Fig. 1.1
Hand motor fMRI activation in a patient with two contrast enhancing tumors that both displace the motor gyrus and efface the central sulcus. The red line indicates the position of the central sulcus
An area where functional imaging for neurosurgical planning excels is foot motor localization. In contrast to the more lateral hand representation, the foot motor region is less well defined anatomically (as the central sulcus sometimes meets the midline and sometimes does not). As such, foot motor can be difficult to predict precisely using anatomy alone, more so in the presence of tumor. Further, mapping of foot motor cortex by intraoperative direct cortical stimulation within the interhemispheric fissure is often infeasible due to limited access beneath the sagittal sinus. Functional MRI is therefore well suited to aid the surgeon in pretreatment planning when a tumor involves or is in the proximity to the foot motor area. A recent study showed that raters who were experienced with fMRI were significantly better at predicting the location of the foot motor area than raters without [15]. This study suggests that even when anatomical landmarks are present, they are not always sufficient to predict more medial motor regions.
The tongue motor region is located in the lateral extremity of the motor homunculus. Anatomically, the central sulcus truncates as it moves inferiorly and can be difficult to extrapolate from more superior/medial localizations (Fig. 1.2). Thus, if a tumor approaches the inferior aspect of the motor gyrus, tongue motor mapping with fMRI may be informative. Because tongue motor cortex is positioned just posterior to Broca’s area in the dominant hemisphere, it is often mapped in combination with language and in anticipation of confirmation by direct cortical stimulation intraoperatively. In this context, preoperative tongue motor localization may be useful to guide this portion of intraoperative mapping by providing a starting point where clear speech arrest is expected.


Fig. 1.2
Tongue motor fMRI in a patient with a low-grade glioma. It can be difficult using anatomy on MRI alone to determine the location of inferior motor regions such as face/tongue
In addition, the tongue motor region is bilaterally represented. So, while resection of the tongue and face motor region will result in central facial paresis and/or dysarthria, these deficits are often transient and recoverable [16]. Therefore, if fMRI can demonstrate that a tumor is relatively confined to the tongue motor region, it may help guide the decision for surgery.
Sensorimotor areas have been successfully localized using RS-fMRI in the brain tumor patient. Kokkonen et al. localized sensorimotor cortices in both brain tumor patients and control subjects using RS-fMRI and found no differences in volume, spatial correlation, or temporal correlation [17]. And yet other studies have also validated RS-fMRI with direct cortical stimulation [4]. Mitchell et al. developed an algorithm to identify language and motor networks, validated in tumor patients undergoing both presurgical fMRI and intraoperative direct cortical stimulation [18]. The RS-fMRI AUC for motor networks when analyzed pairwise with electrocortical stimulation positive sites was 89. This finding has been supported by other studies suggesting that motor gyrus localization for presurgical planning is particularly feasible using resting state fMRI [19]. As such there is little argument that RS-fMRI may prove useful in localizing primary sensory networks even in the presence of a brain tumor. Of note, there is literature to suggest that motor mapping using fMRI can be performed with accuracy in the anesthetized patient as well, offering a potentially valuable adjunct for functional mapping in pediatrics and in adult populations with limited capacity for task participation [20].
Supplementary Motor Area
fMRI is commonly used to localize the SMA a functional designation attributed to the posteromedial superior frontal region in both hemispheres. This region is particularly amenable to fMRI localization for glioma surgery as there are no well-defined anatomical boundaries. The SMA is segregated into functionally specific regions with the anterior aspect planning the motor output of language function and the more posterior region subserving motor planning. Evidence has also suggested both somatotopy within the SMA [21] and a central region between the language and motor components of the SMA with shared functionality [22]. The preoperative localization of tumors in relation to both SMA and the motor gyrus may be useful in order to predict and interpret postoperative deficits. SMA injury is associated with a syndrome of temporary paresis and dysphasia that can mimic effects of injury to primary motor and language cortices, but which is distinguished by a high potential for recovery within weeks [23]. The mechanisms underlying SMA syndrome (and its transient nature) are not clear. A recent study using RS-fMRI suggested that interhemispheric connectivity decreases in the immediate postoperative period and ultimately interhemispheric SMA connectivity rises to higher levels than preoperative measurements. This gives rise to the possibility that SMA syndromes may result from disrupting interhemispheric connectivity [24].
