Abstract
Traumatic brain injury (TBI) is a leading cause of death and disability in the United States, and, to date, no pharmacological agents are known to improve neurological outcome following injury. TBI is associated with a severity-dependent accumulation of intracellular calcium ([Ca 2+ ] i ) lasting hours-to-days postinjury and driving apoptotic and necrotic cell death. Pathological accumulation of calcium can also lead to breakdown of structural proteins and changes in gene expression resulting in long-term dysfunction of surviving cells. In in vitro models of TBI, L- and N-type voltage-gated calcium channel (VGCC) blockers reduced [Ca 2+ ] i accumulation and glutamate release resulting in reduced cell death. In rodent models of TBI, administration of VGCC blockers reduced cell death and improved spatial learning and motor function. Based on these data, there is a clear role of VGCC in postinjury pathophysiology, and manipulation of these channels has the potential to improve neurological outcome following injury.
Keywords
Antagonists, Cognition, Neuroprotection, Small peptide antagonists, Traumatic brain injury, Voltage-gated calcium channels
Traumatic Brain Injury
The most recent reports from the Centers for Disease control estimate that over 3.2 million individuals experience a traumatic brain injury (TBI) annually in the United States, with well over 5.3 million patients reporting injury-induced chronic disability ( ). A fiscal analysis indicated that the annual total cost of TBI management exceeds $221 billion dollars annually with 93% (or $205 billion dollars) associated with chronic medical care, interventions to improve quality of life, and lost wages ( ). Despite numerous clinical trials, no effective pharmacological interventions to improve outcome following TBI have been translated from the laboratory to the bedside ( ). In this chapter we review the role of calcium in posttraumatic pathophysiology, a background into voltage-gated calcium channels (VGCCs), preclinical evidence that blockers of VGCCs can reduce calcium accumulation, and subsequent glutamate release following injury and finally a description of initial clinical trials into these VGCC blockers. Our vision is that continued research into VGCC, as well as other target systems, will identify pharmacological agents that could be used to improve neurological outcome following TBI.
Traumatic Brain Injury and [Ca 2+ ] i
Calcium ions (Ca 2+ ) are essential in the regulation of many cellular systems including release of neurotransmitters and hormones, enzyme activity, intracellular transport, contractile processes, glycolysis, respiration, mitosis, membrane potential, and intracellular communication ( ). Disruption of calcium regulation, specifically prolonged periods of elevated cytosolic free calcium, can be catastrophic for the cell leading to chronic dysfunction or death ( Fig. 11.1 ). It is therefore not surprising that TBI-induced disruption of Ca 2+ homeostasis contributes to cell injury and death ( ). Specifically, influx of Ca 2+ triggers a range of downstream effects including activation of apoptotic pathways ( ), mitochondrial dysfunction ( ), free radical production ( ), lipid peroxidation ( ), and osmotic disturbances ( ). Additional neuronal insults such as posttraumatic hypoxia ( ) and ischemia ( ) can augment the calcium-induced pathophysiology intracellular calcium ([Ca 2+ ] i ) resulting in additional cell death and neuronal dysfunction. In fact, calcium has been called the “final common pathway” for toxic cell death in several pathological conditions, including TBI, stroke, and epilepsy ( ).

Injury-induced accumulation of Ca 2+ can trigger changes in secondary cascades within the cell such as phosphorylation of CaMKII ( ), and expression of immediate early genes, which, in turn, can lead to changes in gene transcription and translation. Subsequent changes in gene expression may be responsible for observed changes in the expression of glutamate ( ), GABA ( ), cholinergic receptors ( ), synaptic excitability ( ), as well as changes in neuronal morphology ( ). Therefore TBI-induced accumulation of [Ca 2+ ] i has been linked to both cell death and dysfunction.
