19 Brainstem and Spinal Cord Mapping
Abstract
During the past few decades, the continuous advancement of intraoperative neuromonitoring and mapping (IONMM) techniques have been pushing forward the limits of surgical resection of brainstem and intramedullary spinal cord (IMSC) lesions. Monitoring modalities such as brainstem auditory evoked potentials, somatosensory-evoked potentials, motor-evoked potentials, D-wave recordings, and free-running electromyography have provided real-time monitoring of the functional integrity of neural tissue, and mapping techniques using hand-held probes and modified surgical instruments allowed for accurate identification of important neuroanatomical structures, paving the way for extensive surgical resection of brainstem and IMSC lesions without compromising neurological function and patient outcomes. The sensitivities and specificities of these modalities in detecting impending neural injury significantly increase when multiple modalities are combined rather than utilized individually. The single most important factor that determines the success of IONMM is employing a multidisciplinary and team-based approach, where open communication between the surgeon, neurophysiologist, and anesthesiologist allows for identifying and controlling possible contributors to changes in the IONMM signal and preventing neural damage before it becomes irreversible.
19.1 Introduction
Surgical procedures targeting brainstem and intramedullary spinal cord (IMSC) lesions remain among the most challenging interventions, even after decades of neurosurgical advancements, detailed neuroanatomical descriptions, and optimization of microsurgical techniques. Gross total resection (GTR) is not always possible, and the risk of postoperative complications is relatively high compared to other anatomical locations. Conservative approaches sometimes deter the surgeon from resecting the tumor, thus limiting his or her surgical options to performing an excisional biopsy. Although most cases cannot be cured by surgical resection alone, since other interventions (chemotherapy, radiotherapy, etc.) have failed to show significant benefit on long-term follow-up, achieving maximal safe resection with minimal tumor residual load is still the best initial step for oncological treatment in many cases. This understanding, among others, created motivation for the development of intraoperative neuromonitoring and mapping (IONMM) techniques over the past three decades, encouraging the surgeon to more aggressively, yet safely, resect brainstem and IMSC lesions with better patient outcomes. The goal of this chapter is to provide a brief overview of the IONMM modalities that are utilized during surgeries for resection of brainstem and IMSC lesions as well as to highlight the roles and significance of effective communication between the neurophysiologist, the surgeon, and the anesthesiologist, allowing for safe maximal resection of these challenging lesions.
19.1.1 Pathophysiology of Surgically Induced Nerve Injury
Nerve injury can be broadly categorized into demyelinating and axonal (Fig. 19‑1). In the case of demyelinating injury, the axons remain intact and rapid recovery is the rule, usually within a few weeks. On the other hand, axonal injury has a grimmer prognosis since axonal regrowth is slow and often incomplete. At a mechanistic level, early compression initially injures the myelin sheath; if corrective measures are undertaken at this stage, full recovery is the rule. With more severe compression, the axons are eventually damaged, and this may lead to a permanent neurological deficit. Alternatively, ischemic injury is fundamentally different since it affects the axons first, and if corrective measures are not undertaken promptly, it leads to permanent damage. At the earliest stages of ischemia and compression, the axon and myelin sheath are structurally intact but unable to function normally. If the underlying insult is removed immediately, quick recovery is expected within seconds to minutes (Fig. 19‑2). The objective of IONMM is to detect the injury at this very early stage.


19.2 Intraoperative Neuroimaging and Mapping Modalities
In general, the neurophysiological techniques utilized to evaluate the functional integrity of the brainstem and spinal cord may fall into one of two main categories: monitoring or mapping. Monitoring modalities provide continuous, real-time data about the functional status of different portions of the brainstem and spinal cord, whereas mapping techniques are based on using a hand-held probe to identify anatomical structures within the surgical field and prevent iatrogenic neural injury. Recently, different surgical tools such as the suction device and bipolar have been modified to allow for continuous, real-time mapping. 1 , 2
19.2.1 Monitoring Techniques
Brainstem Auditory Evoked Potentials
Brainstem auditory evoked potentials (BAEPs) test the integrity of the auditory pathways from the auditory nerve to the inferior colliculus through a sequence of stereotyped waveforms generated from multiple axonal pathways in the auditory brainstem. These waves are evoked by auditory stimuli and occur within 10 ms in normal subjects. 3 Interpretation of the BAEPs usually involves measuring the absolute latency of the three most prominent vertex positive peaks I, III and V, along with their relative interpeak latencies. Based on our current understanding of the generators of BAEPs and the pattern of BAEP changes, the site of dysfunction may be roughly inferred. The generators of waves I, III, and V are the cochlea/distal auditory nerve, cochlear nucleus/trapezoid body, and the inferior colliculus, respectively. As such, selective loss of wave V is indicative of brainstem injury. On the other hand, abrupt loss of all three waves is seen in ischemia to the cochlea and distal auditory nerve due to injury to the internal auditory artery.
