seizure or change in seizure pattern | 7 | Decreased level of consciousness | 13 | ||
Diplopia | 6 | Papilledema | 12 | ||
Worsening school performance | 4 | Sixth nerve palsy | 5 | ||
Hemiparesis | 4 | ||||
Fever | 3 | ||||
Nuchal rigidity | 2 | ||||
Other neurologic deficit | 12 | ||||
Source: From Kestle JRW, Garton HJL, Drake JM. Treatment of hydrocephalus with shunts. In: Albright L, Pollack I, Adelson D, eds. Principles and Practice of Pediatric Neurosurgery. New York, NY: Thieme Medical Publishers;1999:76.94 Republished with permission. |
10.2.1 Diagnostic Tests
The decision to treat hydrocephalus is usually precipitated by the observation of ventricular enlargement. In the baby with an open fontanel, ultrasound will quite readily determine whether there is obvious ventriculomegaly. The addition of computed tomography (CT) or magnetic resonance (MR) imaging to the evaluation will vary depending on the center and the clinical characteristics of the patient being considered. Most surgeons prefer to obtain CT scans or MR images before shunt insertion to assess the morphology of the ventricles (including cysts and compartments) and the condition of the surrounding brain. The exception is hydrocephalus associated with myelomeningocele; some surgeons will place shunts in these patients based on the ultrasound findings alone.5
Ventriculomegaly may be due to hydrocephalus or atrophy of surrounding brain tissue. The differentiation between these two conditions is crucial in deciding to place a shunt. Increasing head circumference or signs of raised intracranial pressure (ICP) make the differentiation straightforward. In more difficult cases, several imaging characteristics may help. The radiographic parameters that suggest hydrocephalus rather than atrophy include the following7:
Dilated temporal horns
Enlarged anterior and posterior recesses of the third ventricle
Downward displacement of the floor of the third ventricle
Dilatation and rounding of the frontal horns
Effacement of the sulci
Periventricular interstitial edema
In addition, inferiorly displaced cerebellar tonsils seen on sagittal MR imaging may indicate raised ICP.
10.3 Indications for a Shunt
Although the management of hydrocephalus is the most common clinical problem in pediatric neurosurgery, the decision to insert a shunt can be one of the most difficult. In the management of hydrocephalus and shunts, the old adage “If it ain’t broke, don’t fix it”8 is well worth keeping in mind.
Ventriculomegaly, either in a baby who presents with irritability, vomiting, a full fontanel, splayed sutures, and increasing head circumference or in an older child who presents with headache, vomiting, and papilledema, poses no therapeutic dilemma. Such children have raised ICP in need of treatment. Other children, however, often present with milder symptoms and signs, or unimpressive imaging studies. In these patients, the decision is more difficult. Several terms are used to describe such children, and a wide range of recommendations can be gleaned from the literature. Some authors believe that asymptomatic mild or moderate ventriculomegaly does not need treatment. The definition of symptomatic, therefore, becomes very important; it is here that opinions vary. This is further complicated by the fact that the decision to place a shunt is often made when the child is very young (in the hope of maximizing cognitive development as the child grows and develops) and subtle cognitive problems are difficult to detect.
