Chapter 39 Animal Models for Disorders of Circadian Functions
Whole Organism
Abstract
One of the most prominent features of life is that plants and animals change their behavior on a regular and consistent basis every 24 hours to match changes in the physical environment that are imposed directly or indirectly by the rotation of the earth. In addition to the easily observed changes in behaviors such as leaf movement, feeding, and sleep and wake, essentially all cellular and physiologic processes within a given organism are also expressing changes over a 24-hour period that repeat themselves like clockwork from day to day. Indeed, as detailed in Chapters 31 to Chapter 34, there is an internal clock located in the suprachiasmatic nuclei (SCN) of the hypothalamus of mammals that synchronizes the animal to the 24-hour solar day, primarily through environmental inputs from the changes in the light–dark (LD) cycle. In turn, the SCN appears to directly or indirectly synchronize hundreds of internal 24-hour rhythmic changes in behavior and in physiologic and cellular functions to both the external environment (external synchronization) and the internal environment (internal synchronization).
One exception to the almost universal feature of regular 24-hour changes in the natural life of an organism is the human. Among the myriad of environmental and lifestyle changes brought about by modern technology is the 24-hour society: our new ability, due to artificial light, to engage in activities at night that in the evolutionary past were limited to the daytime. Of particular interest is shift work, brought about by both technology and economic forces, which thrusts a significant proportion of workers (by some estimates, 15% or more in the United States)1 into highly nontraditional patterns of activity and rest. The sleep–wake cycle of the shift worker represents a significant break from the world in which we evolved, where light and dark alternated reliably. The internal clock system, present in perhaps all living organisms, developed as an adaptation to this predictable day–night geophysical pattern. The biological consequences and causes of living at odds with the normal day–night cycle are just beginning to be appreciated. Shift work, loosely defined to include static night shifts, flex shifts, extended shifts, rotating shifts, and frequent international travel by airline flight crews, has been linked with increased risk of colorectal,2 breast,3 lymphatic,4 and prostate5,6 cancers, as well as gastric ulcers,7,8 obesity,9 diabetes,10,11 stroke,11 coronary heart disease, atherosclerosis, and heart attack.11–14 The mechanisms for these correlations between shift-work exposure and disease are unknown.
Although shift work and jet lag have commonly been the focus of study about the adverse effects of disassociating biological time from real time, technology (television, computers, internet) now allows us to routinely be awake or asleep at any hour, thus spreading the health concerns associated with shift work well beyond that limited population. This drastic change in lifestyle for millions of humans, when coupled with a recent remarkable discovery about the circadian system in animals, makes it particularly important that we develop more (and better) animal models for disorders of circadian function. This recent discovery is that the molecular circadian clock machinery is present not only in the cells of the central circadian clock in the SCN but also in the cells of many other areas of the brain, as well as in most peripheral tissues (e.g., lung, heart, liver, pancreas, muscle).15,16 Furthermore, these cell- and tissue-specific molecular circadian clocks appear to be regulating the 24-hour rhythmic expression of at least 10% of the genes in any given tissue, indicating that circadian dysregulation at the whole-animal level, as well as in specific tissues and organs, could alter the normal synchrony of many components of living systems. Disrupting circadian rhythms could have profound implications for health and disease. This chapter focuses on what is known about the adverse effects associated with disrupting the circadian clock system of the entire organism based on studies using genetic and environmental animal models, and Chapter 40 reviews the evidence for circadian disruption at the cellular, tissue, and organ levels.
Destroying the Master Circadian Clock in the Scn: Effects on Health and Well-Being
Fortunately, one opportunistic cat, meddling with a field study on SCN-lesioned diurnal antelope ground squirrels, lent support for the intuitive expectation of external synchrony.17 These ground squirrels had lesions aimed at the SCN (and verified to be arrhythmic by monitoring daily running-wheel behavior prior to release) and were housed in a large outdoor enclosure along with unlesioned control animals. Behavioral monitoring revealed significantly more nighttime activity among the lesioned squirrels than in the controls. A feral cat entered the enclosure and killed 60% of the lesioned squirrels but only 29% of the controls. This unplanned event revealed a protective role for the biological clock in the “semi wild,” a finding that would be difficult to repeat under normal laboratory-animal housing conditions.