The stimuli are highly recurrent clicks delivered through ear inserts at an intensity of 60 to 70dB above the patient’s hearing threshold. 4 The recording electrode is placed on the ipsilateral earlobe (or mastoid) and referred to an electrode placed on the vertex (Cz according to the International 10–20 System of Electroencephalography [EEG] Electrode Placement). 3 Hundreds of individual trials are averaged to obtain good quality recordings. For more details about the technique and interpretation of the results, please refer to American Clinical Neurophysiology (ACNS) Guidelines: Guideline 9C3 and Petrova et al. 4
Indications for intraoperative BAEPs include surgical resection of lesions involving cranial nerve (CN) VIII or the auditory pathways up to the inferior colliculus, 5 , 6 microvascular decompression for trigeminal/glossopharyngeal neuralgia and hemifacial spasm, 7 , 8 other cerebellopontine angle surgeries involving the use of cerebellar retractors, 9 which may impose an ischemic risk to the auditory nerve from overstretching, as well as surgical resection of brainstem lesions (tumors, arteriovenous malformations, etc.) located between the pontomedullary junction and inferior colliculus. 10 Some authors have also utilized BAEPs in other procedures that may be associated with risk of brainstem injury (e.g., decompression for Chiari malformation 11 ).
BAEPs are sensitive indicators of damage to the auditory pathway, and the absence of wave V at the end of surgery is highly predictive of hearing loss. On the other hand, the predictive value for more subtle changes of wave V is harder to establish and there are no universally recognized warning criteria to date. This is particularly true in acoustic neuroma surgery, where hearing loss tends to occur regardless of the extent of change observed in wave V. It is generally accepted, however, that a 50% amplitude drop and/or a 0.5 to 1 ms increase in wave V latency is predictive of nerve injury. 12 , 13 Nevertheless, these criteria may be too conservative in some types of surgeries, such as microvascular decompression, where the chance of hearing loss is highest when wave V is completely and permanently lost. 14 , 15 As such, the definition of warning criteria should take into account the type of surgery, especially whether the case is an acoustic neuroma or a different nearby lesion. It is also important to note that BAEPs are specific to the auditory tracts and do not detect injury to other nearby structures.
Somatosensory-Evoked Potentials
Somatosensory-evoked potentials (SSEPs) evaluate the integrity of the fast-conducting large sensory fiber pathways, whereas injury to the slow-conducting small fiber pathways that convey pain and temperature cannot be detected. 16 SSEPs are classically recorded by stimulating the median nerve at the wrist (upper extremity SSEP) or the posterior tibial nerve behind the medial malleolus (lower extremity SSEP) using a brief current delivered via a self-adhesive surface electrode. This triggers a sensory potential that ascends through the ipsilateral dorsal column up to the nucleus cuneatus, decussates near the cervicomedullary junction, ascends by means of the contralateral medial lemniscus up to the thalamus, and finally projects to the contralateral parietal sensory cortex. Recording electrodes placed at specific locations pick up this electrical activity at three different levels: peripheral nerve, spinal level, and cortical level, where the latter response is most relevant to IONMM. Averaging of 500 to 1,500 responses is usually necessary to obtain good quality recordings. Consequently, it may take several minutes to update the SSEP data, which is one of the major drawbacks of the technique. This may significantly delay the detection of nerve injury and increase the risk of permanent damage. 17 In addition, SSEPs are very sensitive to electrical power interference. The abundance of electrical equipment in the operating theater can significantly affect the quality of the waves and further prolong the recording time (Fig. 19‑3). For more details about the technique and interpretation of the results, please refer to ACNS Guidelines: Guideline 9D. 18

Compared to baseline values obtained at the beginning of the surgery, a latency delay of 10% and/or amplitude reduction of 50% of the cortical response are considered critical changes in SSEP signals. 19
Trigeminal SSEPs
Trigeminal stimulation and effective recording of long-latency SSEPs have been performed in awake patients with different neurological conditions since the 1970s. 20 However, attempts to record trigeminal SSEP responses in patients under general anesthesia were not as successful as those in awake patients. 21 A recent study was able to optimize the neurophysiological and anesthetic conditions and record stable and reliable intraoperative responses of trigeminal SSEPs. 22 In brainstem surgery, the clinical utility of trigeminal SSEPs is yet to be elucidated and more studies are required to evaluate its significance compared to other IONMM modalities.
