Animal Models of Acquired Epilepsies and Status Epilepticus
Giuliano G. Avanzini
David M. Treiman
Jerome Engel Jr.
Introduction
In animals, epilepsies with focal seizures can be faithfully reproduced by various experimental procedures that can induce an initial status epilepticus (SE) followed after a latent period by a chronic seizure recurrence. Thus, the initial SE and the subsequent chronic epilepsy can be considered the biphasic expression of a common pathogenetic process, which is set in motion by the experimental manipulation.
This chapter reviews models for SE and for epilepsies with focal seizures obtained using experimental epileptogenic procedures in normal animals. Some procedures are relevant to both focal epilepsies and SE, whereas others are specific for either one; therefore, SE and focal epilepsy models are dealt with in two separate sections. Models of epileptogenic dysplasia are also discussed.
In principle, animals presenting with spontaneous seizures can be profitably studied to gain information on species-specific susceptibility and pathophysiologic mechanisms, but, in practice, their interest is limited by the difficulties of obtaining homogeneous populations and the obvious differences in the ability to study domesticated or laboratory species versus those in the wild.
In most domesticated species, spontaneously occurring SE has never been observed, whereas reports of spontaneous localization-related epilepsies are mostly anecdotal and do not provide a basis for defining any suitable model for systematic experimental studies. An exception is the dog, in which prevalence of epilepsy approaches that of humans.64 Because there is evidence for genetically determined susceptibility to epilepsy and/or epileptogenic brain pathologies, canine epilepsies are not dealt with here. None of the other animal models of genetically determined epilepsies are included in this account because they also are models for generalized rather than focal epilepsies.
Reports of experimentally induced seizures in animals date back to the seventeenth century, when Robert Boyle24 observed the occurrence of seizures in sparrows, larks, cats, and mice exposed to low air pressure in a decompression chamber. At the cusp of the nineteenth and twentieth centuries, pioneering studies by Fritsch and Hitzig,81 Openchowski,163 Baglioni and Magnini,10 and later Kaufmann109,110 revealed that other physical and chemical means were effective in inducing epileptic manifestations, including cortical electrical stimulation, freezing, and the topical application of strychnine. Other agents later shown to be effective as topical convulsants include metallic compounds, which were studied for many years by Lenore and Nicholas Kopeloff after their first report,114 metabolic antagonists, and convulsant drugs (see Prince172 for review). The application of intracellular recording to experimentally induced cortical foci provided an early insight into the cellular mechanisms underlying epileptogenesis,134,135 and since then, a number of studies have considerably deepened our understanding of focal epileptogenesis in experimental animals and established a number of pathogenetic concepts that can now be reliably applied to human epilepsies.8,173
Experimental models provide a unique means of testing the efficacy of antiepileptic drugs and antiepileptogenic strategies, although the results may not always be generally applicable. Species-specific or mechanism-specific effects may, in fact, prevent their reproducibility in different species or in other types of experimentally induced or naturally occurring epilepsies.
Chemically Induced Focal Epilepsies
General Characteristics
Focal seizures are defined as those seizures whose symptomatology indicates the initial activation of a system of neurons limited to a part of one cerebral hemisphere.69 Epilepsy is defined as a chronic condition of the brain characterized by an enduring propensity to generate epileptic seizures,75 a definition that implies the tendency of recurrence of ictal manifestations over time. Thus, only animals with recurrent focal seizures can be considered as models for focal epilepsies.
In several instances, SE or closely spaced seizures are the initial event of a process leading to epileptic disorders presenting with recurrent seizures that occur spontaneously and do not require any further exogenously delivered precipitating stimulus. These characteristics correspond to those of human focal epilepsies, which are further classified as idiopathic (i.e., not preceded or caused by a neurologic disorder other than a possible hereditary predisposition), symptomatic (i.e., secondary to a known disorder of the central nervous system), or cryptogenic (i.e., secondary to a disorder whose course is hidden or occult). By definition, experimentally induced animal epilepsies fall into the category of symptomatic epilepsies because the causative factor (i.e., the experimental epileptogenic procedure) is always known.
