Abstract
As part of the innate immune response, leukotrienes initiate the first line of defense against tissue damage. They also play key pathological roles in chronic inflammatory diseases like asthma. The synthesis of leukotrienes requires two key enzymes: 5-lipoxygenase (5-LO) and 5-lipoxygenase-activating protein (FLAP). Antileukotriene therapies have been used for over 2 decades to manage asthma and include 5-LO inhibitors and the leukotriene receptor antagonists. FLAP inhibitors have also been proven safe and efficacious in asthma clinical trials but have not been brought to the market. Accumulating evidence indicates that leukotrienes mediate the pathophysiology of acute brain injuries and chronic neurodegenerative diseases. These findings have generated a renewed interest in FLAP inhibitors as interventional therapies for these disorders. In this chapter, we provide a short introduction to leukotrienes and discuss the potential use of FLAP inhibitors for treating the acute and chronic sequelae of traumatic brain injury.
Keywords
5-Lipoxygenase, Brain injury, FLAP inhibitor, Leukotrienes, Neuroinflammation
Leukotrienes
The 5-lipoxygenase (5-LO) pathway mediates the production of leukotrienes, a class of potent inflammatory lipid mediators made in leuko cytes that contain a conjugated triene as part of their structure. The biosynthesis of leukotrienes is under strict control (for review see ). Upon infection or tissue injury, cells of the innate immune system (neutrophils, monocytes, basophils, eosinophils, and tissue specific macrophages, ie, brain microglia) recognize pathogen-associated molecular patterns (PAMPs) or damage-associated molecular patterns (DAMPs) and become activated. Upon activation, the cytosolic concentration of calcium increases, leading to the activation and translocation of cytosolic phospholipase A2α (cPLA2α) to the nuclear membrane where it cleaves arachidonic acid (AA) from glycerolphospholipids ( ). The release of AA is the first critical step in leukotriene formation. Like cPLA 2 , the enzyme 5-LO translocates to the nuclear membrane in response to elevated intracellular calcium levels. There it interacts with the 5-LO-activating protein (FLAP) and oxidizes AA to its metabolites. The first enzymatic step is the abstraction of a hydrogen atom from C-7 of AA followed by the addition of molecular oxygen to form 5-hydroperoxyeicosatetraenoic acid (5-HpETE). A second enzymatic step is removal of a hydrogen atom from C-10, resulting in the formation of the conjugated triene epoxide leukotriene A 4 (LTA 4 ) which is enzymatically converted into either leukotriene B 4 (LTB 4 ) by LTA 4 hydrolase or leukotriene C 4 (LTC 4 ) by LTC 4 synthase. Metabolism of LTC 4 occurs by sequential peptide cleavage reactions involving a γ-glutamyl transpeptidase that forms leukotriene D 4 (LTD 4 ) and a membrane-bound dipeptidase that converts LTD 4 into leukotriene E 4 (LTE 4 ) before ω-oxidation ( Fig. 12.1 ).

An important regulatory step in leukotriene biosynthesis involves the transport of the intermediate LTA 4 from an immune cell to a nonimmune cell that expresses LTC 4 synthase. For example, infiltrated neutrophils in the brain contain 5-LO and FLAP and are capable of making LTB 4 and the intermediate LTA 4 . The LTA 4 is released from these cells and taken up by neighboring astrocytes that have LTC 4 synthase but silenced genes for 5-LO and FLAP. Astrocytes convert LTA 4 to LTC 4 and then subsequently in a stepwise fashion to LTD 4 and LTE 4 ( ). This mechanism of leukotriene biosynthesis, that requires the interaction of two distinct cell types, is termed transcellular biosynthesis.
The biological activities of LTB 4 include neutrophil chemotaxis and sequential activation of downstream inflammatory responses. LTC 4 , LTD 4 , and LTE 4 , collectively known as the cysteinyl leukotrienes, are known to mediate vascular permeability, cytokine and chemokine production, and smooth muscle contractility. Leukotrienes exert their biological activities through G protein-coupled receptors (GPCRs); BLT-1 and BLT-2 receptors for LTB 4 and Cys-LT1, Cys-LT2, and Cys-LT3 receptors for the cysteinyl leukotrienes. The rank order of potency for the Cys-LT1 receptor is LTD 4 ≫ LTC 4 > LTE 4 ( ) and for the Cys-LT2 receptor is LTD 4 = LTC 4 > LTE 4 ( ). The recently discovered Cys-LT3 receptor (also referred to as GPR17) has the highest affinity for LTE 4 and also binds purinergic ligands ( ). The Cys-LT1 receptor is mostly expressed in lung smooth muscle cells, interstitial lung macrophages, and spleen, and it is known to mediate airway inflammation and asthma. It is the molecular target of the receptor antagonists (montelukast, zafirlukast, and pranlukast) used for treating asthma ( ). The Cys-LT2 receptor is predominately expressed in the heart, brain, and adrenal glands. Until recently, the only pharmacological inhibitor for Cys-LT2 receptor has been the nonselective dual antagonist/partial agonist Bay-u9773. However, a selective Cys-LT2 receptor antagonist, HAMI3379, and a selective Cys-LT2 receptor agonist, N -methyl LTC 4 (NMLTC 4 ), have been reported ( ). These drugs will help to identify the precise physiological and pathophysiological roles of the Cys-LT2 receptor. The receptor that binds both LTE 4 and purinergic ligands (Cys-LT3 or GPR17) is predominantly expressed in the brain and binds two receptor antagonists (AR-C69931MX and MR52179) selectively ( ) ( Table 12.1 ).
