CHAPTER 40 Psychiatric Neuroscience: Incorporating Pathophysiology into Clinical Case Formulation
PSYCHIATRIC NEUROSCIENCE: DEFINITIONS
Psychiatric neuroscience is one of the most interesting and challenging endeavors in all of medicine.1–5 While a great deal is already known, a wide gap remains between neuroscientific explanations and the clinical phenomena of affect, behavior, and cognition. The brain is extraordinarily complex, and less physically accessible than other organ systems, posing great challenges to researchers. However, recent advances, particularly in neuroimaging and genetics, have provided important tools for tackling these problems. Although progress is difficult, the high prevalence and extensive morbidity and mortality rates of mental illness make progress essential. Mental health practitioners will need to incorporate the lessons from psychiatric neuroscience into everyday practice, and communicate them to patients, families, and the general public.
An important goal of this chapter is to explain that much of the business of psychiatric neuroscience occurs at the molecular level of synaptic function. Psychiatric illness is most often due to microscopic and often diffuse pathological changes that impair synaptic function in multiple regions, while disproportionately affecting certain key neuronal circuits. Therefore, a large portion of psychiatric pathophysiology reflects dysfunctional neural communication. This is in contrast to the many neurological conditions caused by gross pathological lesions. Indeed, even modern-day neurology is based in the nineteenth-century traditions of Paul Broca and the “localizing” lessons of focal lesions. The frequent involvement of macroscopic, focal lesions has led neurologists to focus on clinical neuroanatomy, while the diffuse pathological processes affecting synaptic function have led psychiatrists to emphasize neurochemistry. In some ways, these distinctions are both arbitrary and artificial, and one important challenge of psychiatric neuroscience is to integrate information from all clinical fields interested in the brain.6 Nonetheless, the molecular, synaptic, and nonfocal nature of most psychiatric pathology presents unique challenges to psychiatric neuroscience.
HISTORY OF PSYCHIATRIC NEUROSCIENCE
Psychiatry has a strong neuroscientific tradition. Describing all of the important contributions to brain science made by psychiatric researchers could fill many chapters; here we will cite only a few illustrative examples. Early in the last century, the German psychiatrist and neuropathologist Alois Alzheimer discovered plaques and tangles in the brain of his amnestic patient, Mrs. Auguste D., and provided the first description of the clinical syndrome that now bears his name.7 Together with his colleague and renowned psychiatrist Emil Kraepelin, Alzheimer also described abnormalities in the cortical neurons of patients with dementia praecox, likely representing the first neuropathological studies of schizophrenia.8 Their discoveries have been extended to the molecular level in modern studies identifying abnormalities in γ-aminobutyric acid (GABA)–ergic neurons of the prefrontal cortex.9,10 While Alzheimer’s passion was neuropathology, he also spent many years caring for patients with mental illness. Reflecting on his life’s work he reportedly said that he “wanted to help psychiatry with the microscope.”11
Another historical landmark in psychiatric neuroscience was the demonstration of genetic predispositions to major mental illness.12 Danish adoption studies in the 1960s reported a much greater incidence of schizophrenia in biological as opposed to adoptive relatives of people with schizophrenia, providing key evidence for a significant etiological role of genetics in a psychiatric illness. Other landmark contributions include the work of Julius Axelrod, Ulf von Euler, and Bernard Katz on neurotransmitters and their mechanisms of release, reuptake, and metabolism; their discoveries, recognized with a Nobel Prize in 1970, provide a foundation for much of the content of this chapter.13 In the 1970s, the discovery that antipsychotic medications targeted brain dopamine receptors led to the influential dopamine hypothesis of schizophrenia.14,15 Later, converging work characterizing information processing in the brain at a molecular level earned Arvid Carlsson, Paul Greengard, and Eric Kandel the 2000 Nobel Prize at the end of the decade of the brain.16 These brief highlights emphasize the great progress already attributable to psychiatric neuroscience, and illustrate the great potential for important discoveries in the future.