Fouyas et al used ICP monitoring to assess 18 patients previously without shunts ages 1 to 15 years (mean, 4.1 years) in whom the diagnosis of hydrocephalus was suspected but uncertain.9 The patients presented with developmental delay, deteriorating gait, increasing head circumference, headaches, irritability, or decreasing visual acuity. The clinical findings (examinations sometimes were repeated on several occasions) combined with ultrasound, CT, and MR imaging (done in some) had failed in all cases to reveal an unequivocal need for a shunt. ICP was elevated in 9 of 18 patients, and all of these patients improved symptomatically after shunt placement. The other nine patients had normal pressure and did not undergo shunt insertion. Three of them had a spontaneous resolution of symptoms with observation; the remaining six had persistent symptoms. Whittle et al reported the results of ICP monitoring in 46 children with a clinical and radiographic diagnosis of “arrested” hydrocephalus.10 The 16 patients that had never had shunts placed (ages 1 to 15 years; mean, 6.0 years) presented with either delayed milestones or decreased school performance (n = 4), new or unstable seizure disorders (n = 6), behavioral changes (n = 2), or no symptoms (i.e., incidental finding; n = 4). Of these 16 patients, 10 (69%) had elevated ICP. In a subset of children who underwent IQ testing, 2 of 5 with stable serial scores had elevated ICP, whereas 8 of 9 with falling scores had elevated ICP. The authors concluded that many patients with apparent arrest of hydrocephalus in fact have an insidiously progressive disorder. Overall, however, ICP elevations were just as likely in the completely asymptomatic group as in the group with evidence of cortical dysfunction.
McLone et al defined compensated hydrocephalus as untreated hydrocephalus that is clinically and radiographically stable.11,12 In such patients, a variety of imaging studies and invasive tests (e.g., infusion tests, radionuclide studies, ICP monitoring, and Doppler imaging) have been proposed to differentiate which children with apparently asymptomatic ventricular enlargement would benefit from shunt placement. Children who are older than 5 years with a stable clinical course and ventricle size may be monitored without a shunt, but they do require frequent testing of their intellectual development. Children who are younger than 5 years old, particularly those younger than 3 years old, who have anything more than mild hydrocephalus should have shunts placed. This younger group is difficult to assess for intellectual development, and mere attainment of developmental milestones is insufficient to determine ultimate intellectual function.11,12 Measurement of opening pressure may be helpful in some cases.
Dias et al conducted a survey study to analyze the threshold for pediatric neurosurgeons to place shunts in asymptomatic children with ventriculomegaly.13 The majority of respondents, who represented approximately 25% of full-time practicing pediatric neurosurgeons in North America, considered themselves relatively conservative when it came to shunt placement. Across the 22 scenarios presented, however, there was significant variability in the responses to certain cases, especially those in which developmental delays and head circumference were considered abnormal. The study concluded that the overall threshold for shunt placement was high, but significant treatment variability existed when it came to vague cases.
MR spectroscopy has been suggested as a method of differentiating atrophy from hydrocephalus. Brain lactate levels were more commonly elevated and ratios of N-acetyl aspartate to creatine were lower in children with atrophy than in those with hydrocephalus; however, three of five patients in the atrophic group had inborn errors of metabolism, possibly accounting for some of the metabolic abnormalities seen.14
Preterm infants with IVH pose another dilemma in regard to CSF diversion. There are few randomized studies to support surgical indications for temporizing devices, such as reservoirs and subgaleal shunts, and similarly limited data for shunt placement. Riva-Cambrin et al analyzed four large pediatric centers to determine whether clinical and radiologic factors influenced practice patterns and treatment modalities.15 The study evaluated 110 neonates with grade 3 or 4 IVH treated surgically. Thirty-seven patients underwent permanent shunt placement at a mean gestational age of 39.5 weeks. Seventy-three patients (66%) were treated with temporizing devices, and in 65 (89%) of those, the temporizing devices were converted to permanent shunts. The only factors statistically significant in determining placement of a temporizing device were bradycardia episodes, full fontanel, and splayed cranial sutures. Factors associated with conversion to a shunt included a full fontanel and increased ventricular size. Overall, no clear factors aside from bradycardia and ventriculomegaly were significant in determining conversion of temporary to permanent implants. Furthermore, the authors described a “center effect” in determining whether patients underwent a temporizing procedure.