The models reviewed in this section reproduce one important characteristic of human epilepsy, that is, its chronic course. They can be obtained by experimental manipulations that lead to a gradual development of persistent focal epileptogenic activities or by procedures capable of inducing acute epileptic manifestations that are able to set in motion a progressive epileptogenic process leading to a permanent epileptic condition. A typical example of the first possibility is topical application of alumina hydroxide gel, which induces progressive neuropathologic changes affecting cortical excitability, whereas the second possibility is best exemplified by the pilocarpine model,
in which the development of a chronic epilepsy is mediated by pilocarpine-induced SE. The role of the acutely induced limbic SE or closely spaced seizures is also important although not exclusive for kainic acid, whereas afterdischarges are relevant to epileptogenesis induced by focal electrical stimulation. Whether the epileptic manifestations occurring in the early phase contribute to the development of tetanus toxin–induced chronic epileptogenesis is not clear.
in which the development of a chronic epilepsy is mediated by pilocarpine-induced SE. The role of the acutely induced limbic SE or closely spaced seizures is also important although not exclusive for kainic acid, whereas afterdischarges are relevant to epileptogenesis induced by focal electrical stimulation. Whether the epileptic manifestations occurring in the early phase contribute to the development of tetanus toxin–induced chronic epileptogenesis is not clear.
Animal models of acute seizures and in vitro models are dealt with in other chapters.
Interictal and Ictal Clinical Phenomenology
Ictal clinical phenomena depend on the complex of anatomic structures involved in the generation of ictal discharges, referred to as the epileptogenic zone. In principle, the main types of seizures observed in human epilepsies should be faithfully reproduced in experimental animals by creating epileptogenic zones in the appropriate cortical areas. However, the evaluation of ictal phenomenology in animals is necessarily limited to external observations of animal behavior and thus provide only an indirect assessment of the subjective experience, which is an important aspect of seizures originating in cortical areas involved in sensation, emotion, and higher cognitive function.
The evaluation of experimentally induced complex partial seizures presenting with variable sequences of typical symptoms (including loss of contact) is also difficult, as is the comparison of animal and human seizures because of species-related differences in central nervous system organization. Of course, the ictal involvement of some human-specific functions such as language can never be modeled in experimental animals.
Besides these intrinsic difficulties, further problems in assessing the reproducibility of human seizures in experimental animals derive from the scanty descriptions of ictal phenomenology in some experimental studies. For instance, it is insufficient to describe a seizure as focal with elementary motor symptomatology without a qualitative (clonic vs. tonic, tonic vs. postural, positive vs. suppressive, etc.) and topographic (massive vs. segmental, stationary vs. migrating, etc.) analysis of its motor manifestations.
Neurologic, psychic, and neuropsychological persisting signs during the interictal period have been reported in patients suffering from focal symptomatic epilepsies. Interictal neurologic and behavioral studies in animals made epileptic by different experimental procedures have been performed only occasionally (see later discussion).
Interictal and Ictal Electroencephalographic Features
In human localization-related epilepsies, interictal focal “epileptiform” electroencephalographic (EEG) activities are often seen as spikes, sharp and slow waves, fast rhythms, and so on.53 The transition from an interictal to an ictal discharge may be represented by repetitive interictal discharges, localized fast activity (often with a recruiting character), or other rhythmic discharges not necessarily colocalized with the interictal focus (if present).53 Similar EEG changes can be detected in animal models of partial epilepsies, although the internal frequency of interictal and ictal discharges may not be the same as that seen in humans. This may depend on both species-related differences and technical factors (the type of electrodes, interelectrode distances, etc.). In particular, the current use of bone screws or intracranial wire as recording electrodes may exclude or reduce the filtering effect of bone on fast activities. To make recordings from animals comparable with those from humans, standardized EEG methodology and terminology should be developed.74
Natural History
Although human focal symptomatic epilepsies depend on various progressive, stationary, or remitting brain pathologies, their course is to some extent independent of the underlying disorder. In particular, the epilepsies secondary to acute brain insults (such as traumatic, vascular, and infectious epilepsies) display a typical biphasic course that includes an early phase with single or repeated “reactive”68 seizures or SE, which may then subside to give rise to chronic epilepsies after a more or less prolonged interval. Some of the experimental models reviewed here have contributed significantly to clarifying the mechanisms underlying such a biphasic course.
