18 Asleep Motor Mapping
Abstract
Over the years, neurosurgeons have been developing ways to offer safe maximal resection of lesions, such as cerebral gliomas, adjacent to eloquent cortex via supratentorial craniotomy. In addition to advances in neurosurgical technique, a new age of technology over the past decade has ushered in the innovation of intrasurgical electrostimulation mapping, which has permitted neurosurgeons to operate corresponding to the functional boundary. In this chapter, we will discuss the nuances of intraoperative brain mapping, from preoperative planning to selecting the appropriate method to allow for identification and preservation of the motor cortex for safe, gross-total resection of the identified lesion, during general anesthesia craniotomy.
18.1 Introduction
As technology has advanced over recent years, in addition to functional imaging, intraoperative brain mapping techniques have been used widely in patients who undergo craniotomies either awake or under general anesthesia. 1 Unlike functional localization in an awake craniotomy, brain mapping under general anesthesia for tumor resection in eloquent areas requires a more detailed process. Intraoperative somatosensory-evoked potentials (SSEPs) phase reversal and electrical stimulation mapping have been the preferred methods for guiding neurosurgeons to optimize gross total resection, in order to reduce tumor burden and increase survival. 2 At the same time, care must be taken to avoid resultant postoperative neurological deficits, since there is a lack of patient interaction and feedback under general anesthesia as maximal territory is targeted for resection.
18.2 Motor Pathways
The primary motor cortex is an area of the brain located in the frontal lobe in the precentral gyrus and is of principal importance in motor function. This area generates neural impulses that progress along a complex pathway to produce neural excitations, which originate in giant pyramidal cells, known as Betz cells, leading to movement. 3
Signals travel along this tract and cross the midline, and, as such, signals from the right hemisphere control motor activity on the left side of the body. In addition, the body is represented in a somatotopic arrangement, with a specific amount of brain matter devoted to a body part. Additional areas of the cortex also influence motor functions, such as the posterior parietal is involved in interpreting visual information for motor guidance, and the premotor cortex, just anterior and lateral to MC, is involved in sensory guidance of movement and control of truncal and proximal limb muscles. Associated with the premotor cortex are the cingulate motor area (CMA) and the supplementary motor area (SMA). 4 The SMA is medial and superior to the premotor cortex and is involved in planning complex and dual-handed movements. Neurons from the cortex compose pyramidal tract fibers. These fibers project into well-defined portions of the postcommissural putamen and the subthalamic nucleus (STN). 4 Motor cortex projects to the dorsolateral STN, the SMA, and the premotor cortex, and the CMA projects to the dorsomedial STN. 4 These upper motor neurons (UMNs) send signals from the cerebral cortex to the midbrain and medulla oblongata. The UMNs of motor cortex, via the corticospinal tract, descend to the posterior limb of the internal capsule, through the crus cerebri, to the pons and then to the medullary pyramids where the majority of the axons cross the midline to the contralateral side at the decussation. 3 At this point, they descend as either the lateral corticospinal tract which is responsible for controlling appendicular muscles or the anterior corticospinal tract which is responsible for controlling muscles of the trunk. 3 The anterior corticospinal tracts do not cross in the medulla, instead descending ipsilaterally in the brainstem and entering spinal cord, synapsing at that level with lower motor neurons (LMNs). 3 The lateral corticospinal tract synapses with ventromedial LMNs of the spinal cord. These LMNs innervate the skeletal muscles. The corticobulbar tracts follow a similar path as described above, except when reaching the medulla, the fibers do not cross, remain ipsilateral, and exit at the level of the brainstem to synapse on LMNs of cranial nerves (CNs), specifically the nonocular central nervous system. 3 Fibers of the corticobulbar tract are also involved in sensory information, ending in sensory nuclei of the brainstem, for example, the gracile nucleus. 3
18.3 Indications
Neurophysiologic brain mapping offers live assessment of cortical and subcortical function to increase specificity in identification of the essential regions of the brain. It is crucial for the neurosurgeon to determine the right corridor to resect tumors that are in eloquent areas and preserve function (Fig. 18‑1). In order to determine the eloquent area, such as primary motor cortex, primary somatosensory cortex, language areas such as Broca’s and Wernicke’s, and visual areas, brain mapping must be done. Of these modalities, only motor mapping may be performed under general anesthesia, as all other forms of mapping inherently require the patient to be awake to participate in providing feedback during testing.