In a larger follow-up study, DeCoursey and colleagues released lesioned and control eastern chipmunks with radio collars into the wild and followed the population of free-living animals for 18 months.18 Although these normally day-active chipmunks, lesioned or not, never ventured out at night, those with SCN lesions were more active during the day in their dens. Perhaps because of this restlessness, weasels were more successful in preying on the lesioned animals and killed a significantly higher proportion of them than those in the control group. So, although having no clock may carry few penalties in a nonchallenging environment, such as a cage containing no predators or infectious disease agents, and in which food and water are provided ad libitum, two key field studies exposed at least one protective role for the clock, in that it organizes behavior into a safer temporal niche.
Importance of “Resonance” Of the Timing of the Internal Circadian Clock With That of the Entraining Ld Cycle
The free-running (i.e., not entrained) period of most plants and animals maintained under constant environmental conditions (e.g., constant darkness and temperature) is almost always close to 24 hours (hence the term circadian, or about a day), and it is rarely less than 23 hours or greater than 25 hours. This has led to the general hypothesis that organisms survive best under LD conditions when the natural free-running circadian period (tau) of the organism is close to that of the external LD cycle (T). Early studies in plants indicated this to be the case,19–21 and pioneering studies by Pittendrigh22 and Aschoff23 and their colleagues in flies support the hypothesis of the importance of T and tau being in close proximity to one another. Fruit flies, housed from the first day of adult life in symmetrical photoperiods (i.e., equal portions of light and dark) of 21 to 28 hours, were found to survive best with days that were 24 hours long, and they fared much worse when the cycles were 21 or 27 hours long.22 Studies in blowflies showed similar results: at a given time, 100% of flies exposed to LD cycles of 24 to 27 hours were still alive, whereas only 90% of flies exposed to a 23- or 28-hour day were still alive, and for flies exposed to a 22- or 20-hour day, the survival rates were only 85% and 70%, respectively.23
A slightly different question was addressed with genetic manipulation: Is there something specific about 24 hours? Carl H. Johnson’s group24 used an elegant approach to answer this question, and in the process managed to directly demonstrate the adaptive advantage not only of having a clock but also of having the right clock for the environment. Populations of cyanobacteria with altered or normal clocks were co-cultured to directly compete for survival in a variety of light cycles that were resonant with the circadian clocks in both strains, just one strain in the dish, or dissonant with both strains. The study showed that in rhythmic environments, the bacteria with normal clocks outcompeted strains with disrupted clocks. This advantage disappeared in nonrhythmic environments. When strains with different internal periods competed against one another, the photoperiod determined the winner. Resonance between the internal clock and the rhythmic environment led to dominance in the dish. The authors concluded that accurate clocks confer a selective advantage and therefore must serve important physiologic function in cyanobacteria.
The only mammalian example showing a benefit of resonance between internal and external timing was only recently published. Earlier, it was shown that the tau mutant hamster, bearing a spontaneous genetic mutation that shortened the period of the circadian oscillation, had a reduced life span relative to wild-type controls25 (but see also reference 26, where opposite results were reported). Martino and colleagues have now shown that severe renal and heart disease is present in aged heterozygous tau mutant hamsters exposed to a T of 24 hours,27 but that housing the animals in a 22-hour light cycle, matched to their endogenous period, prevented the renal and cardiac phenotypes. Ablation of the SCN at a young age also prevented the development of the pathologic cardiorenal phenotype, indicating that no clock is preferable to an incorrect clock.