PSYCHIATRIC DIAGNOSIS: BIOMARKERS AND BIOLOGICAL VALIDITY
In the context of psychiatric neuroscience, the current diagnostic system (DSM-IV-TR)17 has both strengths and weaknesses. A major advance of the post-1980s DSM was the development of diagnostic categories of psychiatric illness with good interrater reliability, largely based on observation and data collection. This provided a firm starting point for scientific investigation, in contrast to previous diagnostic systems based on unproven etiological theories and associated ill-defined terminology. However, the intentional avoidance of etiological theories in generating DSM diagnoses also makes their biological validity uncertain; the extent to which specific DSM diagnoses correspond to specific pathological neural processes is unknown. Unlike most medical illnesses, the vast majority of psychiatric illnesses have so far not been tightly linked to specific biological markers. The descriptive criteria demarcating current diagnoses are likely several steps removed from core pathological processes.
METHODS IN PSYCHIATRIC NEUROSCIENCE
Researchers have adopted a variety of methods for studying the neural mechanisms of mental illness and behavior (Table 40-1). Each of these methods has particular strengths and weakness.
Table 40-1 Methods in Psychiatric Neuroscience
Animal models Brain lesion cases Genetics and molecular biology Neuroimaging Neuropathology Neurophysiology Neuropsychology/endophenotypes Psychopharmacology |
Neuropathology
Neuropathology was clearly in fashion in the late 1800s and early 1900s, when Alzheimer first described plaques in the brain of his patient with dementia,7 and identified frontal cortex abnormalities in schizophrenia.8 While some skeptics have described schizophrenia as the “graveyard of neuropathologists,”18 recent studies have actually provided reproducible descriptions of deficits (such as those in parvalbumin-expressing GABA-ergic interneurons in deep layers 3 and 4, akin to Alzheimer’s findings) in the cortex. These neuropathological findings have provided one of the strongest etiological hypotheses for schizophrenia.9,10
Neuroimaging
Neuroimaging has provided one of the best modern tools for examining the pathophysiology of mental illness in the living brain. As this topic is covered in greater depth in another chapter, we will only briefly summarize it here. Neuroimaging can provide many different quantitative measures, including morphometry, metabolism, and functional activity. Neuroimaging research using groups of subjects can determine whether mental illness is associated with changes in the size or shape of specific brain regions, the functional activity within these regions, or their concentration of particular neurotransmitters, receptors, or key metabolites.19 Although neuroimaging methods can be used to measure cellular and molecular phenomena, the currently achievable spatial resolution still represents an important limitation in examining the microscopic pathological changes implicated in psychiatric illness.
Brain Lesions and Behavior
There is a strong tradition within neuropsychiatry and behavioral neurology of understanding neuroanatomical circuitry by studying the emergent or lost behaviors in patients with focal brain lesions.20 These studies have provided us with a rich view of various brain regions and their relationship to behavior. Perhaps the most famous case is that of Phineas Gage, the Vermont railway worker who suffered a traumatic lesion bilaterally to the medial frontal lobes and developed personality changes.21 Another famous patient (known by his initials) is H. M., who underwent bilateral medial temporal lobe resection for intractable epilepsy and as a result lost the ability to form new memories.22 While striking and informative, findings from these rare cases may be difficult to extrapolate to the pathophysiology of common psychiatric illnesses, which generally do not involve focal lesions. Traditionally, biological psychiatry has relied more on biometrics and quantitative methods; these population-based approaches risk losing insights available from rare cases but are more likely to produce broadly generalizable findings.
