Fig. 1
Transmission and epidemiology of human African trypanosomiasis (HAT). Top: the two subspecies of Trypanosoma brucei (T. b.), T. b. gambiense and T. b. rhodesiense, causing the two forms of HAT, respectively, are transmitted by the bite of different species of tsetse flies (genus Glossina, G.) in endemic regions of sub-Saharan Africa. Humans are the primary reservoir for T. b. gambiense, which may also infect animals. Cattle and wild animals are the primary reservoirs for T. b. rhodesiense. Bottom left: the chart indicates the number of reported new HAT cases in the last nine decades. Note the epidemic peaks of the disease in the 1930s (when control and surveillance by mobile teams were instituted during the colonial period), and in the late 1990s. The World Health Organization (WHO) and partner organizations, including national control programs, launched then initiatives for HAT control and surveillance, resulting in a steady decrease of new reported cases. Bottom right: geographical distribution of T. b. gambiense infection (yellow shading), which causes the vast majority of HAT cases, and of T. b. rhodesiense infection (light blue shading). The superimposed markers indicate new HAT cases reported in 2009 for each of the countries under WHO observation, according to the “HAT atlas initiative” (Simarro et al. 2010). Blank circles represent countries with no reported new cases; question marks indicate countries with incomplete data. Colored circles represent foci with less than 100 new reported cases; circle size is roughly proportional to the number of reported cases. The number of new reported HAT cases is indicated. Modified from Kristensson et al. (2010), courtesy of Giuseppe Bertini
Two subspecies of T. b. are responsible for HAT forms with different geographical distribution (Fig. 1) and clinical course. Infection with T. b. gambiense causes the chronic form of HAT in Western and Central Africa; infected humans are the primary reservoir, though animals (including pigs and sheep) may also be infected. T. b. gambiense is transmitted by three species of tsetse flies, and mainly by Glossina palpalis. Gambian HAT has an average course of 3 years; parasitaemia is in general low and occurs in cycles, rendering difficult the detection of parasites in blood smears. Infection with T. b. rhodesiense, transmitted mainly by the Glossina morsitans group of tsetse flies, causes the acute, zoonotic form of HAT in Eastern and Southern Africa. A number of wildlife animal species (including those in game parks) and domestic animal species (especially cattle) act as reservoirs for T. b. rhodesiense. This infection progresses rapidly, with an average course of 6–8 weeks and high parasitaemia. The vast majority of cases of HAT (about 97 %) are due to T. b. gambiense infection, while T. b. rhodesiense infection is responsible for about 3 % of the cases.
Both forms of HAT evolve in two stages: an early, hemolymphatic stage and a subsequent, meningoencephalitic stage, due to T. b. invasion of the CNS. The parasite passage across the blood-brain barrier to invade the CNS parenchyma is an active multistep process, dealt with in the companion chapter of this volume (see Masocha et al. 2014).
Due to rapid switching of the expression of genes encoding variant surface glycoproteins that compose the parasite coat, T. b. can evade the immune system of the host. For this high degree of antigenic variation, no vaccine to prevent HAT is available at present. As mentioned further (see Sect. 4), the therapy currently available to cure CNS disease in both forms of HAT has severe adverse effects. Although disease staging is, therefore, essential for therapeutic decisions, precise stage biomarkers have not been identified as yet.
As stated by Gadelha et al. (2011), “the African trypanosome is among the deadliest of human pathogens”. If left untreated, both forms of HAT are almost invariably fatal. However, a limited number of cases of human trypanotolerance, possibly associated with genetic variability of the parasite and/or the host, has been reported (Jamonneau et al. 2012; Kennedy 2013).
2 Notes on the History of Human African Trypanosomiasis
The appearance of African trypanosomes, dating back to prehistory, far preceded that of mankind. Trypanosome resistance probably played an important role in the selection of early hominids (Steverding 2008).