In summary, particularly difficult cases may require ICP monitoring or other adjunctive tests in the assessment of possible hydrocephalus, but in most children, the decision can be based on observation over time. Documentation of a progressive problem is a key factor in the decision (▶ Fig. 10.1). This may include progressive developmental or cognitive delay. The most difficult decision is in the young child with compensated hydrocephalus and moderate to severe ventriculomegaly. Further research in this group of children is needed. Additionally, there is significant variability among surgeons and centers in treatment modalities and practice patterns, and thus a greater effort must be devoted to establishing treatment protocols based on randomized and well-evaluated data.
Fig. 10.1 Selection of patients for shunt insertion.
(From Kestle JRW, Garton HJL, Drake JM. Treatment of hydrocephalus with shunts. In: Albright L, Pollack I, Adelson D, eds. Principles and Practice of Pediatric Neurosurgery. New York, NY: Thieme Medical Publishers; 1999:75–91.94 Republished with permission.)
10.3.1 Disease-Specific Considerations
Posttraumatic Ventriculomegaly
What is the best management of a 13-year-old child who, after experiencing a closed head injury at 10 years of age, has a history of development delay and MR images showing enlarged ventricles? The child’s rehabilitation physician asks whether a shunt would facilitate recovery. This case presents a rare complication of head injury in pediatrics; however, it is a good example of a case that requires the differentiation of atrophy from hydrocephalus.
Marmarou et al monitored 75 patients whose head injuries resulted in a Glasgow Coma Scale (GCS) score of 8 or less.16 According to the size of the frontal horns, the ICP, and the resistance to CSF absorption (measured with a lumbar infusion test), the authors classified patients as having normal ICP, benign intracranial hypertension, atrophy, normal-pressure hydrocephalus, or high-pressure hydrocephalus. They advocated placing shunts in patients in the last two groups; 4 of 15 such patients had shunts placed. All four improved one level on the GCS. In their patients, distention of the temporal horns or third ventricle and periventricular lucency were not specific for hydrocephalus (as they defined it). Subarachnoid hemorrhage on the baseline scan was more common (70% vs. 16%) in patients with hydrocephalus.
Posterior Fossa Tumor
Routine preoperative shunt placement in patients with tumor is no longer common practice because many patients remain shunt-free after tumor removal. Dias and Albright reported a series of 58 patients with posterior fossa tumors and hydrocephalus.17 Of these, 25 had shunts placed preoperatively, 17 had external ventricular drains (EVDs), and 16 had no preoperative ventricular catheterization. Of the 33 patients without preoperative shunt placement, 24 remained shunt-free at long-term follow-up. When a Cox regression model was used, two factors were associated with shunt insertion: subtotal tumor resection and incomplete dural closure at surgery.
Lee et al studied 42 children (younger than 20 years old) with newly diagnosed posterior fossa primitive neuroectodermal tumors who did not have shunts at the time of surgery and who survived the perioperative period.18 Of these, 17 (40%) required a shunt by 4 weeks postoperatively, and an additional 2 patients required late shunt placement at the time of tumor recurrence. The group with shunts was younger (5.4 years vs. 10 years), had more severe hydrocephalus, and had more extensive tumor.
At present, a shunt should be withheld in the preoperative phase unless a significant delay between presentation and surgery is expected. Even then, a temporary EVD or a third ventriculostomy may be preferable to a shunt. Many children with a posterior fossa tumor have resolution of their hydrocephalus with tumor removal alone.
At Primary Children’s Medical Center, our practice is to insert an EVD at the time of tumor removal. We avoid placing ventricular drains before surgery because of the risk for upward herniation. If the patient’s clinical condition requires immediate placement of an EVD, our practice is to proceed with tumor removal at that time. The drain is left at 10 to 15 cm above the head for the first 48 hours postoperatively. The EVD is then gradually elevated over the next 3 to 5 days, clamped, and removed if the child remains well. CT to assess ventricular size with the drain clamped is often useful just before EVD removal.
