Adjacent Level Disc Biomechanics
Brian J. C. Freeman
Donal S. McNally
Adjacent segment degeneration either above or below a previously fused lumbar motion segment has a reported incidence of between 25% to 45% (3,12,16,17). Not all such patients are symptomatic. Lehmann et al. (12) reported a 45% incidence of segmental instability above a previous fusion after a median follow-up of 33 years. Penta et al. (16) performed magnetic resonance imaging on 52 patients 10 years following anterior lumbar interbody fusion. All patients had had preoperative lumbar discography demonstrating a normal disc in the adjacent level. The incidence of a normal adjacent intervertebral disc 10 years following a solid fusion to the sacrum was 68%. This was not influenced by the length of the fusion. The findings suggest that degeneration after an anterior lumbar interbody fusion is determined more by individual characteristics than by the fusion itself. Aota et al. (3) suggested that age is the most significant predictor for developing adjacent segment degeneration above an instrumented fusion. Patients older than 55 years had an incidence of 37%, whereas the incidence for patients younger than 55 years was only 12%. Older patients appeared to be more susceptible with less ability to correct sagittal and coronal balance, leading to acceleration of the process. Rahm and Hall (17) studied 49 patients who had undergone an instrumented lumbar fusion. Thirty-five percent of patients showed degenerative changes above the fused segment after radiographic evaluation. For patients undergoing anterior cervical discectomy and instrumented fusion, approximately 25% will suffer from further degenerative disease at levels adjacent to the fusion within 10 years of the initial surgery (10).
Increased mechanical demands are known to affect the disc adversely and may interfere with the normal nutritional supply of the disc. However, there is dispute over whether the elimination of motion through fusion leads to higher forces on the remaining discs or whether the degenerative changes are merely a part of the inevitable natural history process (11). In two clinical studies, the levels adjacent to the adjacent segment were nearly as likely to have long-term degenerative change as the level immediately adjacent to the fusion (9,19). Battié et al. (5) showed that genetic inheritance accounts for approximately 70% of intervertebral disc degeneration with environmental factors such as heavy work and cigarette smoking having only a modest effect.
Biomechanical testing of cadaveric specimens remains the gold standard for testing spinal implants. It is important to ensure that the spinal device is tested under conditions that simulate the loading conditions that are likely to occur in life. Compressive forces and bending moments can be applied using one or two rollers (13). Recommendations for the standardization of in vitro stability testing of spinal implants have been made by Wilke et al. (24). In particular, the magnitude of the applied loads and moments are
important. Wilke et al. suggested a bending moment of 7.5 Nm for the lumbar spine to simulate everyday loading (24). Corresponding axial compressive loads for the lumbar spine might be of the order 1 to 2 kN for moderate activities.
important. Wilke et al. suggested a bending moment of 7.5 Nm for the lumbar spine to simulate everyday loading (24). Corresponding axial compressive loads for the lumbar spine might be of the order 1 to 2 kN for moderate activities.
The key to understanding the effects of a fixation device on disc biomechanics is the measurement of the internal distribution of load within the disc. This information can be used to give further information about the relative load sharing of the disc and the fixation device. If measured correctly, it can give much information about the behavior of the disc tissues themselves. Load going through the disc may be measured as an intervertebral disc pressure in the center of the nucleus pulposus (15) or across the whole diameter of the disc using stress profilometry (14). In this technique, a needle-mounted solid-state transducer is withdrawn at a steady state across the disc while a static compressive load of 2 kN is applied to the motion segment for a period of 20 seconds. A standard apparatus for measuring stress distributions inside cadaveric intervertebral discs is shown in Fig. 1.1. Typical “stress profiles” for lumbar intervertebral discs of varying degrees of degeneration have been described (2).
One limitation of in vitro biomechanical studies is the fact that they take no account of functional muscle mass, bone density, or load transfers to other adjacent segments of the spine, particularly in the case of a spinal fusion model. In addition simply removing the disc and inserting an interbody cage device into the cadaveric specimen prior to testing will not make any allowance for the biologic changes in stiffness that would normally occur over time as the motion segment fuses. Despite these limitations, much can be gained from this type of research (13).
Adjacent Level Disc Biomechanics above a Simulated Fusion Model
Weinhoffer et al. (23) carried out an in vitro study to determine the intradiscal pressure changes during flexion in levels above a simulated fusion. The authors used six fresh frozen cadaver spines and investigated two levels (L3-4 and L4-5) in each specimen. Pressure measurements were taken with the spine uninstrumented, with bilateral pedicle screw-rod instrumentation between L5 and S1, and with bilateral pedicle screw-rod instrumentation between L4 and S1. Pressure measurements were made using a Millar-mikro tip pressure transducer placed respectively within the nucleus pulposus of L3-4 and L4-5. Pressure data were recorded by computer data acquisition. The pressure data were compared by intervertebral level and by the effects of added instrumentation. The intradiscal pressure increased as the number of levels involved in the simulated fusion increased. The intradiscal pressure increased as flexion motion increased. A greater increase was seen at the L4-5 level compared with the L3-4 level. When the L5-S1 fixation was added, the intradiscal pressure increased. When the L4 to S1 fixation was added, the intradiscal pressure increased still further.