Regulation of calcium homeostasis is complex, with several cellular mechanisms operating to control its influx, sequestration, compartmentalization, and efflux. As a result there are several potential targets in the Ca 2+ cascade that could be exploited for pharmacological intervention following TBI. For example, one could target calcium-dependent mechanisms regulating glutamate release, or various signaling cascades involving calcium including activation of calpains, caspases, and the mitochondrial permeability transition pore. However, blocking important calcium-mediated regulatory pathways necessary for normal cellular function can also be toxic to the cell in physiological conditions let alone pathological. An additional concern is that improving overall cell viability after TBI by manipulating downstream calcium signaling may simply result in greater numbers of surviving but dysfunctional cells. Finally, due to the complexity of calcium signaling mechanisms, blocking a single calcium-dependent pathway after TBI, particularly downstream, once multiple pathways have been activated, may not be sufficient to prevent other calcium-activated mechanisms from producing secondary cell injury. Therefore, targeting a mechanism that can both decrease glutamate release, and also limit [Ca 2+ ] i accumulation, without completely inhibiting neuronal transmission, has the potential to improve neuronal viability while also preserving the function of the cells. In this review we argue that manipulation of VGCCs may be a rational strategy to achieve this goal.
Voltage-Gated Calcium Channels
VGCCs ( Fig. 11.2 ) are heteromultimers formed by four subunits including a single α 1 subunit, which incorporates the conduction pore, the voltage sensor, and the gating apparatus, and a combination of three auxiliary subunits α 2 -δ, β, and γ ( ) each of which modifies the properties of the VGCC ( ). The α 1 subunit contains four homologous domains each with six transmembrane helical segments (S1–S6) ( ). The S4 segment functions as the voltage sensor and ion selectivity and conductance is determined by the S5 and S6. There are 10 characterized α 1 subunits that are associated with six classes of VGCCs ( ). There is also evidence that splice variants of the L- ( ) and N-type ( ) VGCC influence the affinity and sensitivity of channels to specific antagonists.

Voltage-Gated Calcium Channel Subtypes
VGCCs were initially classified based on their biophysical and pharmacological properties ( Fig. 11.2 ) and included the L-, N-, P-, Q-, R-, and T-type channels ( ). Based on the complexity of these channels, a gene-based nomenclature was established. L-type channels are now designated as Ca v 1.1–1.4, P/Q as Ca v 2.1, N as Ca v 2.2, R as Ca v 2.3, and T-type channels as Ca v 3.1–3.3 ( ). To maintain clarity with the published literature related to L- and N-type VGCC and TBI, we will use the original nomenclature for the remainder of this chapter.
VGCC subtypes differ in their conductance rates, voltage activation threshold, and rate of inactivation. L-type channels are high-threshold, large-conductance, and slow inactivating ( ). The N-, P-, Q-, and R-type voltage have intermediate conductances and inactivation kinetics relative to the L-type, yet have medium to high activation thresholds ( ). The T-type channel is a low-threshold, low-conductance, rapidly inactivating calcium channel ( ).
Voltage-Gated Calcium Channel Distribution
When considering potential targets for intervention, it is critical to consider on which types of cells VGCC are expressed and what their specific role is related to cellular function. L-type VGCCs, the initial channel type evaluated for treatment of TBI, are widely distributed across the brain but are less abundant in neuronal tissues compared to other VGCCs ( ). N-type VGCCs, on the other hand, are expressed in high densities in the cerebral cortex, dendritic zones of the hippocampus, amygdala, septal nuclei, medial geniculate, superior colliculus, molecular layers of cerebellar cortex, n. solitary tract, and spinal cord ( ). Similarly, P/Q-type calcium channels are broadly expressed including on both hippocampal pyramidal and granule cells as well as on cerebellar granule cells, interneurons, and Purkinje cells ( ). While expressed in the central nervous system, relatively little is known about the possible role of R- and T-type VGCCs in TBI.
Based on their channel properties and anatomical location, VGCCs play a variety of roles in neurotransmission and cell function ( Fig. 11.3 ). At the cellular level, N- and P/Q-type VGCCs are found both pre- and postsynaptically and interact directly with presynaptic core proteins syntaxin and SNAP-25, providing a molecular basis for Ca 2+ influx into nerve terminals, neurotransmitter release ( ) and fast synaptic transmission ( ). L-type channels, however, are located primarily postsynaptically and do not play a direct role in neurotransmitter release ( ). While all six types of VGCCs are found in brain ( ) only the N-, P-, Q-, and R-type channels are involved in regulating calcium-dependent neurotransmitter release from presynaptic terminals ( ). The T-type channels generate rhythmic burst firing of neurons and are thought to contribute to network synchrony and, in pathological cases, epilepsy ( ). Astrocytes have L-, N-, and possibly T-type channels, while L- and N-type VGCCs have been observed on oligodendrocytes ( ).