Sainte-Rose et al have reported their results of the management of hydrocephalus in a consecutive group of 206 children with posterior fossa tumors.19 Only 4 of 67 patients (6%) who underwent preoperative third ventriculostomy developed progressive hydrocephalus requiring treatment, compared with 22 of 82 patients (28%) who had conventional treatment preoperatively. This is interesting but in our opinion does not justify the risk of doing a preoperative third ventriculostomy in all patients with posterior fossa tumor because a significant number will not need any treatment for hydrocephalus. An endoscopic third ventriculostomy may be considered for patients who appear drain-dependent after tumor removal, but in our experience, most of these patients eventually require a VP shunt.
Riva-Cambrin et al reported a model for assessing the risk of developing hydrocephalus in pediatric patients with posterior fossa tumors.20 They examined 343 patients with posterior fossa neoplasms and identified predictors of hydrocephalus at 6 months after resection. The preoperative predictors included in the model were age less than 2 years, presence of papilledema, preoperative moderate or severe hydrocephalus, cerebral metastasis, and tumor pathology. A probability scale was developed and validated to predict the need for CSF diversion based on the number of preoperative predictors present.
Myelomeningocele
Several studies are available regarding the timing of shunt placement in children with a myelomeningocele. The reported advantages of simultaneous shunt insertion and back closure are a shorter hospital stay and a decreased incidence of back wound problems.21 The disadvantages of simultaneous surgery are increases in the infection rate and the failure rate of the shunt. There is also the risk of committing some children to a shunt who may have not needed one. At present, both approaches are used, and a clear advantage of one over the other has not been demonstrated.
Caldarelli et al compared simultaneous shunt placement with delayed shunt placement in 89 children with myelomeningocele treated between 1980 and 1994.22 The 1-year failure rates in the simultaneous and delayed shunt insertion groups were 31% and 47%, respectively; infection rates were 23% and 7%. Six patients had a shunt inserted first and underwent delayed back closure; 5 of them (83%) had a shunt infection. At the University of Pittsburgh, 69 patients with myelomeningocele underwent back closure and either simultaneous (n = 21) or delayed (n = 48) shunt placement between 1987 and 1993.21 The two groups were similar in terms of head circumference, but no other comparative information was given. There were 8 children with CSF leak from the lumbar wound in the delayed group and none in the simultaneous group. The rate of obstruction, however, was higher in the simultaneous group, so the overall complication rate was not significantly different between the groups. The infection rate was not different between the groups (1 of 21 and 2 of 48). The authors concluded that simultaneous repair led to shorter hospitalizations and lower rates of back wound morbidity.
Adzick et al published a landmark study comparing prenatal and postnatal repair of myelomeningoceles in regard to primary outcomes, including mortality and the need for a CSF shunt.23 They reported that 40% of infants who underwent intrauterine closure of the myelomeningocele required shunt placement by 12 months, whereas 82% of the patients who underwent postnatal repair required a shunt by 12 months. The study concluded that prenatal closure of myelomeningocele increased fetal, pregnancy, and delivery risk but resulted in a lower need for shunt placement. Secondary outcomes also showed improved motor scores for patients with intrauterine repair.
10.3.2 Shunt Versus Third Ventriculostomy
Once a diagnosis of hydrocephalus has been made and treatment is required, a choice must be made between a shunt and a third ventriculostomy. As is discussed in the next chapter, on neuroendoscopy, several patients with previously placed shunts are now being treated with endoscopic third ventriculostomy. Ideal patients for third ventriculostomy have evidence of noncommunicating, or obstructive, hydrocephalus and preservation of CSF absorption. Unfortunately, patient selection remains a challenge because there is no simple, noninvasive test to assess the adequacy of CSF absorption. In general, patients who have late-onset hydrocephalus from aqueductal stenosis do the best after third ventriculostomy, presumably because they had normal CSF absorption before blockage at the aqueduct. The surgical goal is to create an opening in the floor of the third ventricle between the infundibular recess and the mammillary bodies. This creates a free-flowing communication between the ventricular system and the basal subarachnoid spaces. Endoscopic third ventriculostomy is an option for about a quarter of children with hydrocephalus. The remaining majority, however, will still require a shunt.