Cunningham et al. (6) measured intradiscal pressure at three levels in 11 cadaveric lumbar spines under four conditions of spinal stability (intact, destabilized, laminar hook instrumentation, pedicle screw reconstruction). In response to destabilization and instrumentation, proximal disc pressures increased by as much as 45%, whereas in the bridged disc the pressure decreased by 41% to 55%, depending on the instrumentation used. The authors conclude that the changes in segmental intradiscal pressure levels occur in response to spinal destabilization and instrumentation. Intradiscal cyclic pressure differentials drive the metabolic production and exchange of disc substances. Conditions of high or low disc pressure secondary to spinal instrumentation may serve as the impetus for altered metabolic exchange and predispose operative and adjacent levels to disc pathology.
Rohlmann et al. (18) studied the effect of an internal fixator and bone graft simulation on intersegmental spinal motion and intradiscal pressure in the adjacent regions. The authors used seven fresh frozen lumbar cadaver spines (mean age 28 years, range 16–69). Intradiscal pressures were measured in the nucleus pulposus of four levels of each specimen. Intersegmental motion was measured using a three-dimensional motion analysis system. Four situations were studied:
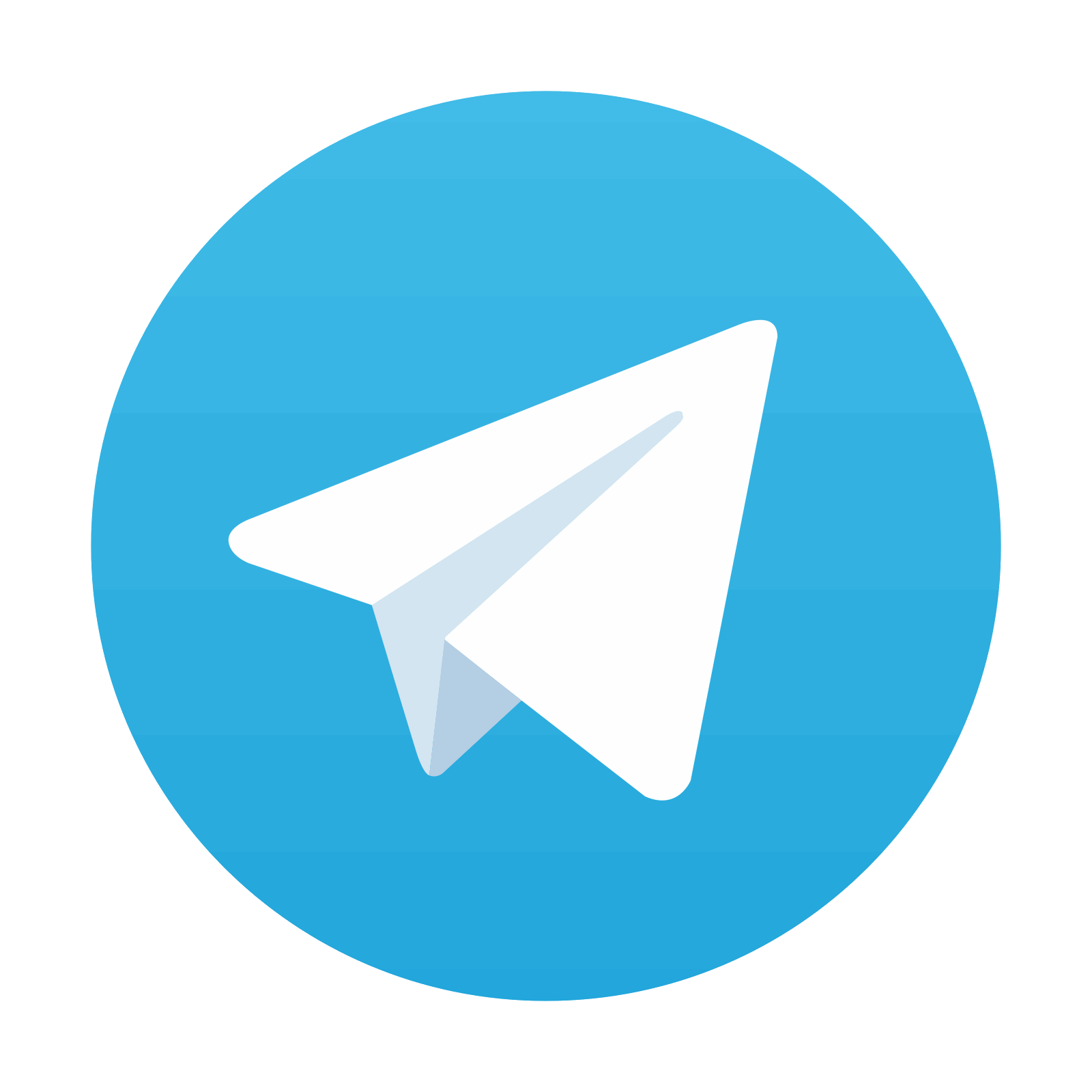
Stay updated, free articles. Join our Telegram channel

Full access? Get Clinical Tree
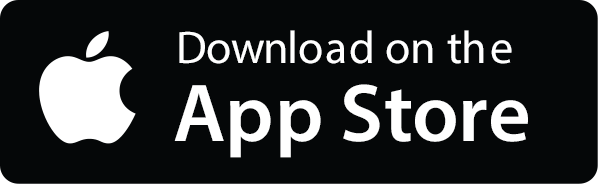
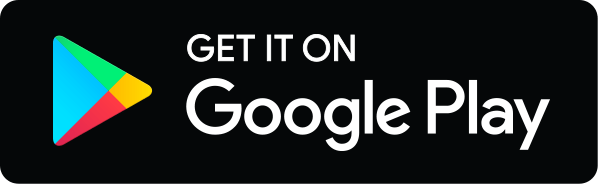