Antagonism of Voltage-Gated Calcium Channel
While there are a range of compounds that are demonstrated blockers of VGCC, for the purpose of this chapter on TBI, we will focus on the two classes that have been the most extensively evaluated ( Table 11.1 ): dihydropyridine antagonists and short amino acid peptides. Dihydropyridine antagonists (eg, nimodipine, nifedipine, verapamil, amlodipine) block L-channels robustly ( ); however, these compounds also can have weak effects across the spectrum of VGCC. The ω-conotoxins or ω-conopeptides are basic, water-soluble, 24–29 amino acid peptides isolated from the venom of fish-hunting marine snails belonging to the genus Conus ( ), and ω-agatoxins are small peptides isolated from spider venom ( ). Many of these peptides are able to specifically block the N-, P-, and/or Q-type channels. For example, ω-conotoxins GVIA, TVIA (SNX185), and MVIIA (ie, SNX111) block the N-type calcium channel ( ) while the ω-agatoxin AgaIVA is a potent blocker of P- and Q-type VGCCs. Some peptides, such as ω-conotoxin MVIIC, have less specific effects, antagonizing the N-, P-, and Q-type channels ( ). Blockade of either L-type or N-type channel has been evaluated as a strategy for improving outcome following TBI, but similar studies have not yet been carried out with blockers of P/Q-, T-, or R-type VGCCs ( ).
Channel Type | L | N | P | Q | R | T |
---|---|---|---|---|---|---|
Dihydropyridines (IC50) | ||||||
Nimodipine | 0.135–2.6 μM | None | None | None | None | 5–11 μM |
Nifedipine | 100 nM | None | None | None | None | 39 μM |
Nicardipine | 9–26 μM | None | 32–97 μM | 32–97 μM | None | 5–13 μM |
Verapamil | 0.6–1 μM | None | None | None | None | 20–30 μM |
Small Peptides (IC50) | ||||||
ω-Conotoxin MVIIA | None | 78 nM–1 μM | None | None | None | None |
ω-Conotoxin GVIA | None | 28 nM–2 μM | None | None | None | None |
ω-Agatoxin AgaIVA | None | None | 15 nM | 50 nM–1 μM | 50 nm | None |
ω-Conotoxin MVIIC | None | 18 nM | 18 nM | 50 nM–1 μM | None | None |
ω-Agatoxin AgaIIIA | 1 nm | 1 nm | IC50 N/A | IC50 N/A | None | None |
SNX-482 | None | None | 30–750 nm | 30–750 nm | 15–30 nM | None |
Neuroprotective Effects of Voltage-Gated Calcium Channel Blockers
The pathophysiology of TBI has typically been separated into primary and secondary injuries. Primary injury is a result of an external force that is applied to the brain. This initial force can cause mechanical tissue destruction producing contusions, lacerations, shearing of axonal connections and hemorrhage and is known to initiate secondary injury cascades. These cascades include a large influx of calcium that can drive additional cell dysfunction, cell death, and ultimately substantial increase in morbidity ( ).
To evaluate the subcellular effects of TBI, several in vitro models of mechanical strain injury have been developed which allow investigators to compare real time changes in [Ca 2+ ] i with cell survival. In these models, cells (ie, neurons, astrocytes, or mixed cultures) are typically grown on a flexible membrane that, when deformed, produces a mechanical strain injury that mimics the shearing forces associated with many types of TBI ( ). These in vitro studies demonstrated that there is an injury severity-dependent increase in [Ca 2+ ] i in both neurons and astrocytes that occurs immediately following injury that, depending on magnitude of injury, appears to resolve in minutes-to-hours following injury ( ). Calcium entry after injury can occur through opening of VGCCs, activation of the N -methyl- d -aspartate (NMDA) receptor ( ) and release of calcium from intracellular stores ( ). Mechanical strain injury of cultured neurons not only increases acute [Ca 2+ ] i but also triggers delayed depolarization that can last for up to 24 h ( ). Ultimately, the activation of these cascades results in significant neuronal and astrocytic cell death ( ) as well as lasting neuronal dysfunction ( ).