Neuropsychology and Endophenotypes
An increasingly important approach in psychiatric neuroscience is to identify and study intermediate phenotypes. These are quantitative phenotypes that are closely associated with the clinical syndrome of interest, but which are less complex and easier to link to the function of specific neural circuits. They can also be used to identify biologically relevant subtypes within a diagnostic category, reducing heterogeneity that may limit the power of scientific investigations. Endophenotypes are intermediate phenotypes that are present both in affected individuals and in their unaffected relatives therefore reflecting genetic risk independent of actual disease. Neuropsychological tests of cognitive function are commonly used as endophenotypes. For example, impairment of working memory, which is closely related to the function of dorsolateral prefrontal cortex, is found within a subgroup of patients with schizophrenia.23 Endophenotypes thus help bridge the gap between brain circuits, which are amenable to study at the molecular and cellular level, and clinical syndromes, which are less tractable. This approach becomes especially powerful when combined with other methods, such as neuroimaging or genetics.24
Neurophysiology
There is a strong tradition within psychiatric neuroscience of studying the electrical activity of the brain and its relation to function. These methods include electroencephalography (EEG), event-related potentials (ERPs), and most recently magnetoencephalography (MEG) and transcranial magnetic stimulation (TMS). Like functional neuroimaging, these modalities provide information about the living, functioning brain. At present, electrophysiological techniques cannot provide anatomical resolution at the level of neurochemistry or synaptic physiology, and are limited to the study of cortical phenomena; however, they can provide excellent temporal and spatial resolution and are invaluable in studying the coordinated function of widely distributed neural circuits. Abnormalities in the timing of oscillations in neural circuit activity have been associated with psychiatric illnesses, and this is an area of intense research activity. For example, the reduction of gamma frequency (30 to 80 Hz) oscillations in schizophrenia has been ascribed to impaired N-methyl-d-aspartate (NMDA) receptor activity on GABA-ergic interneurons.25 These noninvasive methods are particularly heavily used in studies of brain development and function in children.26
Psychopharmacology
More than any other methodology in psychiatric neuroscience, pharmacology has been used to understand the neurochemical basis of behavior and to develop hypotheses regarding psychopathological mechanisms. Famous examples include the dopamine27 and glutamate hypotheses of schizophrenia,28 the catecholamine depletion hypothesis of depression,29 and the dopaminergic models of attention-deficit/hyperactivity disorder (ADHD) and substance abuse. In relating pharmacological effects to potential disease mechanisms, it is important to note that the effects of drugs on clinical symptoms may reflect mechanisms that are downstream of the core pathophysiology, or even unrelated to core disease mechanisms. By analogy, diuretics can improve the symptoms of congestive heart failure while providing less direct insight into its core pathophysiology. Nonetheless, by clearly connecting cellular and synaptic mechanisms with clinical symptoms, pharmacology provides mechanistic tools and information with enormous clinical and scientific utility.
Animal Experiments
In the authors’ opinion, the value of animal experiments has received too little emphasis in psychiatric neuroscience. Clearly, complex psychiatric symptoms (such as delusions) cannot be modeled well in animals, and anthropomorphic interpretations of animal behavior should be taken with due skepticism. Despite these caveats, animal behaviors with known neuroanatomical correlates have been critical in elucidating the neurocircuitry and neurochemistry underlying many psychiatric phenomena. For example, anxiety- and fear-related behaviors have been very productively modeled in animals, leading to a detailed understanding of the role of the amygdala in these behaviors.30 Of course, animal studies also permit a wider range of experimental perturbations than possible with human investigations. Independent of their value as behavioral models, animal models therefore offer the opportunity to explore cellular and molecular pathophysiology in ways that are ethically or technically impossible in human subjects. For example, the Fragile X knock-out mouse is an excellent model for Fragile X syndrome, the most common form of inherited mental retardation. Studying these mice has led to a deep understanding of relevant defects in dendrite formation and neurophysiology.31
Human Genetics and Molecular Biology
Adoption, twin, and familial segregation studies have proven that many psychiatric conditions are highly heritable (i.e., caused in large part by the additive effect of genes).32 Genetic endeavors in psychiatric neuroscience may be broken up into two broad categories: “forward genetics,” or genome-wide attempts to identify genetic loci (genes or their regulatory elements) that underlie susceptibility or contribute to pathophysiology; and “genotype-phenotype” studies, whereby candidate genes are chosen based on a priori biological hypotheses and the degree to which a gene plays a role in a given phenotype is assessed. Such phenotypes may be clinical diagnoses, or endophenotypes from neuropsychology or neuroimaging. The promise for human genetics in psychiatry is tremendous,32 especially for forward genetics, wherein researchers may be led to the core pathophysiology without requiring any a priori hypotheses. Yet human genetics research is exceedingly challenging for various reasons that are beyond the scope of the current discussion. In brief, the genetic architecture of neuropsychiatric conditions is heterogeneous and complex. That is, the majority of psychiatric illnesses likely reflect complex interactions of multiple genes, as well as their interaction with environmental factors that are difficult to assess. Despite these difficulties, there have already been a few notable examples of success.