Questions that can be Addressed by Animal Studies
Experimental studies of epilepsies try to answer various questions concerning brain physiology, pharmacology, and pathophysiology that are all basically related to the aim of gaining a better understanding of the mechanisms underlying epileptogenesis.
The characterization of a cellular hallmark of epilepsy in the “penicillin” focus of cat neocortex, the paroxysmal depolarization shift (PDS),134,135 was seen as a major breakthrough that could directly lead to the unraveling of the “basic mechanism of epilepsy.” However, it was soon realized that the analysis of this characteristic burst-discharge seen in individual neurons only partially explains the biologic basis of epileptogenesis. Moreover, it became clear that similar shifts could result from a variety of experimental manipulations differentially affecting the excitable properties of cortical neurons, thus suggesting that a unitary explanation of epileptogenesis was unrealistic.172
Selection of a model for study depends on the specific question being asked. However, whether designed to replicate a specific component or an overall process, the ideal model is one that most closely approximates the phenomenon or process of interest. Thus, experimental models should closely approximate behavior, electroencephalographic characteristics, and pharmacologic responses of the type of human epileptic manifestation being studied.
In considering the questions to be addressed by a given experimental model, it must be borne in mind that the answers an investigator may obtain are valid only for that specific model unless otherwise demonstrated. Therefore, the relevance that an experimentally demonstrated epileptogenic mechanism may have in relation to human epilepsies depends on the strength of the evidence of its involvement in human epilepsies. Ultimately, therefore, parallel studies in patients are required.
The following is a discussion of some of the models that have been in use for many years. Others not discussed in detail that are being developed include models of traumatic brain injury, hypoxia-induced epileptogenic encephalopathy, stroke, febrile seizures, intraparenchymal bleeding, and cerebral infection.168
Alumina Model
The epileptogenic effect of the topical application of alumina hydroxide gel to monkey neocortex was discovered by Kopeloff et al.114 in the context of a study aimed at investigating
immunologic factors in epilepsy. Among the many other metallic compounds that were later shown to induce epileptic foci, alumina cream is the most suitable for inducing chronic focal epilepsy.
immunologic factors in epilepsy. Among the many other metallic compounds that were later shown to induce epileptic foci, alumina cream is the most suitable for inducing chronic focal epilepsy.
Methods
Autoclaved aluminum hydroxide 4% gel is injected in a few adjacent sites of the exposed neocortex using a small syringe needle.247 Epileptogenic foci are optimally induced in primates but less consistently in dogs, cats, and guinea pigs. There are no reports of spontaneous seizures induced by intracortical alumina in lower forms. Alternatively, the systemic76 and intracisternal111 administration of aluminum salt or aluminum metallic powder29 has been used to induce encephalopathy with multifocal seizures in susceptible animals (rabbits, cats, and ferrets, but not rats).
Neuropathology
Dendritic distortion with decreased branching size irregularities and spine loss has been observed in pyramidal neurons within an alumina cortical focus.250 These changes are due to a toxic effect leading to neurofibrillary degeneration, which has been studied in aluminum encephalopathy induced by both systemic and intracisternal administration.29,111 The further stages of toxic degeneration lead to neuronal loss with marked gliosis, which is particularly prominent in “mature” alumina cortical foci.102 A decrease in GABAergic nerve terminals at the sites of alumina foci has also been reported.178 In most experiments, alumina has been topically applied to the sensorimotor cortex or the hippocampus; in both regions, pyramidal neurons have been found to be consistently affected after the intracisternal injection of aluminum powder.29
Natural History and Clinical Phenomenology
Spontaneous clinical seizures appear between 2 weeks and 6 to 8 months after intracerebral injection of alumina cream, depending on the number of injections and the width of the involved area.247 Once established, the seizures spontaneously recur, probably throughout the life span of the animals; in monkeys, they have been observed for at least 7 years.247 With large foci, seizure frequency may increase to status epilepticus and require antiepileptic treatment to maintain a viable preparation. The seizure patterns depend on the location of the focus. According to Ward,247 in monkeys receiving alumina injections in the face and arm area of the somatomotor cortex, the seizures begin with contralateral facial or hand twitching, spread gradually to the entire contralateral side (mimicking a jacksonian march), and may eventually become generalized. During the seizures, muscular jerks occur at an increasing rate, which then fuse into a tonic contraction with superimposed strong generalized jerks, cyanosis, and sialorrhea. The tonic or tonic–clonic phase ceases abruptly, giving place to a postictal depression with hypotonia and unresponsiveness to external stimulation of variable duration. When a particularly intense epileptogenic focus has been obtained, focal motor status epilepticus can be observed, with continuous 1/10-second jerks of the contralateral face and hand, mimicking Kojeknikoff epilepsia partialis continua. Behavioral seizures reminiscent of human complex partial seizures of temporal origin can be observed after alumina injection in the anterior part of the temporal lobe and/or in the amygdala of monkeys and cats.83,251 Soper et al.203 consistently obtained chronic temporal lobe epilepsy in monkeys only by means of bilateral alumina injections in the hippocampus; the clinical seizures consisted of head-turning, lip-smacking, mastication, and salivation.