10.4 Equipment
VP shunts are composed of three basic elements: a ventricular catheter, a valve, and a peritoneal catheter (▶ Fig. 10.2). There are many variations of each of these components available on the market.
Fig. 10.2 Shunt systems typically contain three parts: a ventricular catheter, a valve with or without a reservoir, and distal tubing. (a) They are available as separate components or (b) unitized.
10.4.1 Ventricular Catheter
Barium-impregnated Silastic (Dow Corning, Midland, MI) tubes that enter the ventricle are available in several configurations. Several transverse diameters are available, but the difference is primarily in the wall thickness because the internal diameters are almost identical. Right-angled ventricular catheters are also available, but they are somewhat limiting because the intracranial length is fixed. If right-angled catheters are used, it is necessary to have several catheters of different lengths available for different clinical situations. Ventricular catheters with flanged tips were thought to minimize proximal obstruction, but this has not been supported in the literature.24,25
Another consideration is whether the ventricular catheter should be a separate or integral part of the valve mechanism. When the ventricular catheter is inserted, if bleeding occurs, it is best to allow drainage of fluid through the ventricular catheter before attaching it to the valve. With an integral ventricular catheter and valve, this is not possible; the blood and cellular debris can occlude the valve immediately. For this reason, we use a separate ventricular catheter.
Recent advances in ventricular catheter development have included antibiotic-impregnated proximal catheters. In prospective trials, the clindamycin- and rifampin-impregnated catheters (Bactiseal; Codman & Shurtleff, Raynham, MA) have been shown to provide antistaphylococcal coverage.
10.4.2 Valves
As outlined previously, most modern valves can be grouped into one of several categories based on their hydrodynamic characteristics24,26: differential-pressure valves, siphon-resisting valves, flow-regulating valves, and adjustable valves (▶ Fig. 10.3 and ▶ Fig. 10.4).
Fig. 10.3 Standard shunt valve designs. In a standard differential-pressure valve (a), flow increases rapidly once the opening pressure is exceeded. The PS Medical Delta (Medtronic Neurosurgical) valve (b) consists of a standard differential-pressure valve (diaphragm type) followed by an antisiphon device to reduce the effects of gravity when the patient is upright. The Orbis Sigma (Cordis Corporation) valve (c) is a flow-limiting valve.
(From Drake JM, Kestle JRW. Determining the best cerebrospinal fluid shunt valve design: the pediatric valve design trial. Neurosurgery 1996;38:605. Republished with permission.)
Fig. 10.4 Programmable shunt valve designs. Both the (a) Strata (Medtronic Neurosurgical) and (b) the Codman-Medos (Codman & Shurtleff) valves allow percutaneous adjustment of the valve pressure with an external magnetic tool. CSF, cerebrospinal fluid.
Differential-Pressure Valves
Differential-pressure valves have been available longer than the other valves, and surgeons have accumulated the most experience with this type of valve. These valves open when the pressure difference across the valve exceeds a predetermined threshold. The valve then remains open, and during this time it has a very low resistance to flow. When the pressure difference drops below the predetermined threshold, the valve closes again and flow stops. When the patient is in the upright position, a large differential pressure between the head and the abdomen develops from the long column of water in the shunt tubing. The valve therefore opens, and fluid flows until the pressure in the head is excessively negative.27 This phenomenon is called siphoning, and it is thought to be responsible for overdrainage and its associated complications (▶ Fig. 10.5).
Fig. 10.5 Schematic diagram illustrating the siphoning effect in patients with shunts. Valve dynamics predominate in the supine position; hydrostatic effects predominate in the upright position.
(From Drake JM, Iantosca MR. Management of pediatric hydrocephalus with shunts. In: McLone DG, ed. Pediatric Neurosurgery: Surgery of the Developing Nervous System. Philadelphia, PA: W. B. Saunders; 2001:508.96 Republished with permission.)