Throughout history, African trypanosome infection has represented a severe obstacle to the socio-economical development of Africa. This is also due to animal African trypanosomiasis, nagana (from the Zulu word “N’gana” meaning “powerless, useless”), which rendered stock farming very difficult (Steverding 2008). A first case report of sleeping illness, fatal to the Emperor of Mali, is described by the Arabian historian Ibn Khaldun (1332–1406). The first accurate medical report of sleeping sickness was published by the English naval surgeon John Atkins (1685–1757) (Atkins 1734).
Clear understanding of African sleeping sickness etiology and transmission had to wait for the discovery of trypanosomes as a causal agent of cattle nagana by David Bruce (1855–1931) (Bruce 1895, 1897), and that of trypanosomes from the blood of a British patient, who lived in Gambia, affected by the so-called Trypanosoma fever (Dutton 1902). One year later, Aldo Castellani (1878–1971) found trypanosomes in the cerebrospinal fluid (CSF) of patients with symptoms of sleeping sickness and suggested that these parasites could be the causative agent of the disease (Castellani 1903), which initiated a debate on the priority of the discovery (Bentivoglio et al. 1994). The track for causal agents ended with the discovery of Trypanosoma rhodesiense (Fantham and Thomson 1910).
At the end of the nineteenth century, two entities were considered separately: Trypanosoma fever and sleeping sickness. In 1903, the report of the British Sleeping Sickness Commission in Uganda (Anonymous 1903) reached the following conclusions (1) trypanosomes found in Ugandan and Gambian patients are the same parasite; (2) Trypanosoma fever represents the first stage of sleeping sickness; (3) monkeys contract a sleeping sickness-like disease when they are infected with either blood from Trypanosoma fever patients or CSF from sleeping sickness patients; (4) trypanosomes are transmitted by Glossina palpalis tsetse flies; and (5) sleeping sickness is a human disease due to transmission of trypanosomes from patients to healthy individuals by tsetse fly bite. Therefore, Trypanosoma fever and sleeping sickness were then considered as two consecutive stages of the same disease caused by African trypanosomes (Greig and Gray 1904).
Severe epidemics of sleeping sickness ravaged sub-Saharan Africa, affecting mainly Uganda and the Congo basin at the turn of the twentieth century. Led by Charles Louis Alphonse Laveran (1845–1922), the French Commission created a central laboratory at Brazzaville (Congo) and launched mobile teams along the Congo River and affluent rivers. In 4 years, the work accomplished was impressive and was reported in an extensive publication (Martin et al. 1909). The clinical aspects of the disease were presented in detail and its course in two “periods” confirmed. The first period is the “time lapse since the parasites appear in the blood or lymph glands and their discovery in the CSF” (Martin and Leboeuf 1908); “the second period of the disease starts with the appearance of the flagellates in the sub-arachnoid spaces”. The French Commission stressed the importance of clinical signs in stage determination, and distinguished also a third terminal period with cachexia, permanent torpor, convulsions, and final coma. The conclusions of the French Commission were corroborated by Belgian, Portuguese and German reports. At the same time, Broden and Rodhain (1908) used the absence or presence of white blood cells (WBC) in the CSF to distinguish between the two stages of sleeping sickness.
After World War I, extensive control operations established by the colonial authorities “fearing an unpopulated continent and a shortage of human labour to exploit natural resources” (Simarro et al. 2011a), promoted the search and treatment of HAT patients by mobile teams. By the 1960s, sleeping sickness was almost eliminated (Fig. 1). The control of the disease thus lost priority, also due to political instability of many African countries after independence. In the 1980s HAT re-emerged, and the number of reported cases kept increasing. Towards the end of the twentieth century HAT almost reached the levels of epidemics seen at the beginning of the century (Fig. 1), and the World Health Organization (WHO) estimated 300,000 new infected cases every year. At the turn of the twenty-first century, the WHO, with partner organizations including national control programs in endemic countries, reinforced HAT control and surveillance, and the number of reported cases started to decline (Fig. 1). Between 50,000 and 70,000 infected patients were reported by WHO in 2006.