Electroencephalogram
Serial recordings show the gradual development of interictal foci of slow and sharp waves, spikes, and delta activity in the region of the scalp corresponding to the underlying alumina focus (Fig. 1).96 The transition to ictal discharge may be difficult to identify when interictal activity is sustained; otherwise, it is characterized by focal fast activity of increasing amplitude.202 As the seizure progresses, spikes and sharp waves tend to recur rhythmically and spread to other regions. In animals with bilateral foci, ictal discharges invariably begin at the site of the older focus.202
Pharmacology
Antiepileptic drug efficacy on interictal EEG discharges or seizure frequency has been widely studied in correlation with pharmacokinetic parameters.121 Phenytoin (Dilantin), phenobarbital (Arco-Lase, Bellergal-S, Donnatal, Quadrinal Mudrane, Rexatol, Solfoton), primidone (Mysoline), and carbamazepine (Atretol, Tegretol) showed a significant effect on both seizure frequency and interictal EEG discharge. The efficacy was correlated with drug plasma levels. A less clear level/effect correlation was found for sodium valproate (which improved when the correlation was evaluated on an hour-by-hour basis).
Pathophysiology
Neurons belonging to alumina foci have a high probability of burst-firing, closely associated with surface epileptiform waves.173 These putative epilepsy-related changes are not maintained in in vitro slices prepared from monkey alumina foci, which have been found to have physiologic properties that are not significantly different from those of control tissue.186 On the contrary, Franceschetti et al.77 observed significant changes in the excitable properties of hippocampal pyramidal neurons in slices prepared from intracisternally AlCl2-injected rabbit. These changes were accounted for by a decrease in the efficacy of Ca2+-dependent K+ conductances and GABAergic transmission and by electrotonic shortening due to dendritic debranching (Fig. 2). They were clearly detectable in the very early phases of the aluminum-induced encephalopathy, even before the manifestation of neurofibrillary degeneration, cell loss, and gliosis. On the other hand, the hypothesized epileptogenic role of the gliotic scar (which is believed to impair K+ regulation and thus lead to K+ accumulation in the interstitial space) is controversial102 and could not be directly demonstrated by Heinemann and Dietzel,100 who found that the spatial buffer capacity of gliotic tissue for K+ was not severely impaired in cat alumina cream foci.
Pilocarpine Model
The first evidence that rats with brain damage induced by the cholinergic agent pilocarpine develop spontaneous recurrent seizures after a silent period of 14 to 15 days was provided by Turski et al.,226 who were investigating the acute effects of pilocarpine treatment. As was stressed later by the same authors,34 this natural history is reminiscent of that of human temporal lobe epilepsy, which often begins with prolonged status epilepticus in infancy and develops with recurrent seizures in later life.89
Methods
Thirty minutes after subcutaneous pretreatment with scopolamine, 1 mg/kg (to minimize peripheral cholinergic effects), a single high dose of pilocarpine (300–380 mg/kg) is injected intraperitoneally in rats and mice.33 The acute pilocarpine-induced status epilepticus must be continuously monitored by behavioral observation and EEG recording because of the high mortality rate (30%),33 which can be partially prevented by repeated intraperitoneal injections of diazepam 10 mg/kg plus phenobarbital 30 mg/kg117 at 30 minutes 1, 2, and 6 hours after the beginning of the status epilepticus. Further behavioral-EEG monitoring is indicated during the silent period to detect the onset of chronic recurrent seizures.