Differential-pressure valves are available with low, medium, and high opening pressures. In general, low-, medium-, and high-pressure valves refer to opening pressures of approximately 5, 10, and 15 cm H2O, respectively. Unfortunately, however, there are no uniform standards for these designations, and the manner in which the pressure is measured is variable. Furthermore, the large column of fluid and the associated negative pressure overwhelm the differences among these three pressures when the patient assumes the upright position. Drake and Sainte-Rose have reviewed the many brands and configurations of differential-pressure valves.24
Siphon-Resisting Valves
The Delta valves (Medtronic Neurosurgical, Goleta, CA) contain a device that is designed to reduce flow as the patient assumes the upright position (i.e., when siphoning occurs). Similar mechanisms are available as separate components that can be added to other shunt systems (e.g., Mueller Heyer-Schulte antisiphon device [Integra, Plainsboro, NJ] and Medtronic Neurosurgical siphon control device).
Flow-Regulating Valves
The Orbis Sigma valve (Cordis Corporation, Bridgewater, NJ) consists of a flexible diaphragm that moves along a piston of variable diameter, resulting in three pressure flow stages. In stage 1, the valve functions like a differential-pressure valve. In stage 2, as the ventricular pressure increases, the diaphragm descends along the piston, whose diameter progressively enlarges. This reduces the flow orifice and dramatically increases the resistance to flow. A very small increase in the flow rate results despite a progressive increase in pressure. In stage 3, a high-pressure safety release mechanism results in open flow when the pressure in the ventricular catheter reaches approximately 40 cm H2O. The diaphragm at this point is beyond the end of the piston and resistance is very low.
Externally Adjustable Valves
More recently developed shunt systems have incorporated an adjustable valve, which enables the surgeon to make noninvasive alterations in the valve’s pressure–flow profile as the patient’s clinical course changes.28–33 Unlike traditional valves, however, programmable valves may be percutaneously adjusted with an external magnet or a special programming tool that works via a magnetic field.34,35 This may be advantageous in patients with normal-pressure hydrocephalus, in patients with arachnoid cysts, and in patients with complications caused by acute or chronic overdrainage, such as subdural hygromas, chronic subdural hematomas, and slit-ventricle syndrome (SVS).36 In pediatric patients, closure of the sutures, attainment of erect posture, growth, and aging are all additional situations in which the opening pressure of the valve may require adjustment.33 A randomized clinical trial did not demonstrate any survival benefit of the Codman-Medos programmable valve (Codman & Shurtleff) over standard valves.37 In a prospective study examining the Strata valve (Medtronic Neurosurgical), 315 patients undergoing first shunt insertion (n = 201) or shunt revision (n = 114) received the valve. One-year shunt survival after first insertion was 68% and after shunt revision was 71%. These shunt survival data are similar to those published for other valves. Valve adjustments resulted in complete resolution of symptoms in 26% of the patients and an improvement in the symptoms of 37% of the patients. When symptoms improved or resolved, they did so within 24 hours in 89% of the adjustments that were made.38 Although a clear advantage to adjustability has not yet been demonstrated in terms of shunt survival, many surgeons find this feature desirable in an attempt to relieve symptoms, maintain large ventricles, or deal with small fluid collections (▶ Fig. 10.6). The ability to adjust the valve pressure noninvasively, and thus potentially minimize subsequent operative manipulations of the shunt system, may warrant the increased expense and complexity of the programmable system.28,33,35,37
Fig. 10.6 (a) Subdural collections caused by overdrainage (b) can often be managed successfully by percutaneously increasing the valve pressure of a programmable valve until the subdural collections have resolved.
10.4.3 Reservoir
A reservoir is very commonly used; it may be incorporated as part of the valve or added separately. It is usually placed near the valve or at the bur hole. It is useful for access to the CSF for diagnosis of infection and occasionally for removal (or attempted removal) of CSF in emergent situations.