The effects of TBI on calcium have also been evaluated using several in vivo models. In weight drop, or impact acceleration model of TBI, calcium-sensitive electrodes detected a rapid and significant decrease in extracellular calcium at the focus of injury ( ), argued to be caused by a significant movement of calcium into cell near the focus of injury ( ). Sustained calcium accumulation in the cortex, hippocampus, and thalamus lasting up to 2 weeks following a fluid percussion TBI has been demonstrated using 45 Ca 2+ autoradiography ( ). In brain slice preparations, [Ca 2+ ] i levels remained elevated for at least 1 week following fluid percussion TBI with calcium homeostasis remaining impaired for at least 30 days following the injury ( ).
One of the major concerns following TBI is the uncontrollable increase in intracranial pressure that can be caused, at least in part, by cytotoxic edema ( ). There is well-documented evidence that TBI induces microglial activation leading to the synthesis and release of proinflammatory cytokines, chemokines, nitric oxide, prostaglandins, growth factors, and superoxide species ( ). Interestingly, there is evidence that L-type VGCCs are expressed on microglia ( ) and that blockade of L-type channels can reduce inflammation. While there is no data related specifically to TBI, in an in vitro model of lipopolysaccharide-induced inflammation, decreasing calcium currents through the L-type channel on microglia by application of nimodipine inhibited the production and release of proinflammatory molecules such as nitric oxide, tumor necrosis factor-alpha, interleukin-1beta (IL-1β), and prostaglandin E2. Ultimately, blockade of these L-type channels led to increased viability in surrounding neurons ( ). Similarly, nimodipine was able to dose-dependently reduce the synthesis and release of IL-1β from microglia and improve neuronal viability following injection of Aβ directly into the hippocampus ( ). Therefore, when considering potential intervention in TBI using VGCC blockers, it is important to consider both neuronal and nonneuronal targets and the specific channels expressed on each of the cell types.
Considered together the in vivo as well as in vitro data describe a postinjury environment that is characterized by a rapid increase in [Ca 2+ ] i , and altered calcium dynamics that can last for weeks following an injury. As previously described, VGCCs are a portal for calcium movement, and when neurons are depolarized after TBI the opening of VGCCs can contribute to the accumulation of toxic levels of calcium, both through the channel as well as indirectly via enhanced release of neurotransmitters such as glutamate. Therefore antagonism of VGCCs is a rational approach to reduce the posttraumatic calcium-induced pathophysiology and has the potential to be an effective therapeutic strategy to reduce cell death and dysfunction.
Evidence for the Role of L-Type Voltage-Gated Calcium Channel in Traumatic Brain Injury
The first clinical trial of VGCC blockers to improve outcome following TBI focused on compounds that block the L-type channel. As previously described, the L-type VGCC require strong depolarization for activation, and activation is related to long-lasting calcium currents ( ), features consistent with post-TBI pathology. There are three primary classes of L-type VGCC antagonists: including phenalkylamines (verapamil, D-600), benzothiazepine (diltiazem), and dihydropyridines (nimodipine, nifedipine, nitrendipine) ( ). Preclinical studies of the dihydropyridine nimodipine to treat ischemia in both rodent ( ) and primate models ( ) revealed that antagonism of the L-type channel improved cerebral blood flow, reduced injury-induced infarct volume, and critically improved behavioral outcome. These improvements were observed in the absence of significant drug-related side effects. Preclinical trials of nimodipine were successfully translated to the treatment of the acute effects of ischemia in patients ( ), including an improvement in blood flow with improved behavioral outcomes.
While L-type channels were well studied in models of ischemia far fewer preclinical studies examined the ability of dihydropyridines to improve outcome in models of TBI. In an initial in vitro study, the application of a cocktail of a sodium channel blocker (tetrodotoxin, TTX), NMDA antagonist ((2 R )-amino-5-phosphonopentanoate; APV), AMPA antagonist (6-cyano-7-nitroquinoxaline-2,3-dione; CNQX), and nimodipine applied immediately before a mechanical strain significantly reduced the average accumulation of [Ca 2+ ] i as well as lowered the total number of neurons experiencing significant increases in [Ca 2+ ] i ( ). Administration of a different dihydropyridine L-type antagonist, nifedipine, also significantly reduced cell death and improved cell function in an organotypic hippocampal slice culture ( ).