Neuropathology
At the end of the acute phase, widespread bilateral morphologic changes appear that involve the hippocampus, amygdala, thalamus, pyriform and entorhinal cortices, neocortex, and substantia nigra.226 These consist of massive swelling of dendrites and neuronal cell bodies with relative sparing of axons and astroglial cell swelling.40,159 During chronic stages, there is invariably cell loss in all of the structures mentioned, including the hilus of the dentate gyrus. Clear-cut evidence of supragranular sprouting of the mossy fibers, beginning 4 days after the episode of status epilepticus and reaching a plateau after 100 days, can be found using Timm staining.152 The severity of the chronic neuropathologic changes is proportional to the duration of the status episode117 but not to the number of chronic seizures.120
Natural History and Clinical Phenomenology
The temporal evaluation of pilocarpine-induced behavioral and EEG changes is schematically illustrated in FIGURE 3. Immediately after the pilocarpine injection, the animal is hypoactive; subsequently, there is the appearance of facial automatisms, including chewing and eye blinking, followed by head bobbing and motor limbic seizures (forelimb clonus, salivation, and rearing on hind limbs). Generalized convulsions and limbic status usually occur 40 to 80 minutes after the injection, depending on the injected dose.33,120,226 After a silent period of 4 to 44 days, the spontaneous seizures that characterize the chronic period appear and recur with a variable frequency of 2 to 15 per month, with no evidence of spontaneous remission for at least 6 months.33
Electroencephalogram
During the acute phase, the electroencephalographic changes evolve from early surface low-voltage fast activity correlated with a theta hippocampal rhythm to high-voltage fast activity associated with spiking in the hippocampus and, finally, surface spiking activity that correlates with head bobbing.120 Limbic seizures are associated with high-frequency, high-voltage spike discharges that become continuous during limbic status. The EEG progressively normalizes at the end of the acute phase, although spontaneous spike discharges reappear after the silent period. The ictal electrographic discharges of the chronic period consistently originate in the hippocampus and subsequently spread to the cortical electrodes.33 FIGURE 4 summarizes the evolution of EEG changes.
Pharmacology
Diazepam (Dizac, Valium) and scopolamine, 10 mg/kg, provide effective protection from acute seizures and limbic status.33 Phenobarbitone 40 mg/kg/d, phenytoin 100 mg/kg/d, and carbamazepine 120 mg/kg/d have been found to be effective against the spontaneous seizures occurring during the chronic phase. Sodium valproate has been found to be effective only at very high doses (600 mg/kg/d); ethosuximide (Zarontin) 400 mg/kg/d is totally ineffective.116
Pathophysiology
In vitro experiments15,16 have shown that the epileptogenic effect of cholinergic agents depends on the facilitation of burst discharges in hippocampal pyramidal neurons by means of a block of the K+ transmembrane current IM. This mechanism explains the massive activation of hippocampal neurons during pilocarpine-induced status epilepticus, which leads to cell death, axonal sprouting, and a synaptic reorganization of hippocampal circuitry that results in permanent epileptogenic changes.33,35,120 The neuronal damage is not attributed to a direct toxic effect of pilocarpine, but to a seizure-related excitotoxic effect involving glutamate receptors and Ca2+ influx.
Tetanus Toxin
The epileptogenic properties of intracerebral tetanus toxin have been known since the end of the nineteenth century180 but have been exploited in experimental studies of epileptogenesis only more recently after studies published by Brooks and Asanuma28 and Carrea and Lanari32 in cat and dog neocortex and by Mellanby et al.152 in rat hippocampus and neocortex.106
Methods
Tetanus toxin is a protein with a molecular weight of 150 kDa released by Clostridium tetani and is now available in a purified form. It is quite stable and can be kept at 4°C for months. Tetanus toxin is extremely toxic and must be handled only by effectively immunized people wearing protective clothing (gloves and a mask). Tetanus toxin is active in all of the mammalian species tested180 when very small amounts are injected in appropriate regions. The dose is usually expressed in toxicologic units that correspond to different quantities, depending on the degree of purification: 5 to 20 mouse half lethal dose (LD50) has been used in rat hippocampus,106 2 to 30 minimum lethal dose in rat neocortex,24 10 to 103 mouse lethal dose in cat neocortex,25 and 28 to 83 LD50 in dog neocortex.32 It is important to bear in mind that tetanus toxin is very efficiently transported along axons.