10.4.4 Peritoneal Catheter
Peritoneal catheters are also made of Silastic and impregnated with barium to make them radiopaque. The length is suitable for adult insertion, but the catheter can be shortened for use in children if necessary. In most cases, a full-term baby can accept nearly the full length, so elective lengthening is unlikely to be necessary. The catheters have an open distal end, and some have distal ports on the side. Peritoneal catheters are also available with a closed distal end and slits in the side of the tubing (distal-slit valves), which function in a differential-pressure fashion. In a review of 1,719 patients from Toronto and Paris, Sainte-Rose et al found that distal slit valves had a significantly higher failure rate than proximal non-slit valves.25
10.4.5 Antibiotic-Impregnated Shunt Systems
Antibiotic-impregnated shunt (AIS) systems are designed to reduce bacterial colonization and the risk for infection. An AIS system was evaluated in a prospective, randomized clinical trial to determine whether it reduced the incidence of shunt infections compared with standard shunts. After a median follow-up of 9 months, 10 of 60 patients in the control group and 3 of 50 in the AIS group developed infections (p = 0.08).39 Parker et al published a systematic literature review with 12 studies comparing AIS and non-AIS systems.40 Across these studies, 5,613 patients were identified with reported shunt procedures: 2,664 patients who received AIS systems and 2,949 patients who did not. The rate of shunt infection in pooled AIS patients was 3.3%, whereas the non-AIS patients had a rate of 7.2% (odds ratio [OR], 0.439; p < 0.0001). These data favor the use of AIS systems; however, large randomized, prospective studies are needed to truly ascertain the absolute risk reduction obtained by using AIS systems.
10.4.6 Choosing the Appropriate Equipment
The amount of evidence that is available for choosing shunt equipment is limited. Probably the best advice is for a surgeon to become familiar with one system and use it consistently. Our preference is to use a straight ventricular catheter with a snap-on reservoir. A ventricular catheter integrated into the valve is not used, so that blood and debris can be drained away before the ventricular catheter is hooked up to the valve. An open-ended peritoneal catheter may or may not be integrated with the valve. A reservoir may be used as part of the valve system for access to CSF.
The choice of a valve has commonly been an area of great interest and controversy. A multicenter trial has compared a standard differential-pressure valve, a siphon-resisting valve (Delta valve; Medtronic Neurosurgical), and a flow-regulating valve (Orbis Sigma valve; Cordis Corporation) for children with newly diagnosed hydrocephalus.5 No significant difference was found in the time to first shunt failure among the three systems (▶ Fig. 10.7). Furthermore, as previously mentioned, clinical studies have not demonstrated a significant survival benefit for the adjustable valves (▶ Fig. 10.8 and ▶ Fig. 10.9) compared with standard valves.37
Fig. 10.7 (a) Overall results of the Shunt Design Trial. At 1 year, 40% of the patients had shunt failure. (b) Results of the Shunt Design Trial by valve. At 1 and 2 years there was no significant difference between valves.
(From Drake, JM, Kestle JR, Milner R., et al. Randomized trial of cerebrospinal fluid shunt valve design in pediatric hydrocephalus. Neurosurgery 1998;43(2):2965. Republished with permission.)
Fig. 10.8 Results of the programmable valve trial. Percentage of first shunt systems with no surgical intervention as a function of time from implantation in the experimental (Codman-Hakim programmable valve; Codman & Shurtleff) and control groups (conventional valve system). (a) Patients with no prior history of shunt placement. (b) Patients with a history of one or more previous shunts before study entry. Differences between the curves were not statistically significant (p > 0.05).
(From Pollack I, Albright L, Adelson PD, et al. A randomized, controlled study of a programmable shunt valve versus a conventional valve for patients with hydrocephalus. Neurosurgery 1999;45(6):1402. Republished with permission.)