Language fMRI
Applications of fMRI language mapping in the brain tumor patient can be segregated into evaluations of language laterality and language localization.
Language Lateralization
Using fMRI to lateralize language was historically seen as a welcome alternative to the intracarotid amobarbital procedure (IAP or “Wada” test). During the IAP, the patient is tested for language and memory task performance while each hemisphere of the brain is anesthetized separately via angiographically directed injection of individual carotids or selected arteries. This invasive test carries a small risk of significant complications including stroke [25]. Multiple studies have investigated the concordance between fMRI and IAP language lateralization. A meta-analysis of twenty-three studies showed that the sensitivity and specificity of fMRI for atypical language dominance (using IAP as the standard of reference) were 83.5–88.1%, respectively [26]. This study also showed that fMRI and IAP showed at least 80% concordance between the two tests in 21 of 23 studies. A separate study reported 10 patients who underwent anterior temporal lobectomy for epilepsy in whom preoperative fMRI and IAP lateralization were discordant. Postoperative language deficits were more accurately predicted by the fMRI result in 7 of the patients, and IAP more predictive in 2 [27].
Discordance between IAP and task-based fMRI is not surprising, given the significant variability in both IAP and fMRI task selection, and MRI image acquisition, analysis, and interpretation. In an attempt to address fMRI task variability, Rutten et al. have proposed a combined task analysis wherein multiple language task runs are concatenated and only activations in common with all language tasks are represented in the output [28]. In this procedure, task-dependent activations fall away and, arguably, only language essential regions remain. Combined task analyses were more concordant with IAP than their individual counterparts in all cases in this study.
A second cause for discrepancy between fMRI and IAP results is the relatively high granularity of fMRI mapping and its sensitivity to nondominant hemispheric activation leading to more cases of fractional laterality indices [29]. It remains to be seen whether fractional laterality indices are predictive of deficits.
Lastly, differences between techniques may partly account for the discordance that is seen not only between WADA and fMRI, but also between DCS and fMRI. For example, most language fMRI is acquired with the patient performing tasks silently in order to avoid head motion, whereas intraoperative mapping involves the arrest of vocalized speech. Our group showed that when tongue movement is added to the silent speech fMRI mapping protocol, the region of speech arrest found using DCS encompasses the fMRI prediction in a way that it does not with silent speech tasks alone [11]. Mismatches like this have driven refinement of intermodality testing protocols.
Language Localization
Localization of peritumoral activations comes with its own set of caveats. Localizing language may assist the neurosurgeon in planning a trajectory to a tumor. This can be helpful information because both intra-axial and extra-axial tumors can displace function.
It is important and appropriate here to note that fMRI activations are thought to represent local field potentials that include both excitatory and inhibitory components [30]. Some have even argued that the LFP is a combination of local and distant sources [31]. Related, a language fMRI map (and any other fMRI signal) is comprised of both essential and supportive activations. An essential activation can be defined as a region critical for a task and cannot be resected without significant deficit. A supportive function, if resected, may impart a minor deficit or none at all. fMRI maps of language cannot distinguish between the two, and as a result, DCS is often performed in order to confirm which fMRI localizations are critical and which are expendable in the service of the most complete resection possible (Fig. 1.3).


Fig. 1.3
Language fMRI (Phonemic Fluency) in two patients with very similar low-grade gliomas. The patients are similar both in the anatomical location of their tumors and in the fMRI activation patterns in the middle frontal gyrus. The patient on the left demonstrated speech disruption upon direct cortical stimulation of the middle frontal gyrus fMRI activation loci in the operating room and the patient on the right did not. This is a key example of how what is essential fMRI activation for one patient is not for another
Technical Considerations
There are many technical malignant considerations in the use of fMRI in malignant glioma patients. Of these, abnormal neovasculature that is inherent to many glial tumors is one of the most significant. High-grade gliomas in particular have been shown to decouple the BOLD fMRI signal from behavior through dysautoregulation [32]. In such cases, the measured BOLD response lags the expected response when compared to the timing of the stimulus presentation. If not carefully identified, such an error can lead to false negatives.