Initial in vivo studies of L-type antagonists in TBI tested the efficacy of the phenalkylamine verapamil, to improve outcome following lateral fluid percussion injury in rats. Similar to what was observed in ischemic models, antagonism of the L-type VGCC significantly improved cerebral blood flow and behavioral outcome. Based on the observed effect on blood flow, the authors suggested that a TBI-induced large-scale activation of L-type VGCC would lead to a loss of vasoreactivity which was why antagonism of these channels resulted in improved blood flow ( ). A subsequent study of nimodipine indicated that not only could antagonism of L-type channels improve blood flow but it could also reduce injury-induced lipid peroxidation ( ).
Based on such preclinical evidence, L-type VGCC antagonists have been extensively evaluated in clinical trials for severe TBI. In the first two clinical trials, HIT1 and HIT2, 7 days of nimodipine was administered to a cohort of severe TBI patients. Initial findings indicated only a modest trend toward improved outcome in the absence of any significant side effects ( ). However, there were sufficient patients between the two trials that the authors were able to subdivide the injured population based on injury type. Following reanalysis of the data based on injury type, the authors made an additional observation that nimodipine was more efficacious in patients with evidence of traumatic subarachnoid hemorrhage (determined from their initial CT scan). Perhaps these data are not surprising given the known benefit of L-type VGCC antagonism on spontaneous subarachnoid hemorrhage. However, these data have been contradicted by subsequent studies that failed to find a similar beneficial effect even within this subpopulation ( ). A more recent study reported that cerebral metabolism and outcome were improved in a small TBI patient population by intravenous nimodipine treatment ( ). In summary, antagonists of L-type VGCC have not provided compelling evidence that L-type channels are an effective therapeutic target for acute treatment of TBI.
Evidence for the Role of N-Type Voltage-Gated Calcium Channel in Traumatic Brain Injury
Similar to the L-type channels, N-type VGCCs are activated by strong depolarization. However, unlike L-type channels, the N-type are found on the axon terminals of neurons ( ) as well as on the soma and in dendrites. As previously described, presynaptic N-type channels play a critical role in regulating neurotransmitter release. Therefore blockade of the N-type channel can reduce postsynaptic accumulation of [Ca 2+ ] i both by reducing entry through the channels themselves, as well as reducing the release of neurotransmitters. For example, if glutamate release were reduced, N-type VGCC blockers would also decrease activation of multiple postsynaptic targets reducing depolarization (AMPA and kainite) as well as entry of calcium through the NMDA receptor.
As previously described, a number of small peptides were isolated from venomous cone snails that are very specific and potent inhibitors of N-type VGCCs ( ). One subclass, the ω-conotoxins, is highly selective for the N-type VGCC, and a synthetic form of the toxin called ziconotide (also known as MVIIA, SNX-111, Prialt) was subsequently developed and tested preclinically for pain management and for neuroprotection. Preclinical studies with ziconotide found that blockade of N-type channels can reduce chronic pain as well as reduce cell death in models of ischemia and TBI ( ). While both L- and N-type channels have demonstrated efficacy in preclinical models, the hypothetical mechanism of protection is different: evidence suggests that L-type channels can improve outcome by improving blood flow, while N-type channel blockade appears to be neuroprotective by blocking calcium-mediated secondary injury mechanisms. An in vitro study examined the effects of the VGCC blocker SNX-185, a synthetic form of ω-conotoxin TVIA, for its ability to limit [Ca 2+ ] i accumulation in neurons using Fura-2 radiometric imaging and to improve cell viability after mechanical strain injury ( ). SNX-185 is similar to MVIIA (ie, ziconotide) in its selectivity for N-type VGCCs. Pretreatment of mixed neuronal/astrocyte