3 Prevalence and Geographical Distribution
Transmission of HAT occurs in discrete areas of endemicity or foci (Fig. 1). Approximately 70 million people (10 % of the total population in endemic countries) are estimated to be at various levels of risk of HAT infection over an area of 1.55 million km2 (7.4 % of the total area of endemic countries) in sub-Saharan Africa (Simarro et al. 2012). The vast “tsetse belt” extends between the Sahara and Kalahari deserts, 14° North and 20° South to the Equator (Fig. 1). Twenty-one million people are currently at moderate to high risk of infection, the largest proportion (82.2 %) being at risk of T. b. gambiense infection (Simarro et al. 2012). While the geographical distribution of the two forms of HAT is roughly delimited by the Rift Valley, there are areas at risk of merges (e.g. lake Tanganyika) between the two forms (Simarro et al. 2010).
Tsetse flies are attracted to dark colors (blue and black) and moving vehicles. Their ecology requires shadow, coolness and warm blood diet. Tsetse flies occur predominantly along lakes and riversides, forest galleries (where they can move beyond 2 km), plantations and village surroundings. However, houses can also attract tsetse flies in their host-seeking behavior (early morning and late afternoon), and during hot hours (late morning and early afternoon) to find a cool refuge (Vale et al. 2013). The natural habitat for the T. b. rhodesiense vector is the savannah. Passive dispersion through movements of domestic animals and humans can cause reinvasion of cleared zones. In particular, movements of cattle along trade routes and through livestock markets represent a big challenge for the control of Rhodesian HAT (Simarro et al. 2010). Population displacements may also spread the disease by new cycles of transmission in previously uninfected vectors (Tong et al. 2011).
Transmission of HAT occurs primarily in rural areas, during activities such as farming, fishing and hunting. Reports of HAT in peri-urban Kinshasa (Democratic Republic of Congo, DRC) indicate that transmission can also occur in peri-urban belts (Fèvre et al. 2008), where tsetse flies have adapted to high population density.
The “HAT atlas initiative”, based on geospatial analysis allowed by modern technologies, was launched in 2008 by WHO with joint implementation by partner organization (Simarro et al. 2010). Geographical-epidemiological maps of the HAT atlas can be found in the WHO website (http://www.who.int). Interestingly, many of the foci of HAT cross national borders, indicating that partnerships in the governmental initiatives of the involved countries are needed for surveillance and control of the disease.
The number of currently reported HAT cases due to T. b. gambiense infection shows a steady decrease in most endemic countries, but still an increase in others, and is highest in DRC (Fig. 2), indicating that sustained surveillance and targeted actions are needed. In 2009, the number of reported new HAT cases has dropped “below the symbolic number of 10,000” (Simarro et al. 2011a). These figures should, however, be regarded with extreme caution due to under-detection and under-reporting. The rate of under-reporting (not formally quantified for Gambian HAT) is as high as 40 % in some foci of Rhodesian HAT (Fèvre et al. 2008), also due to the acuteness and rapid progression of T. b. rhodesiense infection (Simarro et al. 2012).