Receptor | Predominant Location | Agonist | Antagonist |
---|---|---|---|
BLT1 | Leukocytes | LTB 4 | LY293111, CP105696 LY255283, U75302 |
BLT2 | Ubiquitous expression | LTB 4 | LY255283 |
Cys-LT1 | Spleen, lung, smooth muscle | LTD 4 ≫ LTC 4 > LTE 4 | Montelukast, pranlukast, BAYu9773 |
Cys-LT2 | Heart, brain, adrenal glands | LTD 4 = LTC 4 > LTE 4 | BAYu9773 |
Cys-LT3 (GPR17) | Brain | LTE 4 > LTC 4 , LTD 4 | AR-C69331MX MR52179 |
Approved Antileukotriene Drugs
The pathophysiological role of leukotrienes in asthma and allergic rhinitis is well documented ( ). Over the past 25 years, considerable effort has focused on identifying and developing drugs that block the actions of leukotrienes to improve asthma management and limit its morbidity. Currently, the leukotriene receptor antagonists (zafirlukast (Accolate), montelukast (Singulair), and pranlukast (Onon)) and the 5-LO inhibitor, zileuton (Zyflo), are approved drugs for treating asthma. The receptor antagonists primarily block the actions of Cys-LT1, only one of the three receptors for leukotrienes and do not block the actions of LTB 4 . The 5-LO inhibitor zileuton has suboptimal efficacy and an associate risk of liver toxicity. These limitations have led to the ongoing exploration of FLAP inhibitors as alternative therapeutics for asthma as well as other inflammatory disorders.
The Discovery of 5-Lipoxygenase-Activating Protein and the Development of 5-Lipoxygenase-Activating Protein Inhibitors
In the search for drugs to treat asthma, in the late 1980s Merck-Frosst scientists identified an indole compound in a chemical screen that blocked the synthesis of leukotrienes both in vitro and in vivo without binding to 5-LO ( ). Using affinity chromatography, an integral membrane protein that specifically bound the indole compound was identified and named FLAP ( ). Although the exact role of FLAP in the leukotriene biosynthesis is still not fully elucidated, a widely accepted hypothesis is that it selectively transfers AA to 5-LO and enhances the sequential oxidation of AA to 5-HpETE and the dehydration to LTA 4 . These are the only known roles of FLAP, and cellular synthesis of leukotrienes can be completely abolished by antagonists that bind to FLAP ( ).
Shortly after the discovery of FLAP, FLAP antagonists including the indole MK-886, the quinoline BAY X1005, and the quinoline–indole MK-591 were developed and tested in phase I/II asthma trials. All three drugs were efficacious and had good clinical safety profiles ( ). However, in contrast to the leukotriene receptor antagonists that were being developed concurrently and brought to the market, none of the early FLAP inhibitors were commercially developed ( Fig. 12.2 ).

Theoretically, FLAP inhibitors have added therapeutic benefits over leukotriene receptor antagonists by virtue of their ability to block the production of LTB 4 as well as LTC 4 , LTD 4 , and LTE 4 . Amira Pharmaceuticals picked up where the early studies left off and modified the indole compound MK-591 to generate compounds like AM-103 and AM-679 that had improved potency and longer half-lives ( ). GSK recently licensed the Amira FLAP inhibitor AM803 (GSK2190915) and completed several Phase II clinical trials with asthmatic subjects. By substituting the indole of MK-591 with indolizines, oxadiazoles, and biarylamides, Boehringer Ingelheim has also claimed multiple proprietary FLAP inhibitors ( ). With several FLAP inhibitors now reaching late-stage clinical trials, it is likely that FLAP inhibitors will be brought to the market in the next few years.