cultures with SNX-185 (100 or 1000 nM) significantly reduced the rise in [Ca 2+ ] i and improved survival of injured neurons following mechanical strain injury ( ). However, treatment delayed by 5 min after injury did not improve cell survival nor did it prevent the immediate rise in [Ca 2+ ] i . However, further investigation of the effects of posttreatment of cultures with SNX-185 revealed that elevated levels of [Ca 2+ ] i returned to near normal levels more rapidly in injured cells treated with SNX-185 than in injured but untreated cells, suggesting that treatment with the N-type channel blocker facilitated a return to a more normal state of calcium homeostasis ( ). This was argued to be due, at least in part, to the ability of N-type blockade to reduce the release of glutamate from presynaptic neurons ( ). Recently, these same authors tested whether SNX-185 would have similar effects in an in vitro model of mechanical injury with hypoxia as a second insult ( ). Compared to the normoxic condition, hypoxia further increased the rise of [Ca 2+ ] after injury at three postinjury imaging time points examined, and resulted in greater cell death across all mechanical strain injury severities. Postinjury treatment with SNX-185 reduced the effects of secondary hypoxia on [Ca 2+ ] i and improved neuronal viability. In summary these in vitro data demonstrate that antagonism of the N-type channel can reduce glutamate release, blunt the accumulation of [Ca 2+ ] i, , and facilitate a more rapid return to calcium homeostasis.
In vivo studies have also provided support for the therapeutic potential of N-type VGCC blockade to be neuroprotective in models of TBI. For example, when administered intravenously 1 h following lateral fluid percussion TBI, ziconotide significantly reduced the increase in 45 Ca 2 accumulation in the dorsal hippocampus, thalamus, and cortex for up to 48 h following injury ( ). Intravenous administration of ziconotide between 4 and 10 h from the time of a weight drop impact acceleration injury significantly improved mitochondrial respiration in the injured brain, likely related to the decreased calcium load experienced by mitochondria ( ). Repeated intravenous injections of ziconotide at 3, 6, and 12 h after an injury, significantly improved cognitive and sensory-motor function in rats after diffuse axonal injury ( ). Direct stereotaxic injection of SNX-185 into the dorsal hippocampus acutely following LFP injury in rats significantly reduced loss of pyramidal neurons in the ipsilateral hippocampus and improved motor and cognitive function ( ). Therefore, similar to L-type VGCC, N-type antagonists improved outcome in both in vitro and in vivo models of TBI. However, unlike L-type channels, whose effects appear to be secondary to improved blood flow, N-type channel blockers appear to be neuroprotective by limiting accumulation of [Ca 2+ ] i resulting in increased cell viability after injury along with improved cognitive and motor outcomes.
One of the key findings in the preclinical research was that ziconotide was efficacious in rats even when delivered as much as 4 h following injury ( ). Furthermore, several key sequela that were reduced with ziconotide treatment in rat are observed in TBI patients. Specifically, similar to the rat elevations in extracellular glutamate ( ), mitochondrial dysfunction ( ) and calcium-induced neurotoxicity ( ) have been observed in clinical studies. Therefore, the enthusiasm for translating an N-type VGCC blocker from bench-to-bedside was high. It is noteworthy, therefore, that intravenous ziconotide was used early in its initial preclinical development in a feasibility trial in severe TBI patients. Unfortunately, the study was halted before completion due to complicating cardiovascular effects (ie, hypotension) that had not been observed in preclinical rodent models. In fact, the authors of the clinical trial suggested that, perhaps, the effects were not seen in rodents as the model injury was less severe than TBI that were being treated clinically ( ). It was hypothesized that these effects were likely due to the effects of N-type antagonists on heart rate and blood pressure ( ), and the results of this trial have never been published.