Asleep motor brain mapping is indicated for those who have pathologic lesions (vascular, tumor, epileptogenic focus), and who are not able to engage reliably or productively during an awake craniotomy. This may include patients with comorbidities such as anxiety, developmental delay, or any form of preclusion from communication. Asleep mapping may be contraindicated in persons with medical issues that would make conversion to general anesthesia challenging, such as obesity, obstructive sleep apnea, or any condition creating a difficult airway. 5
18.4 Preoperative Imaging
It is important for one to be comfortable recognizing the anatomy of regions in question, thereby allowing for conservation of the eloquent areas, including the primary motor cortex. Radiographic review of neuroanatomy on a computed tomography (CT), CT angiogram (CTA), magnetic resonance imaging (MRI), MR angiogram (MRA), and catheter angiography has traditionally been fundamental in aiding preoperative planning in neurosurgery. The above radiographic forms can be utilized in this planning phase, and each offers its own varied aids and advantages compared to the others during this process. Plain CT reveals the overall brain matter, gyral pattern, abnormal attenuation, and mass effect. It also provides especially important views of the cranial vault for any tumor involvement, destructive lesions, or presence of hemorrhagic tumor core.
In 1982, Lee and colleagues first reported the use of an intraoperative CT scanner both in morphologic and in functional stereotactic surgery, and later the use of MRI in selected functional and tumor cases. 6 Localizing the lesion can be approached in a stepwise manner using known anatomical landmarks. On plain CT and T1- and T2-weighted MRI sequences, the superior frontal sulcus posteriorly is seen to end at the precentral gyrus, where on axial imaging, the “omega sign” correlates with the motor hand area on each side, and anterior to the marginal ramus of the cingulate sulcus, which when taken in view bilaterally forms the “pars bracket sign,” is the central sulcus (CS; Fig. 18‑2). 7

CTA is a noninvasive way to visualize a tumor’s feeding vessels, as well as illustrate the surrounding vasculature, and plays an important role in preoperative planning and surgical approach. However, though CTA has a high degree of specificity in regard to identifying the vasculature, the “gold standard” remains cerebral angiography. MRA offers the same visualization benefit as CT without the risk of radiation exposure or intravenous contrast use, thus making this modality a better alternative for those patients with contraindications to intravenous contrast and radiation, such as, but not limited to, those with a prominent radiographic contrast allergy, or compromised kidney function; though the risk of nephrogenic systemic fibrosis with renal failure may preclude the use of gadolinium that at times may provide additional detail beyond the noncontrast time-of-flight or phase-contrast MRA techniques.