The Potential Use of 5-Lipoxygenase-Activating Protein Inhibitors in Acute Brain Injury
Leukotriene levels in healthy brain are very low or absent. However, after acute injuries like focal ischemia ( ) and traumatic brain injury (TBI; ), leukotrienes are markedly elevated in the brain. Using reverse-phase chromatography coupled to tandem mass spectrometry (RP-LC/MS/MS) to measure and quantify leukotrienes, our laboratory has shown that leukotrienes are produced rapidly after TBI by a transcellular process involving endogenous microglia or infiltrating neutrophils and nonimmune brain cells. In rodents, leukotriene levels peak within 1–3 h of injury and return to basal levels by 24 h after injury ( ). reported a second phase of leukotriene production at 7 days after experimental TBI. It is intriguing to speculate that a second phase of leukotriene production after TBI could mediate a second chronic phase of blood–brain barrier (BBB) permeability and inflammation ( Fig. 12.3 ).

Consistent with the finding that leukotrienes are increased after TBI, leukotriene receptors (Cys-LT1 and Cys-LT2) are also upregulated following brain injury ( ). The Cys-LT1 receptor has been reported to mediate increased permeability of the BBB, vasogenic brain edema, and astrocyte proliferation after brain ischemia, while the Cys-LT2 receptor is thought to regulate cytotoxic brain edema after ischemic injury ( ). In agreement with these findings, the Cys-LT1 receptor antagonist, pranlukast, was shown to decrease neutrophil infiltration, immunoglobulin G (IgG) extravasation, and lesion volumes when administered before ischemic brain injury ( ). The Cys-LT1 receptor antagonist, montelukast, was reported to decrease BBB permeability and neutrophil infiltration after diffuse brain injury when administered preinjury ( ). Additional studies are needed to understand the precise location and role of the leukotriene receptor subtypes in the brain.
More recently, our laboratory demonstrated the protective effects of FLAP inhibitors on TBI-related outcomes when administered before or after injury ( ). The FLAP inhibitor MK-886 significantly blocked the injury-induced production of leukotrienes in the brain. Blocking the production of leukotrienes, in turn, attenuated brain swelling, BBB permeability in the hippocampus, and deficits in both synaptic plasticity (ie, long-term potentiation; LTP) and learning and memory.
It seems likely that the inflammatory actions of leukotrienes (ie, increasing BBB permeability, recruiting activated microglia, and regulating cytokine production) contribute to impaired synaptic functioning. The neurovascular unit that makes up the BBB, comprised of endothelial cells, astrocytic end-feet, pericytes, and neurons, is known to play a key role in regulating synaptic activity. Other investigators reported that blocking leukotrienes before head injury either has no effect ( ) or mitigates ( ) BBB permeability. An important factor that could explain these differences is the contribution of mechanical injury to blood vessels following a TBI. Mechanical injury to blood vessels could mask an effect of leukotrienes or other neurochemicals on regulating BBB permeability. reported a similar conclusion regarding the potential masking of neurochemical regulation of BBB permeability by mechanical damage to blood vessels. In their study, neutrophil depletion, which reduces leukotriene production, resulted in reduced vasogenic edema and tissue loss but had no apparent effect on BBB disruption following controlled cortical impact. In our studies, fluid percussion injury results in extensive damage of the leptomeningeal layer at the surface of the brain. FLAP inhibitors had little effect on the extravasation of gandolinium in this area. However, in brain regions farther away from the impact, we were able to detect an effect of leukotriene blockade on extravasation of dyes into the brain parenchyma.
In addition to their proinflammatory actions, leukotrienes may influence synaptic signaling by other mechanisms. In our fluid percussion injury model, the deficits in hippocampal LTP following TBI were observed in the presence of normal basal synaptic responses and without detectable hippocampal cell loss. Neuronal mechanisms that could result in LTP deficits without the loss of neurons could involve changes in the morphology or density of hippocampal dendritic spines as well as changes in the levels or activity of postsynaptic density proteins known to regulate LTP. and group has reported a calcineurin-dependent loss of dendritic spines in rat forebrain following fluid percussion injury that eventually led to an overgrowth of spines. Other laboratories have shown decreased hippocampal levels of calcium/calmodulin-dependent protein kinase II (CaMKII) and activated CaMKII, a protein kinase that regulates LTP by modulating the phosphorylation state and insertion of AMPA receptors in postsynaptic membranes, after TBI ( ). Another possible mechanism by which leukotrienes regulate cognition may involve β-amyloid peptides. Leukotrienes increase β-amyloid production in mice ( ) and in hippocampal cultures (Heidenreich, unpublished data), and soluble αβ peptides are known to decrease LTP and impair synaptic activity. More research is needed to understand the precise mechanisms by which leukotrienes regulate synaptic plasticity, but the mechanisms are likely to include both proinflammatory and noninflammatory actions of these lipid mediators.