Neuropathology
Early tetanus toxin–induced histologic changes with minimum effective doses injected into the hippocampus are very mild and consist of a loss of neurons in the CA1 region. Much higher doses cause neuronal loss at the injection site. It is suspected that late changes in hippocampal circuitry occur, but these have not yet been systematically investigated.106
Natural History and Clinical Phenomenology
Animals that are injected with low doses in either the neocortex or hippocampus develop seizures 3 to 7 days after the injection.27,106 Shorter intervals are reported with higher doses.28 In rats receiving hippocampal injections of the toxin, there is a reduction in the number of seizures after some weeks of intense epileptic activity; the seizures eventually disappear but may relapse in the longer term.106 In neocortex-injected rats27 and cats,125 tetanus toxin–induced foci can be permanent. Hippocampal injections induce limbic seizures that start with a behavioral arrest, followed by vibrissal and facial twitching and forelimb myoclonus that may eventually develop into a clonic–tonic seizure involving the hindlimbs. Injections in rat cortex are followed by early focal motor seizures in the jaws and/or contralateral limbs, tending to progress to generalized convulsive seizures after 16 hours.27
Electroencephalogram
Interictal biphasic sharp waves are recorded from the injected hemisphere 3 to 5 days after the injection and, a few days later, from the contralateral homotopic sites with synchronous or asynchronous expression (Fig. 5).27 Ictal discharges of 3- to 20-Hz spikes and spikes-and-waves may be limited to one hemisphere or may spread rapidly to the contralateral side. The injected hemisphere leads in the early phases, but 5 to 13 days after the injection, the ictal discharge can be initiated in the contralateral hemi-sphere.27
Pharmacology
Drugs effective in human focal epilepsies, such as carbamaze-pine, have also been found to be quite effective in the tetanus toxin model but not the N-methyl-D-aspartate (NMDA)–receptor antagonist 2-amino-5-phosphonopentanoic acid (APV).106
Pathophysiology
The tetanus toxin–dependent epileptogenic process has various phases. At the very beginning, the toxin induces epileptiform discharges in the injection site as a result of a block of γ-aminobutyric acid (GABA) release,46 leading to the impairment of the local inhibitory circuits established by GABA interneurons in both the hippocampus and the cerebral cortex. The epileptiform neuronal aggregate is contained in hippocampal and neocortical slices prepared from the injected areas, and this has allowed a detailed electrophysiologic analysis.67,105 In a second phase, the toxin is transported through the axons to even remote sites, where it can move transsynaptically inside local GABAergic neurons. A transport velocity of as much as 200 mm/d has been estimated in peripheral nerves,185 and, therefore, transport-related patches of epileptiform activity can be generated in a few hours through corticocortical and callosal connections (Fig. 6). This mechanism might account for the rapid generation of mirror foci.27 In a third chronic phase, GABA-mediated inhibition recovers, due probably to an increased RNA expression of glutamic acid dehydrogenase (GAD). The epileptic activity persisting in this chronic phase is attributed to seizure-induced plastic changes in the hippocampal or neocortical circuits, leading to the functional disconnection of GABAergic neurons or the sprouting of new excitatory axons.106
Kainic Acid
Kainic acid is a highly potent glutamate agonist that is obtained from the seaweed Digenea simplex and used as an ascaricide. Kainic acid was found to be excitatory when applied iontophoretically to rat cortex195 and to induce seizures when injected intracranially19,20 or systemically.21,47,126 Olney et al.160 highlighted its neurotoxic properties and reported its toxicity to be particularly prominent on the hippocampus, even when systemically injected. Kainic acid–induced cell injury is attributed to an excitotoxic mechanism triggered by the activation of excitatory amino acid (EAA) receptors. The same mechanism is also responsible for the hippocampal seizures occurring in animals injected, either intravenously126 or in the hippocampus,34 with doses less than those required to produce direct cell damage.