Blood-oxygen-level-dependent functional MRI (BOLD fMRI) is used to localize regions of sensorimotor or language functions when one is actively performing tasks. 8 This is elucidated by detecting the amount of deoxyhemoglobin (paramagnetic compared to oxyhemoglobin) in the capillary and venous structures in brain regions responsible for carrying out movement, sensory perception, or speech generation while said actions are performed. 7 This is feasible, because while oxygen extraction from hemoglobin is increased in activated brain tissue, the amount of increased oxygenated blood flow and volume in response to activity is disproportionately greater, leaving a lesser amount of deoxyhemoglobin in the regions sampled compared to times of inactivity. However, according to Glover, BOLD fMRI faces limitations with low temporal resolution, signal dropout, and/or spatial distortion in frontal, orbital, and lateral parietal regions. This is caused by the approximately 9 ppm difference in magnetic susceptibility at interfaces between air and brain tissue, resulting in lack of BOLD signal in ventral, temporal, and prefrontal cortex regions. Moreover, poor data quality can also result from excessive movement and poor task performance. The scanner’s loud noise associated with switched magnetic fields can cause deranged results in studies of audition and resting state networks, though this can be combated with certain techniques. 9 , 10 The most staggering limitation of fMRI has been in language lateralization, with poor sensitivity noted (only 22% in naming tasks and 36% in verb generation tasks). 7
Other imaging techniques, including diffusion-weighted imaging (DWI) and diffusion tensor imaging (DTI), are noninvasive MRI sequences that allow preoperative visualization and incorporation of the pyramidal tracts for intraoperative navigation to facilitate localization of lesions in eloquent areas. 7 It is essential to consider that while utilizing neuronavigation during the surgical procedure with the use of mannitol and dexamethasone intraoperatively, changes in tissue volumes and amount of brain shift can alter the correlation between real-time visualization and preoperative images. 7 Therefore, it is essential to keep this phenomenon in mind while utilizing neuronavigation during the surgical procedure. In addition, one must also account for the brain’s ability to undergo anatomical and functional reorganization to compensate for pathological damage, that is, brain plasticity. 11 , 12 Thus, the normal eloquent anatomy identified in preoperative images might not undertake the same function anticipated in case of lesions that have been growing in eloquent areas. DTI and DWI sequences purely provide preoperative prediction of pyramidal tracking, which can be superimposed with real-time, intraoperative mapping, providing real-time detection of the eloquent functional relocation, ensuring a safe and optimal resection. 7
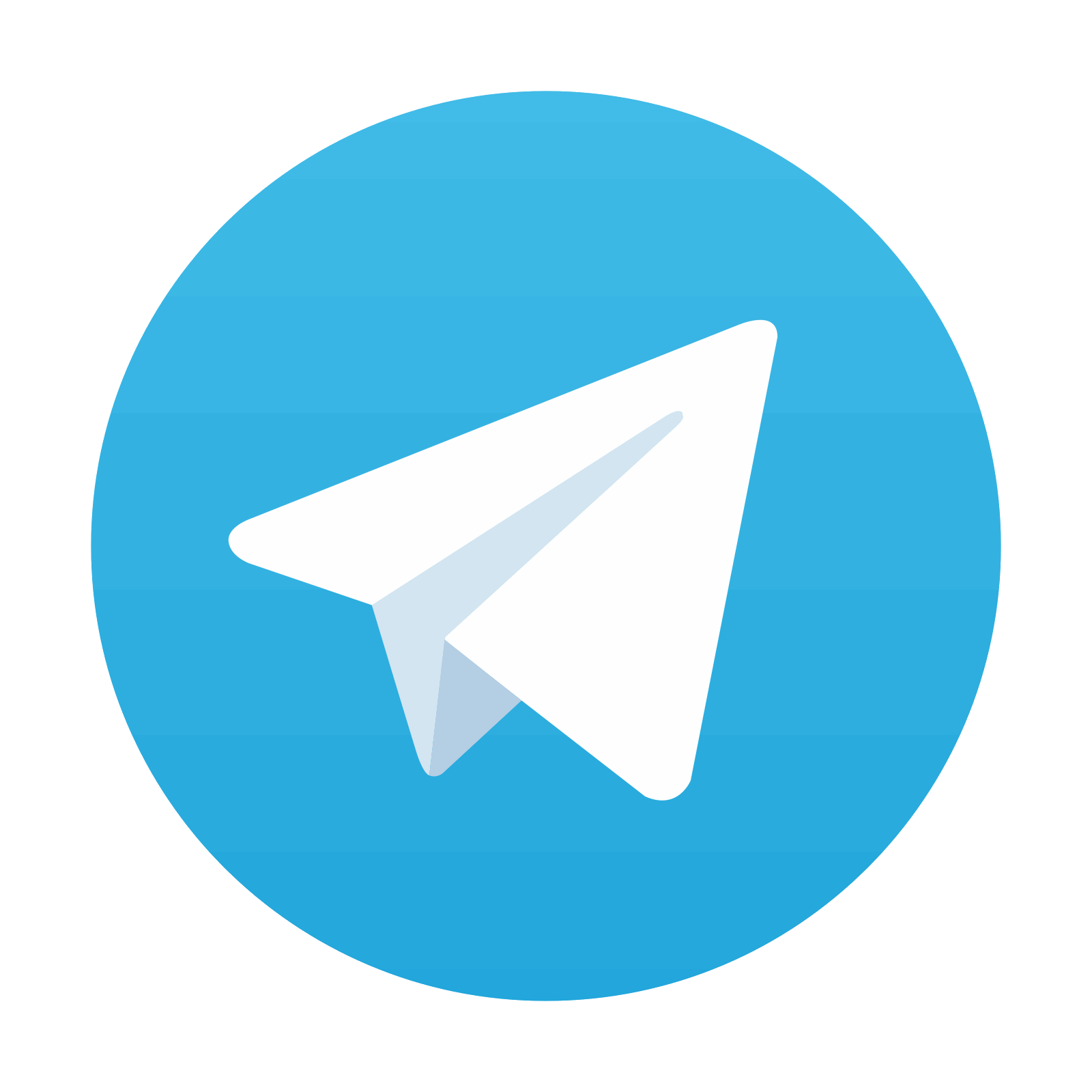
Stay updated, free articles. Join our Telegram channel

Full access? Get Clinical Tree
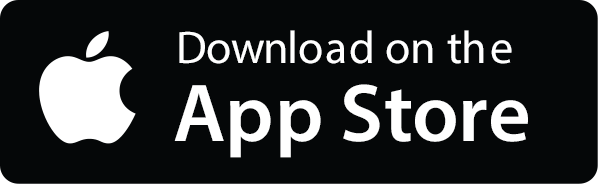
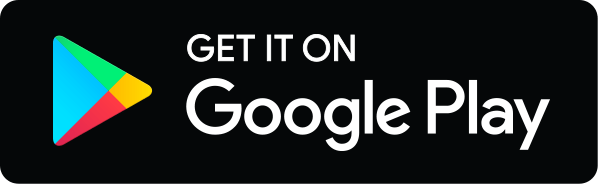