Transcranial Motor-Evoked Potentials
Transcranial motor-evoked potentials (TcMEPs) are used to monitor the integrity of the corticospinal tracts (CSTs). Transcranial activation of the motor cortex generates a motor potential that follows the descending motor tracts down to the corresponding muscle. Therefore, normal TcMEPs depend on the integrity of the corticospinal fibers, the lower motor neuron, and the neuromuscular junction. When the motor cortex is exposed (e.g., during brain tumor surgery), MEPs can be elicited by stimulating the motor cortex using epidural or subdural electrodes or a hand-held probe.
Trains of electrical pulses are delivered to the motor cortex using scalp electrodes (needle, corkscrew, or regular EEG cup electrodes) placed at C1/C2 or C3/C4 scalp positions of the 10–10 EEG system. 23 The more dorsomedial C1/C2 montage is the preferred montage for eliciting responses in the lower extremities, whereas the C3/C4 montage is used for the upper extremities. In general, the C3/C4 montage causes more current penetration and is therefore more potent; however, it causes more patient movements. It also has the disadvantage of activating the jaw muscles and, therefore, exposes the patient to bite injuries. 24 Recording is done using surface or needle electrodes inserted into the target muscles. It is advisable to choose muscles with heavy corticospinal innervation such as the intrinsic hand muscles, abductor hallucis, tibialis anterior, or extensor hallucis muscles. Although surgical injury to the CSTs will only affect MEPs below the level of surgery, it is recommended to also monitor MEPs above the surgical level. The latter will serve as a control condition to differentiate between surgically induced injury and other confounding factors 25 (Fig. 19‑4). MEPs are very sensitive to anesthetics and various other systemic factors such as systemic hypotension, anemia, hypoxemia, and hypothermia. 25 When the upper extremities are included, the recordings provide monitoring and protection for the brachial plexus and peripheral nerves (Fig. 19‑5). For more details about the technique and interpretation of results, please refer to Legatt et al. 25


MEPs are polyphasic waveforms and have a latency of approximately 20 ms for the hands and 45 ms for the feet 26 (Fig. 19‑6). MEPs often display significant intertrial variability in morphology and amplitude. Therefore, judging whether any change in MEP amplitudes is related to variability or surgical injury is not always straightforward, and this explains why there are no universally accepted warning criteria. Some programs adopt the “All or Nothing” rule, 26 and others would consider a 50 to 80% drop in amplitude as significant. 27 While a 50% amplitude drop may be significant in cases with perfectly stable MEP throughout the surgery, it could be purely physiological when highly variable MEPs are recorded at baseline (Fig. 19‑7). In summary, latency-based criteria for MEPs are not reliable as stand-alone criteria. 28 Compared to SSEPs, TcMEPs have the major advantage of being instantaneous since no averaging is needed. Thus, data can be updated very rapidly, and injury can be detected very early. However, one disadvantage of TcMEPs is that these cause patient movements, which could be hazardous in some surgical settings. Other issues include specific anesthetic requirements for MEPs such as the need to avoid muscle relaxing agents, which may cause discomfort for the surgeon.