Additionally, dysautoregulation poses a particular risk to language lateralization studies as lateralization is based on a ratio measure (# Left Hemisphere Voxels-# Right Hemisphere voxels/# Left Hemisphere Voxels + # Right Hemisphere voxels). Ulmer et al show that tumor suppression of the BOLD signal can make contralateral fMRI speech signals take on more significance in the ratio determination of hemispheric dominance for language. As such, it can erroneously lead to what the authors’ term pseudodominance [33]. Further, decoupling has been shown to affect RS-fMRI as well as it is derived from the same vascular signal [34]. The use of perfusion imaging to identify patients with tumors that are highly perfused may contribute by identifying those fMRI scans that are at high risk for false negatives in regions that are hyperperfused. Therefore, fMRI in low-grade tumors where abundant abnormal neovasculature is less burdensome may be more reliable.
Another technical consideration worth mentioning is vascular density. Vascular density differs between brain regions and will affect spatial specificity. For example, the temporal lobe shows regions of both rich and sparse capillary bed density. This variable distribution of capillaries will affect spatial specificity and may yield false-negative estimates of function [35]. To the authors’ knowledge, this physiologic limitation has not been shown to have direct consequences clinically but it is worth noting as a potential limitation.
While head motion is an issue for any fMRI study, it is of particular concern in impaired patients. Anything that changes the gray-scale value of the fMRI volume registers as a statistical difference to most analysis software programs if it occurs at the right time. If a patient were to move their head every time, they attempted to move a paretic limb; for example, the resultant fMRI map may represent that stimulus-locked motion and not BOLD perfusion itself. Additional concern is warranted to use of RS-fMRI clinically as multiple studies have suggested that poor motion correction can lead to false-positive network correlations [36, 37].
Diffusion Tractography
Whereas fMRI measures changes in gray matter perfusion, diffusion tractography (DTI) uses water diffusion to estimate white matter tract directionality. Water diffuses parallel to axonal fibers and is restricted in the perpendicular direction. This directionality is termed anisotropy [38]. With six or more MR gradient measurements, a diffusion tensor (a vector measure) can be calculated. From the diffusion tensor, the fractional anisotropy (FA) (a scalar measure) ranging from zero to one represents the degree of directionality of a tract. FA is used to infer tract density and demonstrates anatomical connectivity (as opposed to functional connectivity that we saw with RS-fMRI). FA maps are color-coded 2D visual representations of directional water diffusion (Fig. 1.4), whereas tensor maps are their 3D counterparts (Fig. 1.5). Some of the most common tracts mapped in the treatment of gliomas are the cortico-spinal tracts (motor), the arcuate fasciculus (language), and the visual projections. DTI provides more sensitivity to tract disruption than routine MR imaging where white matter tracts can appear normal [39].