Analysis of rare, large families with early-onset dementia led to the discovery of mutations in amyloid precursor protein (APP) and presenilins in Alzheimer’s disease (AD).33 In these rare families, these mutations are statistically “linked” to disease and considered “highly penetrant.” However, the vast majority of AD patients do not have mutations in these genes. Indeed, in psychiatry examples of highly penetrant, simple dominant or recessive gene mutations are rare. That is, examples in neuropsychiatry of a particular gene mutation “causing” a specific condition are exceedingly rare and somewhat controversial, and the generalizability of these findings to the common conditions with more complex inheritance is usually unclear. Nonetheless the APP pathway has provided an important target for drug development, which may lead to a medicine that stalls the progress of disease.
Methodologies in molecular genetics and molecular neuroscience also promise improved understanding of gene function in the brain. These methods include the following: comparison of gene sequences in human to nonhuman primate and other animals34; a deeper understanding of how noncoding elements within the genome may regulate important brain genes35 and thereby play a role in psychiatry; the study of gene expression using microarrays36; the study of gene function in mice in which specific genes have been modified by recombinant methods (e.g., “knock-out” or “knock-in” studies)37; and studies examining how experience and the environment alter gene expression.38 In summary, genomics and molecular genetics hold great promise for identifying genes and thus biological mechanisms at the core of psychiatric pathophysiology.
BIOLOGICAL CASE FORMULATION: NEUROSCIENTIFIC CONTENT AND PROCESS
Process
We will consider three brain processes that may lead to illness: neurodevelopment, neurotransmission, and neurodegeneration (Table 40-2). Under neurodevelopment we include related processes that continue into adulthood (such as neuroplasticity and neurogenesis). Synaptic plasticity mediates the effects of experience, learning, and memory on affect, cognition, and behavior. Previously underestimated, adult neurogenesis is now known to continue in select regions of the human brain, most notably the olfactory bulb and the hippocampus. Although the role of adult neurogenesis in humans remains largely unknown, some evidence has connected altered hippocampal neurogenesis to mood disorders.39
Table 40-2 Process in Neuroscientific Explanation in Psychiatry
Processes | |
Neurodevelopment/neuroplasticity | |
Neurotransmission | |
Neurodegeneration | |
Examples |
Disorder or Treatment | Process Type |
---|---|
Attention-deficit/hyperactivity disorder (ADHD) | Neurodevelopmental |
ADHD pharmacotherapy | Neurotransmission |
Alcohol use disorders | |
Neurodevelopmental/genetic | |
Neuroplastic changes | |
Neurotransmission | |
Neurotransmission | |
Neurodegeneration | |
Neurodegeneration | |
Delirium | Neurotransmission |
Alzheimer’s dementia | Neurodegeneration |
Neurotransmission |
Neurodevelopmental processes shaping brain circuits have life-long effects on patterns of affect, behavior, and cognition with direct relevance to mental health. The effects of childhood experience have always been central to psychiatric understanding; psychiatric neuroscience has also attempted to provide a biological grounding for this understanding.38,40 Thus, neurodevelopmental processes include the interacting effects of genes and environment on brain and behavior. Figure 40-1 shows the processes of brain development, including intrauterine neuronal patterning, neurogenesis, cortical migration, gliogenesis, myelination, and experience-dependent synapse modification.41,42 In the first years and decade of life, the brain undergoes a process of synapse formation and pruning.43 Initially, neurons form an overabundance of synapses that are then strengthened and pruned possibly based on experience, learning, or aging (Figure 40-2).
Specific psychiatric disorders may be framed in terms of one or more of these three mechanisms. Autism is an example in which a process of brain development goes awry. At the other end of life, neurodegenerative processes dominate, and can lead to various dementias. Substance use disorders may reflect a combination of all three processes (see Table 40-2). Patients with substance dependence may have a susceptibility based on neurodevelopment, including a predisposition to risk-taking behaviors.44 Substance abuse also causes neuroplastic changes at the level of the synapse.45 Intoxication influences neurotransmission, activating dopaminergic reward circuits. Finally, chronic use of substances can cause neurodegeneration and dementia.46
Content
The “content” of a psychiatric illness comprises the neuroanatomy, neurochemistry, and neuropharmacy that may play a role in the given illness. Examples are shown in Table 40-3. This is the subject of the remaining sections of this chapter. For most conditions, our knowledge of content is incomplete, but this should not lead us to ignore the large amount of information that is available for many conditions. Characterizing neuropsychiatric conditions in terms of both biological processes and substrates (content) can provide a framework to facilitate understanding of etiology, loci of intervention, and potential treatments.