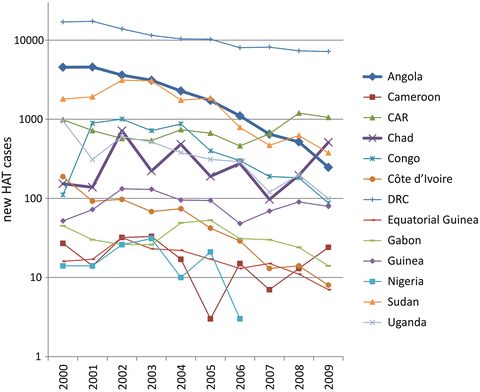
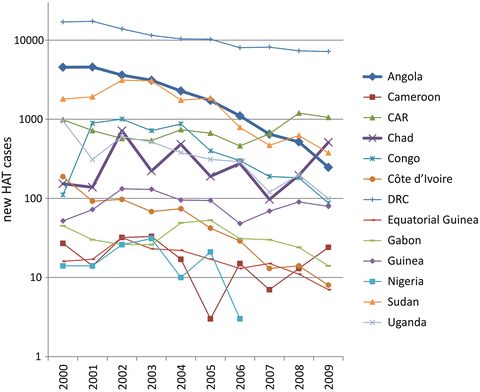
Fig. 2
Graphic representation of the number of reported new HAT cases caused by T. b. gambiense infection in 13 endemic countries in 10 years (2000–2009), based on the “Atlas of human African trypanosomiasis” (Simarro et al. 2010). The thicker lines indicate two paradigmatic situations: a country (Angola) in which new HAT cases dropped, and the worrying situation of another country (Chad), which reported a recent increase of new cases, with foci at the border with the Central African Republic (CAR) in which an increase of new cases is also reported. Courtesy of Giuseppe Bertini
Foci of HAT are currently mostly confined to resource-poor settings: remote rural areas, areas of political instability or armed conflict, where it may be difficult for patients to access treatment (Tong et al. 2011). Qualitative and quantitative analyses of 30 year data have confirmed that clusters of HAT incidence have predominantly coincided with periods of conflict and civil wars (Berrang-Ford et al. 2011). Importantly, these analyses have also indicated a lag period of approximately 10 years between the beginning of conflict events and a peak of HAT incidence (Berrang-Ford et al. 2011). This further implies that the effort for surveillance and control of HAT should be maintained for decades for a chance of success in the elimination of this disease from the African continent.
Active screening (through mobile teams travelling to remote villages to test the population) and passive case-finding (testing at fixed health facilities), followed by treatment and vector control are essential for interventions against HAT (Simarro et al. 2010; Tong et al. 2011). Passive screening is less efficient than active screening, as access to health facilities is very difficult for most HAT patients (Tong et al. 2011). Under-reporting and under-detection of HAT cases can occur both in areas covered by active or passive surveillance, and in areas not covered by surveillance due to remoteness or instability (Simarro et al. 2012).
Disease control can be very difficult in health resource-poor regions, as shown by a recent survey in ten health institutions in Zambia (a country with low scale HAT endemicity). In this survey, based on questionnaires on general knowledge on HAT targeted to health workers, most respondents scored very low, and none of them knew how to differentiate HAT stages (Mwanakasale et al. 2013). Also the follow-up (24 months with control visits every 6 months, recommended by WHO) can be difficult to achieve in resource-limited settings.
Cases of HAT have also been reported in non-endemic areas in Africa, as well as in travelers returning to Europe or North America (Brun et al. 2010; Blum et al. 2011; Migchelsen et al. 2011; Simarro et al. 2011b; Malvy and Chappuis 2011; Kennedy 2013; see also Bisoffi et al. 2014). Gambian HAT has been reported in immigrants, refugees, expatriates resident for long periods in rural areas, and is very rare in short-term travelers. Rhodesian HAT has instead been reported in short-term tourists travelling to game reserves in East Africa. The diagnosis of these cases poses serious challenges (see Sect. 4.2). Awareness of the fact that T. b. infection still represents a risk for travelers and migrants is mandatory. Because of its long incubation time, Gambian HAT should be considered for diagnosis even after long intervals from the stay in an endemic country.
4 Clinical Features
Pathogenetic mechanisms and experimental data on animal models are beyond the scope of the present chapter, as they are discussed in the companion chapter of this volume on the neurobiology of African trypanosomiasis (see Masocha et al. 2014).