Fig. 1.4
Directionally encoded color FA map in a glioma patient. Red left to right, Green anterior to posterior, Blue inferior to superior (courtesy of Dr. Kyung Peck, MSKCC)

Fig. 1.5
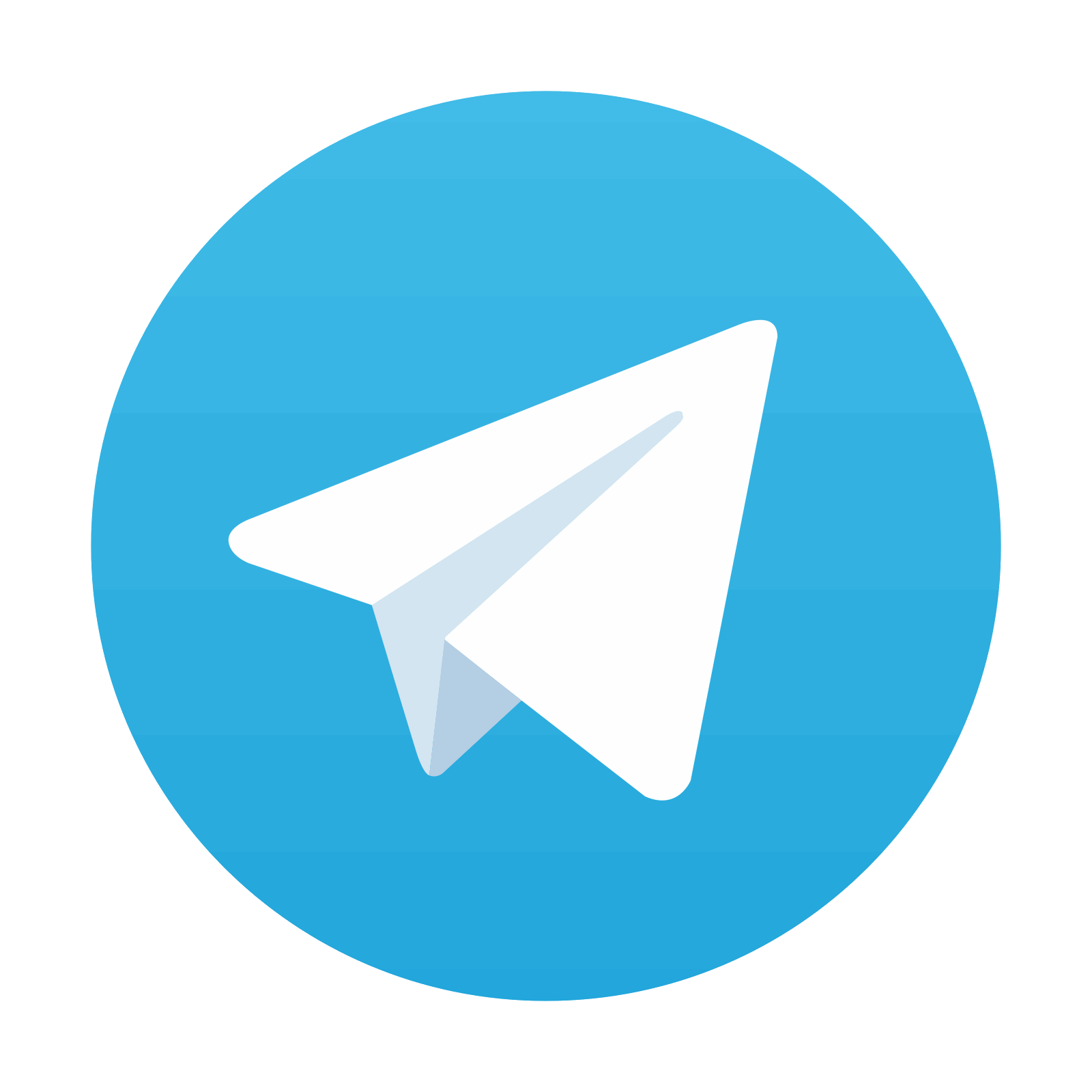
Cortico-spinal tractography in a glioma patient imported into the neurosurgical navigation system. In