Table 40-3 Content in Neuroscientific Explanation in Psychiatry
Content | ||
Neuroanatomy (regional localization, distributed neurocircuitry) | ||
Neurochemistry (neurotransmitters, cells, genes, molecules) | ||
Neuropharmacology (mechanism of action of therapeutic interventions or exogenous drugs of abuse) | ||
Examples |
Disorder | Content Type | Details |
---|---|---|
Heroin dependence | Neuroanatomy | Reward circuitry, ventral tegmental area → nucleus accumbens and cortex |
Neurochemistry | Opioids bind mu-opioid receptors in ventral tegmental area and other regions | |
Ventral tegmental area neurons release dopamine in nucleus accumbens | ||
Neuroplastic changes in neurocircuitry | ||
Neuropharmacology | Naloxone inhibits opioid action acutely | |
Narcolepsy | Neuroanatomy | Loss of hypothalamic orexin projections to areas of brain mediating wakefulness, in particular the brainstem locus coeruleus, which projects diffusely to cortex |
Neurochemistry | ||
Neuropharmacology |
OVERVIEW OF THE STRUCTURE OF THE CENTRAL NERVOUS SYSTEM
The structural organization of the central nervous system (CNS) is shown in Figure 40-3, A. The human brain is organized into the cerebral cortex, brainstem, subcortical structures (e.g., basal ganglia, brainstem, thalamus, hypothalamus, pituitary), and cerebellum.3,47,48
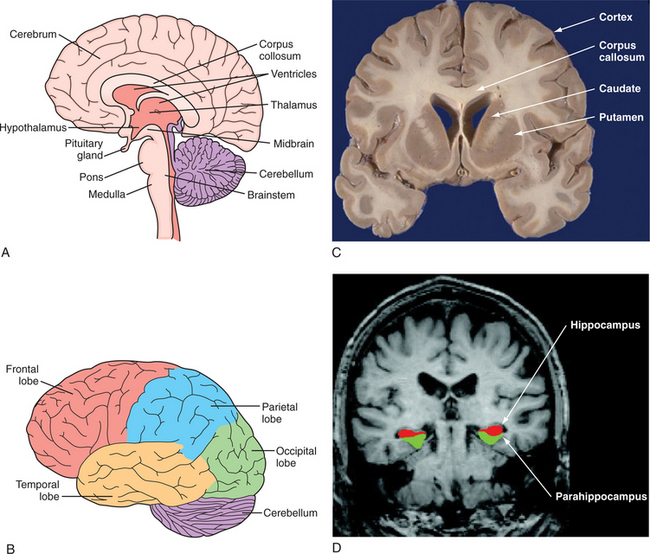
(C, From http://library.med.utah.edu/WebPath/HISTHTML/NEURANAT/CNS213A.html. D, From Dickerson BC, Salat DH, Bates JF, et al: Medial temporal lobe function and structure in mild cognitive impairment, Ann Neurol 56[1]:27-35, 2004.)