Detailed accounts on the current status of the diagnostics and therapy of HAT is also beyond the limits of the present treatise, and are presented in recent publications (Brun et al. 2010; Blum et al. 2011; Bouteille and Buguet 2012; Kennedy 2013; Lejon et al. 2013). Concerning diagnostics, we only wish to recall here that the card agglutination test for trypanosomiasis (CATT), developed in 1978 (Magnus et al. 1978), is still the primary screening tool used in areas endemic for Gambian HAT. This test has 95 % specificity but may result in false positivity, and cannot be used for follow-up or relapses. No similar test is available for Rhodesian HAT, during which, however, there are higher chances to detect the parasites in blood smears due to the high parasitaemia.
Treatment of stage 1 HAT utilizes pentamidine (developed in 1937) and suramin (developed in 1916), which are relatively well tolerated though they may have adverse effects. These drugs, however, do not cross the blood-brain barrier and cannot cure CNS disease. The arsenical compound melarsoprol (developed in 1949) is still used to cure stage 2 of both forms of HAT. Melarsoprol injections are very painful (the patients even refuse the therapy) and adverse effects of the treatment are very severe. In 10 % of the patients, melarsoprol treatment causes post-treatment reactive arsenical encephalopathy, which is fatal in half of the cases. Eflornithine, and more recently the nifurtimox-eflornithine combination therapy have been introduced for CNS disease caused by T. b. gambiense, but require cycles of intravenous administration and have side effects. The search for new drugs for treatment of CNS disease in HAT is ongoing in different laboratories (see Kennedy 2013), but has not been successful up to now.
The issue of HAT staging, which is obviously crucial for therapeutic decisions, is currently highly debated, and also research for effective biomarkers is actively ongoing. Waiting for new, substantial discoveries, the criteria still in use for HAT staging are those indicated by WHO (1998, 2007) guidelines related to CSF parameters (which therefore require a lumbar puncture): the presence of trypanosomes in the CSF and/or ≤5 WBC per μl for stage 1, and >5 WBC per μl for stage 2. Due to different cut-off criteria in different HAT foci, the definition of an intermediate stage (>5 up to ≤20 WBC per μl in the CSF) is adopted in many endemic countries before treatment for HAT stage 2 is initiated (see, for example, Chappuis et al. 2005; Blum et al. 2006; Wastling and Welburn 2011).
An objective clinical evaluation, very much needed for the assessment of HAT severity, is made difficult by the fact that signs and symptoms of HAT are heterogeneous and variable, and would therefore require standardization. Variability of the clinical features of HAT, including the predominance of different neurological signs and symptoms, is also related to different foci (Blum et al. 2006; Kennedy 2013). Clinical features, often described as early and late symptoms, actually evolve as a continuum, with an insidious progression.
A skin lesion (trypanosomal chancre), with erythema and edema, appears from a few days to 2 weeks at the site of the tsetse bite, especially in T. b. rhodesiense infection, while it is rarely seen in T. b. gambiense infection.
Early lesions can include facial edema, fugacious and mobile, mainly localized at the external corner of the lower eyelids which gives the patient a puffy face. It is more common in T. b. gambiense infection than in T. b. rhodesiense infection, during which, however, edema of the extremities can occur.
Skin rash (trypanides) may be observed as polymorphic papulo-erythematous eruptions, often edematous and itchy, distributed in large plaques or in one to several cm-wide striated formations over the trunk or at the thigh, or even all over the body.