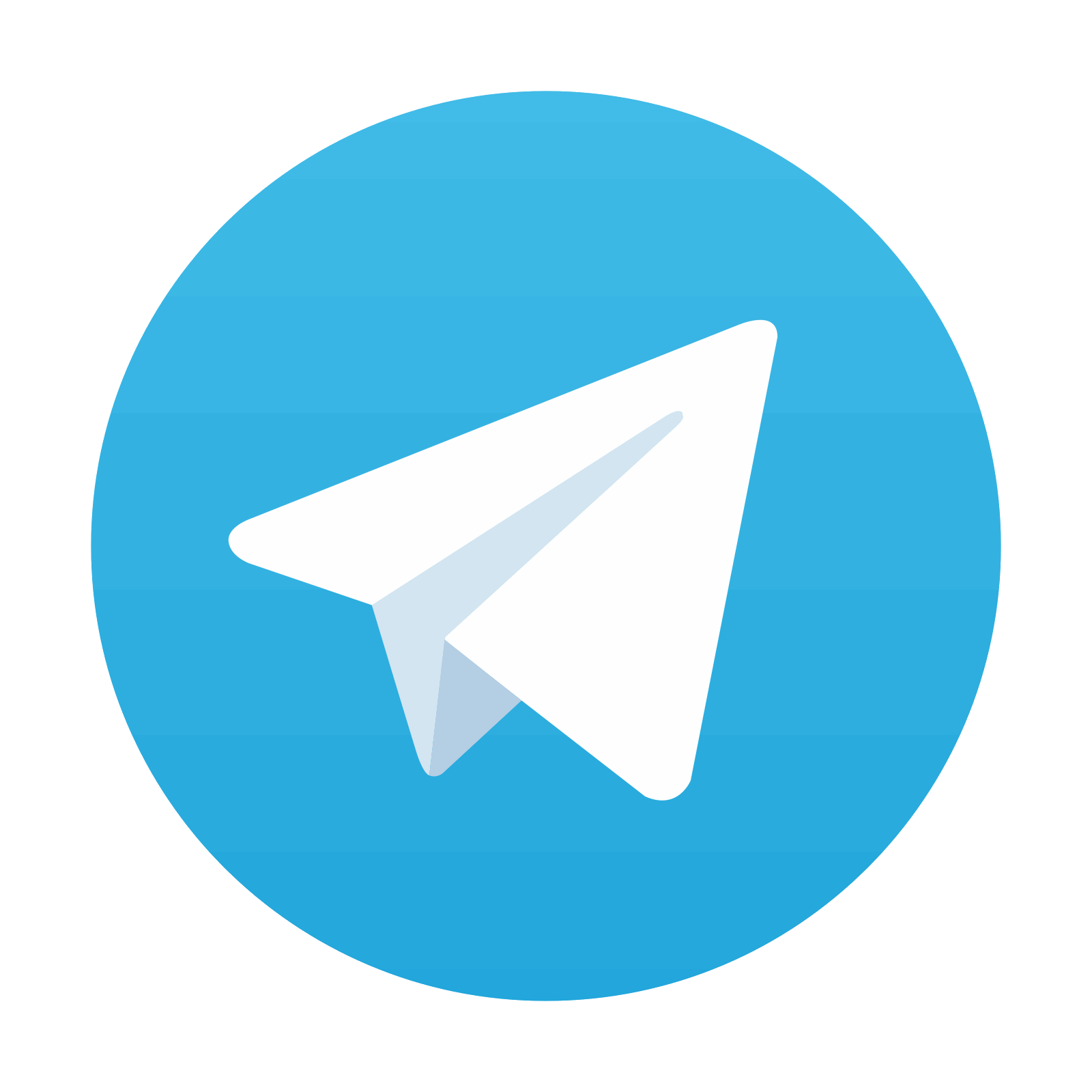
Stay updated, free articles. Join our Telegram channel

Full access? Get Clinical Tree
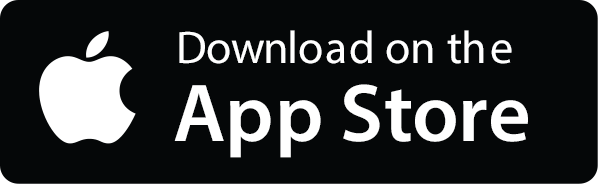
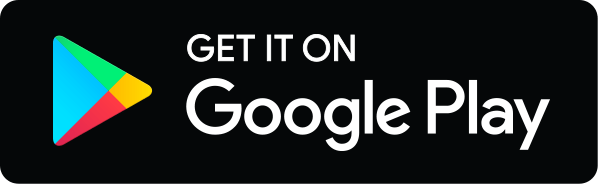