The cerebral cortex is the outermost layer of the cerebrum. The cerebral cortex consists of a foliated structure, encompassing gyri and sulci. Within the most highly evolved cortical regions (isocortex), a six-cell layered structure orchestrates complex brain functions (including perceptual awareness, thought, language, planning, memory, attention, and consciousness). Cortical anatomy can be subdivided many different ways, including into anatomical regions (such as the occipital, parietal, temporal, insular, limbic, and frontal lobes) (Figure 40-3, B, C, and D
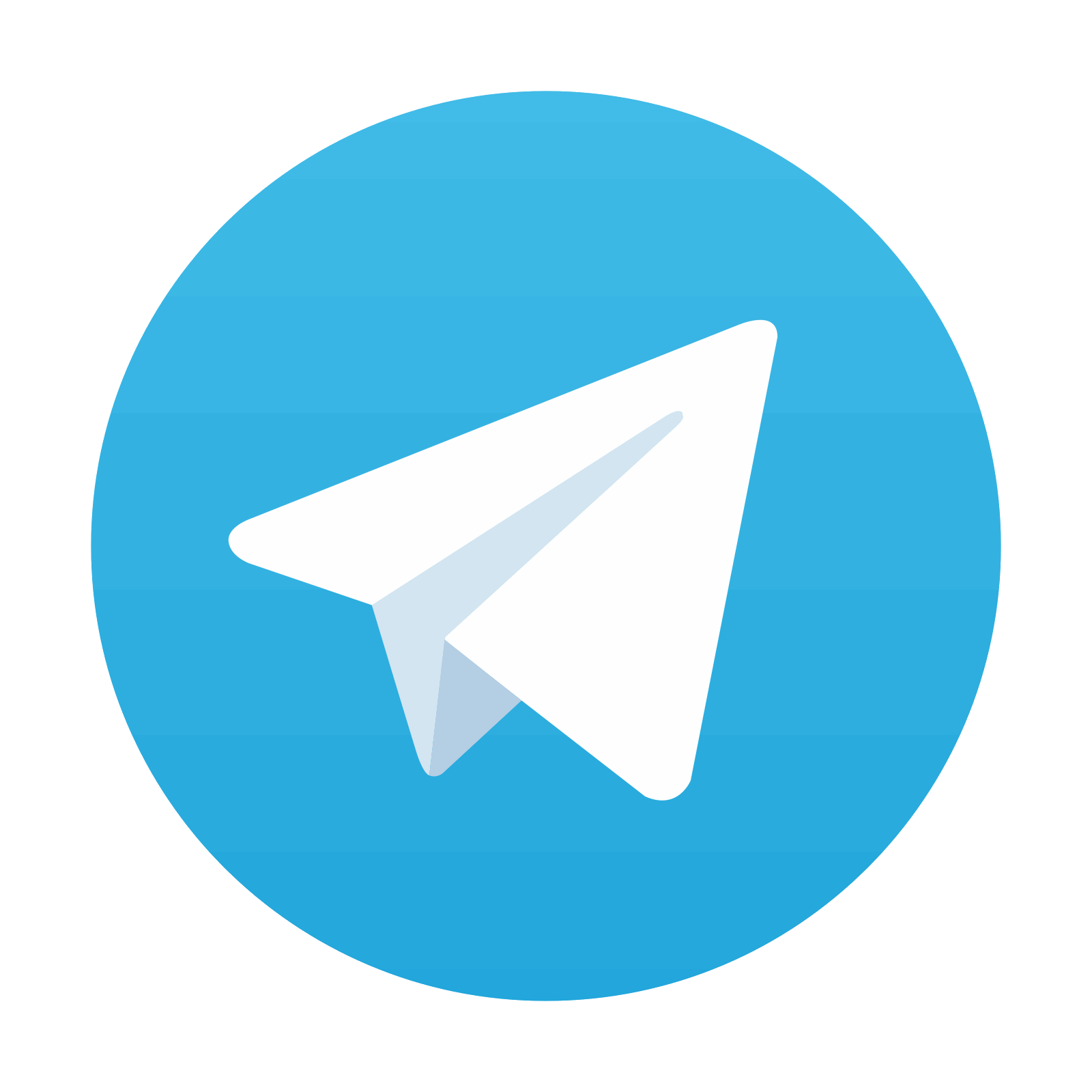
Stay updated, free articles. Join our Telegram channel

Full access? Get Clinical Tree
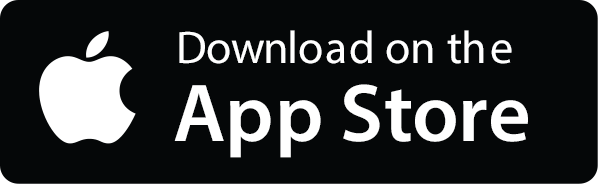
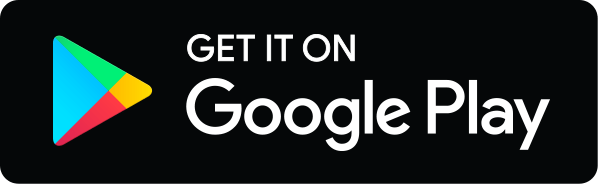