this case, the cortico-spinal tract is estimated and converted to a 3D object for intraoperative dynamic navigation
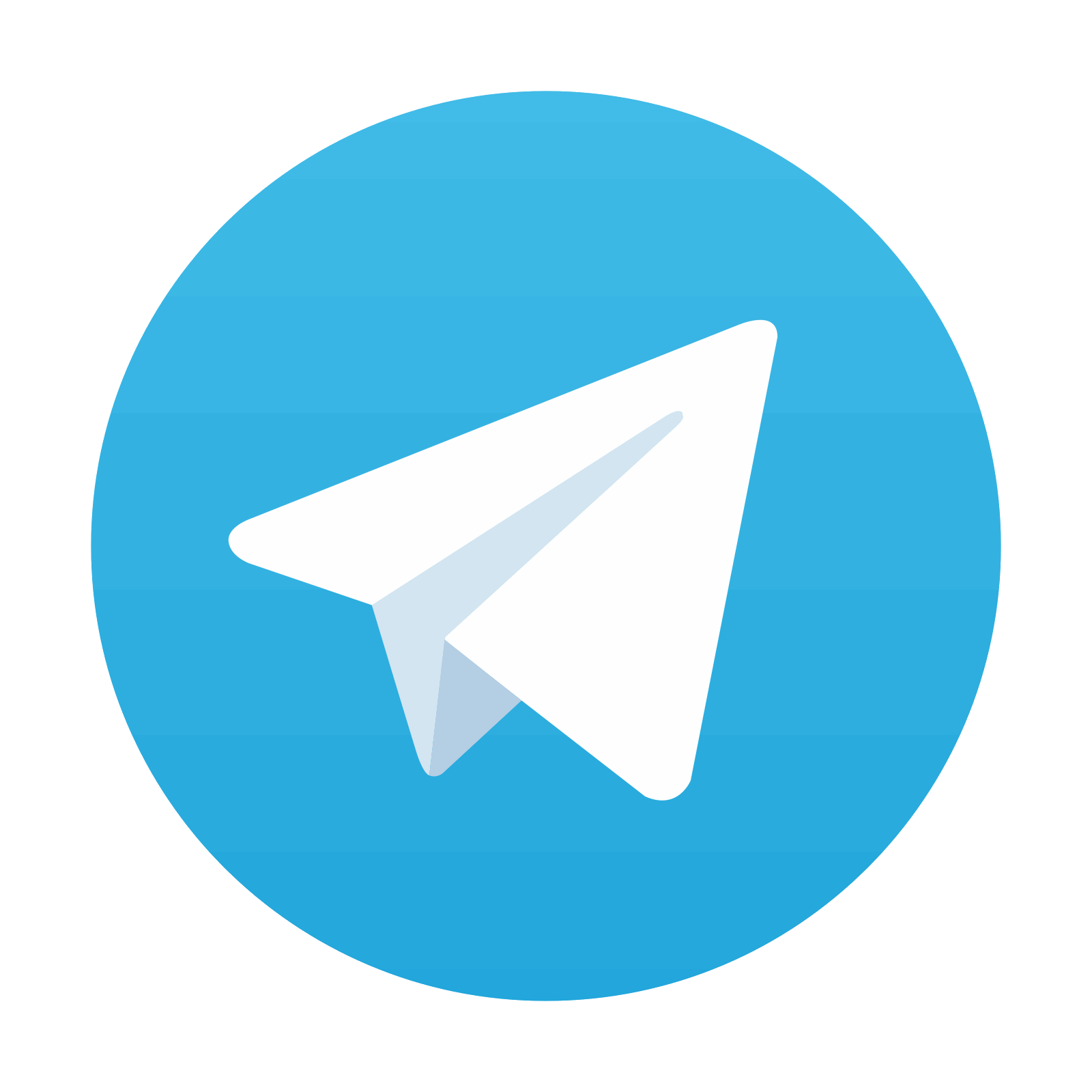
Stay updated, free articles. Join our Telegram channel

Full access? Get Clinical Tree
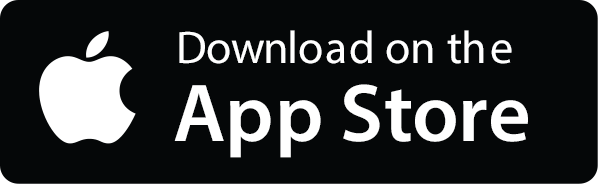
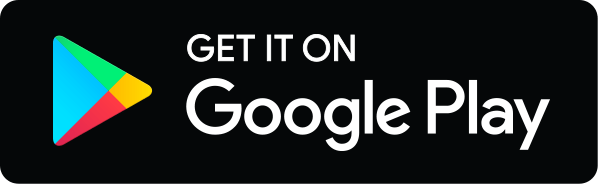