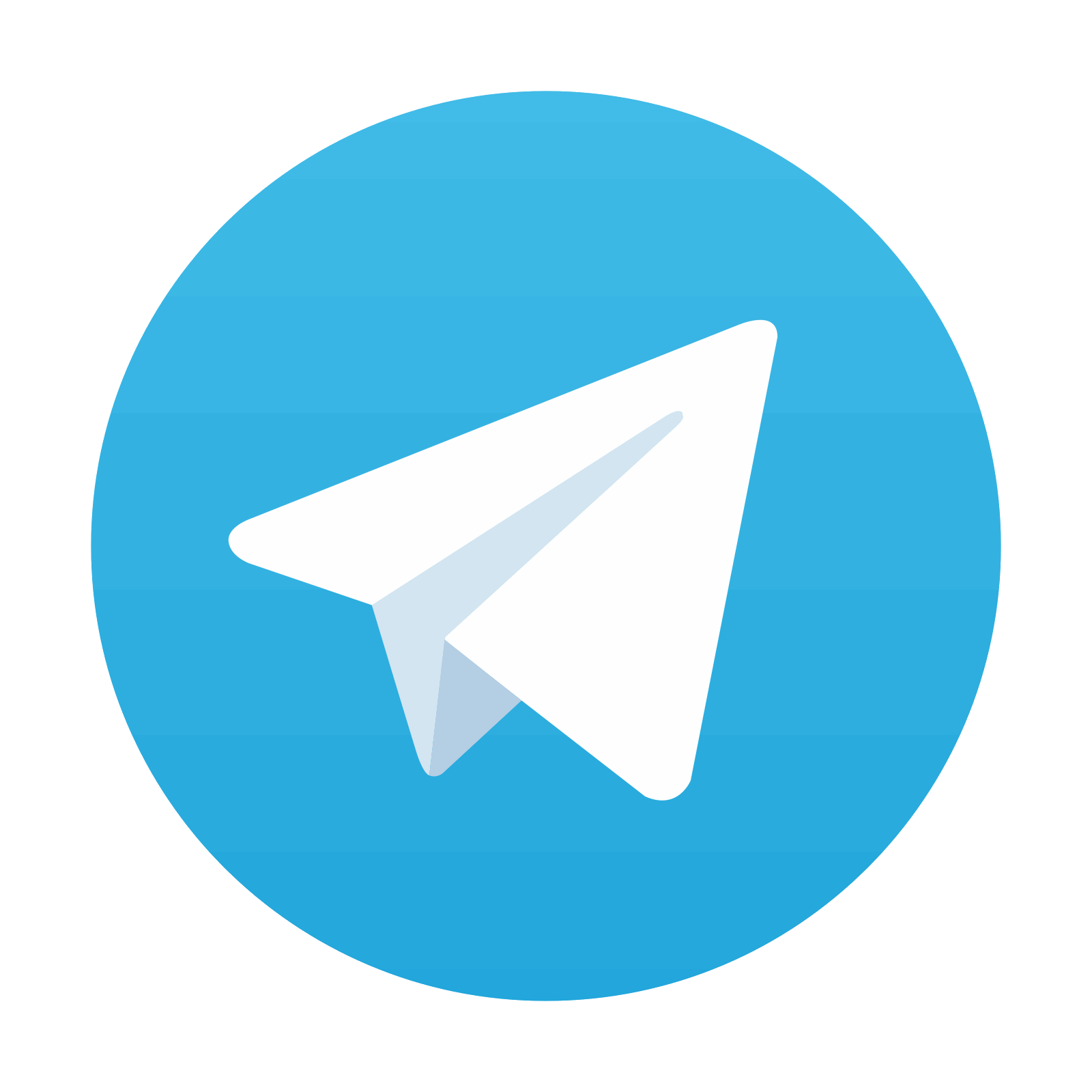
Stay updated, free articles. Join our Telegram channel

Full access? Get Clinical Tree
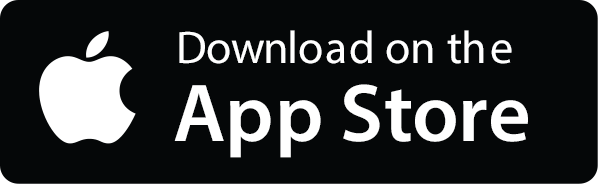
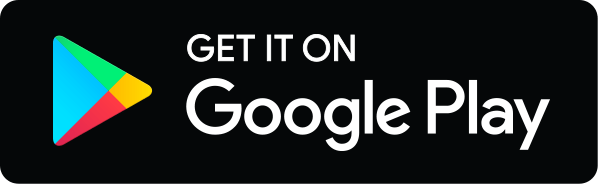