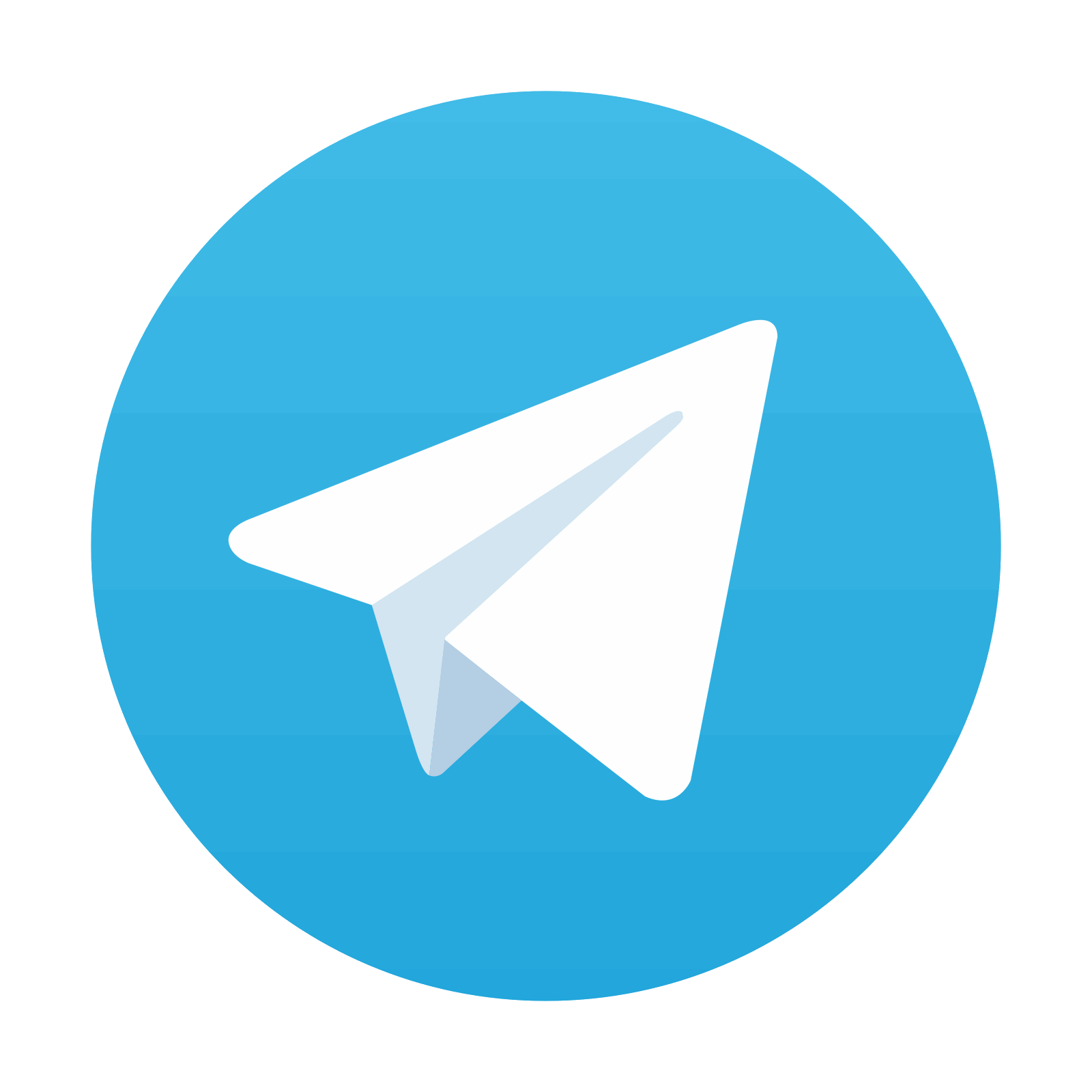