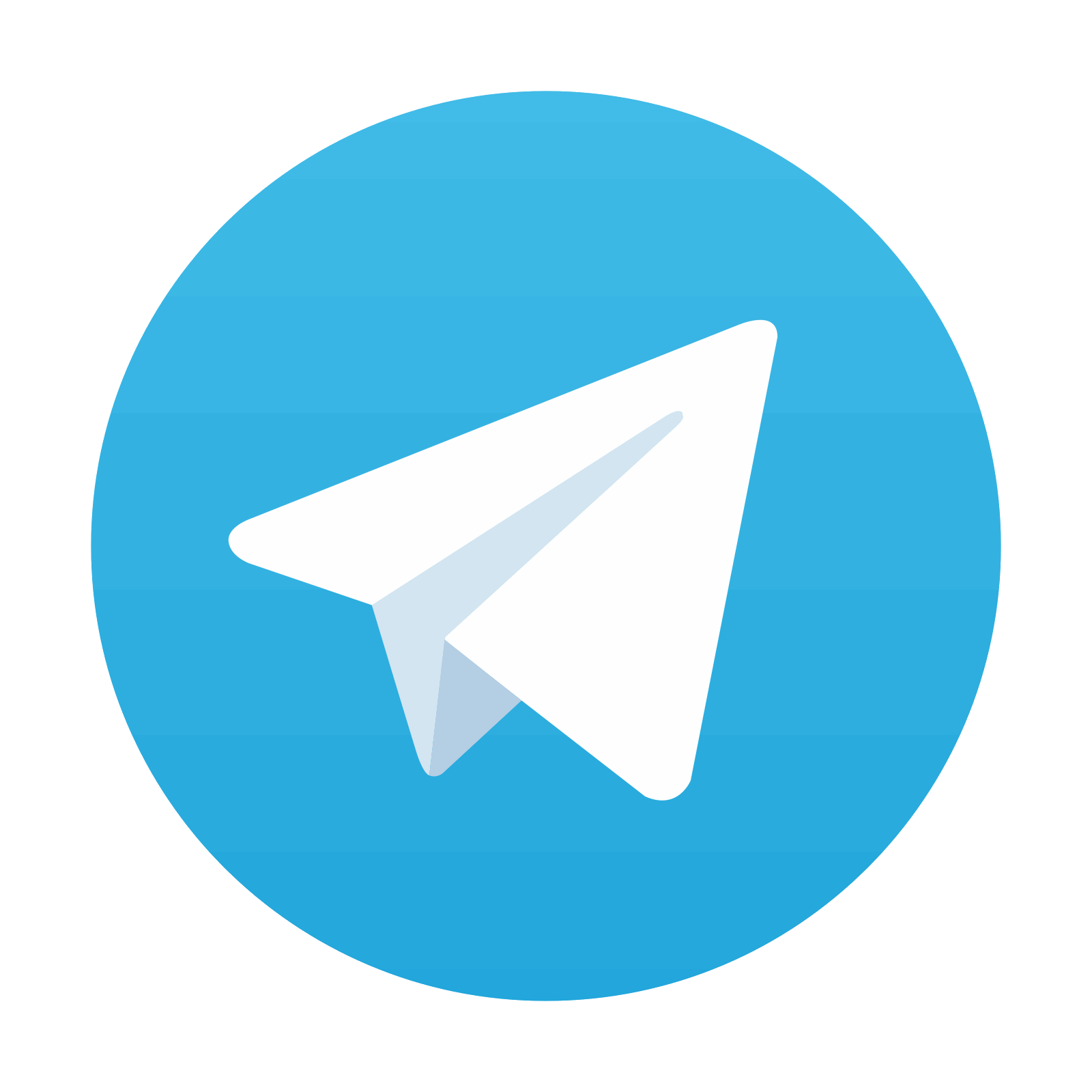
Stay updated, free articles. Join our Telegram channel

Full access? Get Clinical Tree
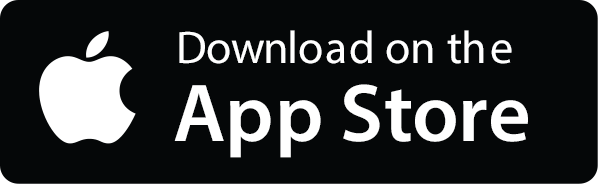
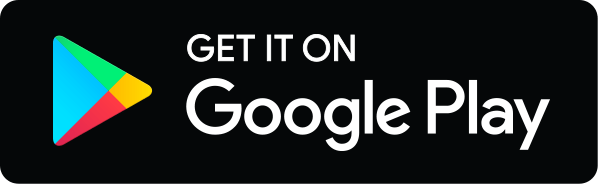