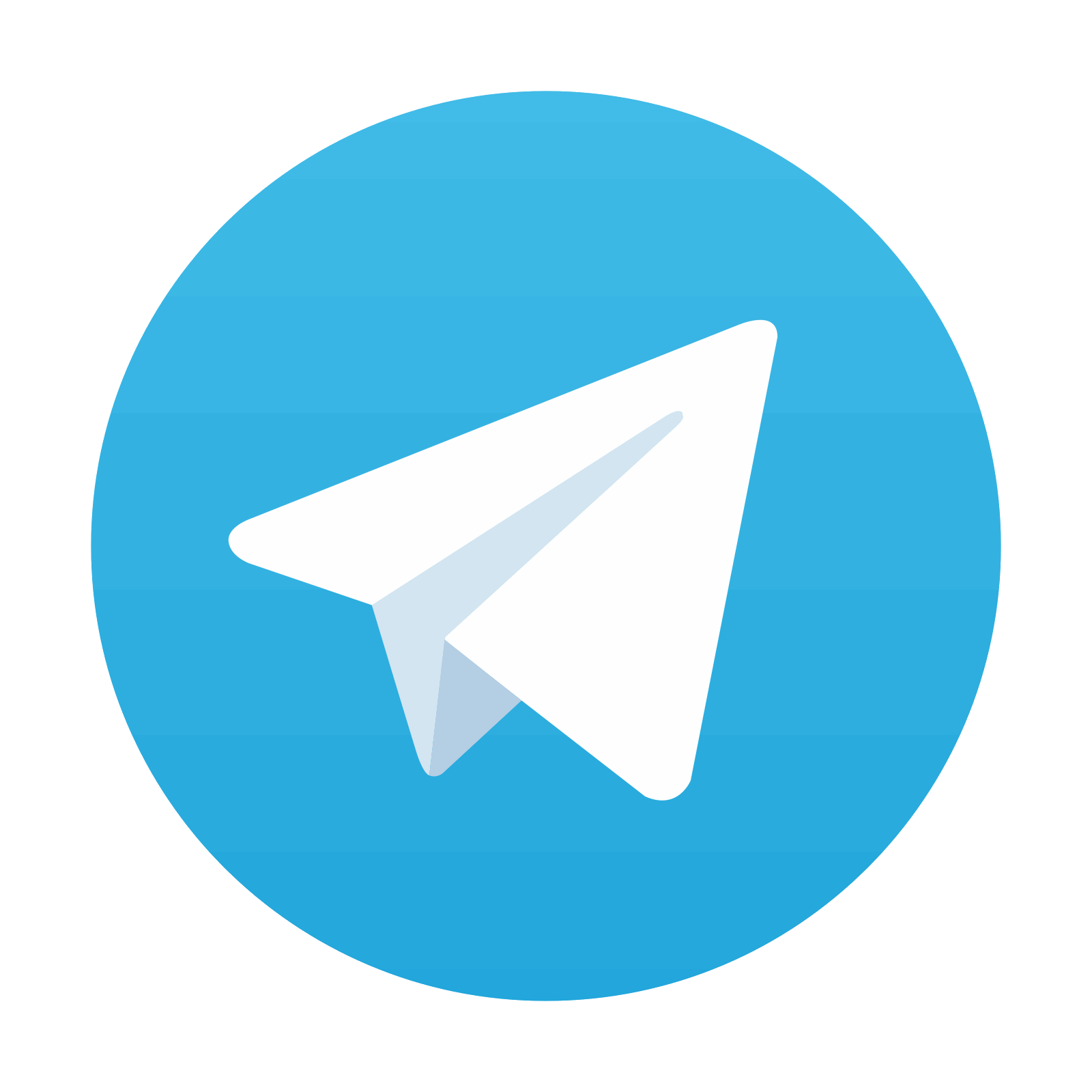
Stay updated, free articles. Join our Telegram channel

Full access? Get Clinical Tree
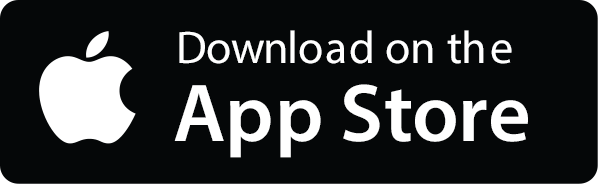
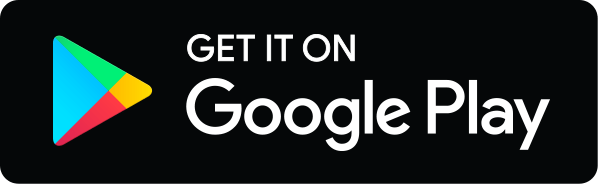