Corticobulbar MEPs
Following the same concept of TcMEPs, corticobulbar MEP (CoMEP) monitoring provides a real-time functional assessment of corticobulbar pathways from the descending corticobulbar tracts to the CN nuclei and CNs, the significance of which is highest during brainstem surgery. A train of pulses is applied to the dorsolateral aspect of the motor cortex using scalp electrodes (C3/C4 29 or C5/C6 30 ). Recordings can be obtained from trigeminal, facial, and bulbar muscles. One drawback of this technique is that given the lateral position of the stimulating electrodes, the CNs can be activated directly by the spreading of current to the base of the skull. When this occurs, the current shortcuts the corticobulbar tracts leading to false negative results. Switching to monopulse stimulation should abolish CoMEPs and help differentiate between these two scenarios. For detailed description of the technique, please refer to Deletis and Fernández-Conejero 29 and Deletis et al. 31
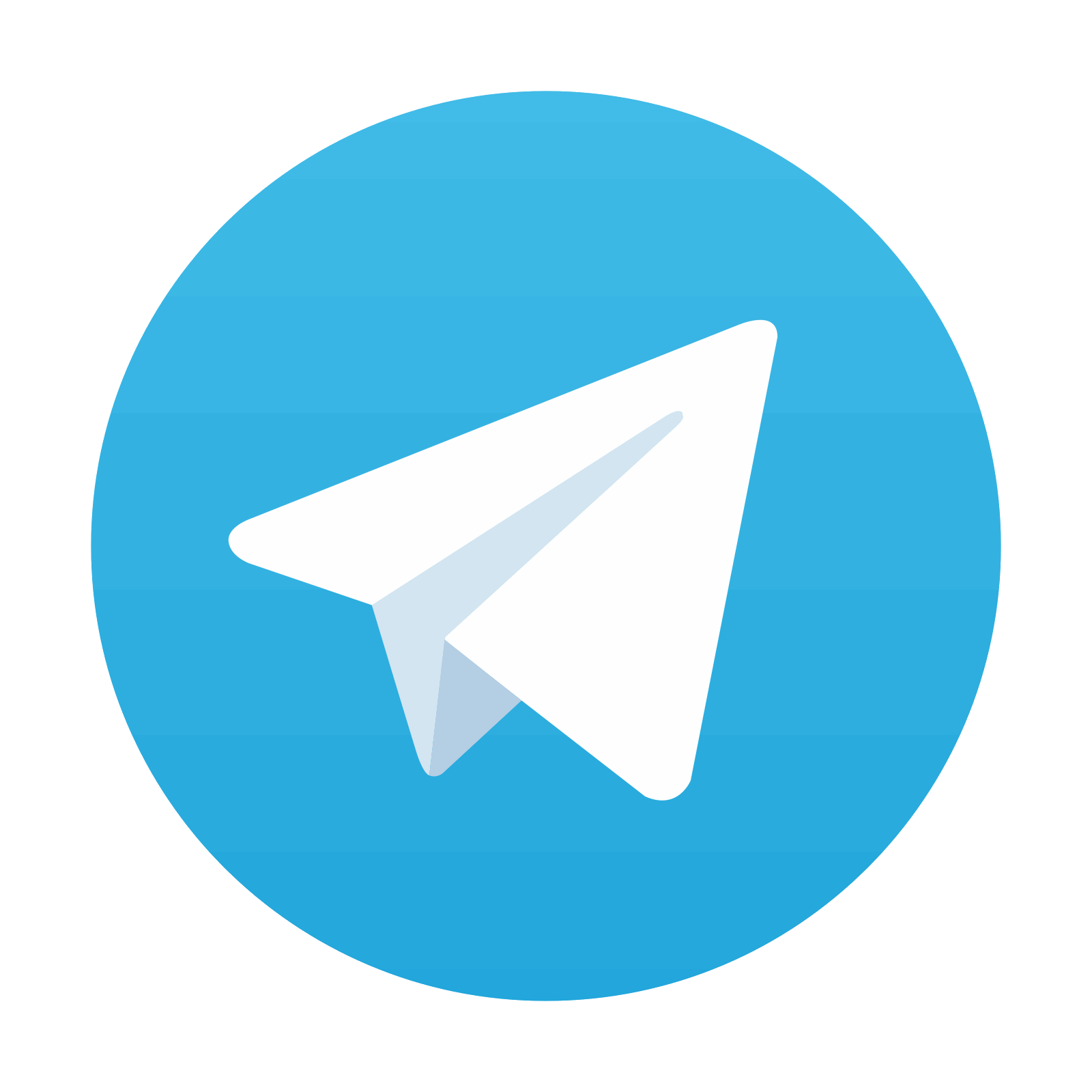
Stay updated, free articles. Join our Telegram channel

Full access? Get Clinical Tree
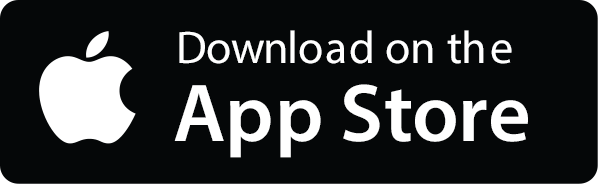
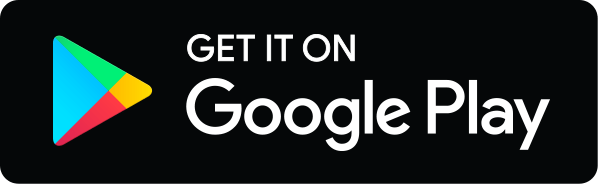