Methods
Most of the studies with kainic acid have been done in rats. Systemic doses of 4 mg/kg126 and local injections of 0.4 mg8 are effective in inducing persistent seizures. Similar effects have been obtained by means of intracisternal ventricular injections in mice,227 for which a 50% convulsive dose of 0.3 nmol has been calculated. A single intra-amygdaloid injection of 1 μg in cats has been found to be effective in inducing focal status epilepticus.126,214
Neuropathology
The systemic administration of kainic acid induces a variable pattern of cortical and subcortical damage involving the pyriform and entorhinal cortices, the hippocampus, the lateral septum, and several thalamic and amygdaloid nuclei.159 In the hippocampus, degenerating neurons are found in the dentate hilus, CA1, and CA3.74 A similar pattern of hippocampal degeneration has been found after local hippocampal injection during the acute phase,133 with evidence of active cellular phagocytosis of the necrotic zone in the next active phase. The Timm217 sulfide silver method for heavy metals, subsequently modified,54 shows an initial sprouting of zinc-containing mossy fibers into the inner molecular layer. This sprouting progressively increases during the latent phase, reaching its maximum at the beginning of the chronic phase, when a thick band of Timm-positive fibers is permanently found in the inner molecular layer (Fig. 7). With unilateral injection, the changes are largely unilateral, resembling human hippocampal sclerosis.
![]() FIGURE 7. Interictal recording from a rat bilaterally injected with kainic acid in the hippocampus 122 (A) and 130 (B) days before. Fr, single wire electrodes placed into the frontal cortex; HC1, 2, double-twisted wire electrodes inserted in the hippocampus; L, left; R, right. The independent epileptiform transient (arrows) are seen mostly in the left hippocampus, which showed evidence of mossy fiber sprouting, as shown by Fig. 8. (From Mathern GW, Cifuentes F, Leite JP, et al. Hippocampal EEG excitability and chronic spontaneous seizures are associated with aberrant synaptic reorganization in the rat intrahippocampal kainate model. Electroencephalogr Clin Neurophysiol. 1993;87:326–339; with permission.) |
![]() FIGURE 8. In the same animal whose electrographic recording is shown in Fig. 7, Timm-stained sections show a zinc-positive band (arrows) in the left (A) but not in the right (B) inner molecular layer (IML). Note that both hippocampi (C, D) show evidence of neuron loss in the stratum granulosum (SG) and hilus (H). (From Mathern GW, Cifuentes F, Leite JP, et al. Hippocampal EEG excitability and chronic spontaneous seizures are associated with aberrant synaptic reorganization in the rat intrahippocampal kainate model. Electroencephalogr Clin Neurophysiol. 1993;87:326–339; with permission.) |
Natural History and Clinical Phenomenology
The evolution of the epileptic phenomenology and electrophysiologic correlates induced by low doses of kainic acid injected
bilaterally in rat hippocampus has been longitudinally studied by Mathern et al.133 and correlated with concomitant histologic changes. Similar asymmetric changes occur with unilateral hippocampal injections, which more accurately reproduce the predominantly unilateral condition of human mesial temporal lobe epilepsy.25 On the basis of the clinical and electrophysiologic course, four phases have been recognized as sequentially occurring after kainic acid injection at the following approximate time intervals in days: acute (0–10), active (10–30), latent (30–90), and chronic. The earliest seizures characterizing the acute phase occur within 1 hour after hippocampal injection and consist of behavioral arrest; the association of sniffing and facial myoclonus is ascribed to the frontal spread of the discharge. After several hours, the seizures increase in duration and may eventually evolve into a partial status characterized by simple staring. The active phase is characterized by short-duration seizures (<1 minute) with generalized motor clonus and very rapid behavioral recovery. The seizures disappear during the latent period but relapse during the chronic phase, with characteristics similar to those of the acute stage: A motionless stare that may occasionally progress to a more complex phenomenology, including facial automatisms, forelimb clonus, and generalized clonic–tonic seizures. The chronic seizures do not tend to subside, but rather increase in frequency and duration and may generalize over time.133
bilaterally in rat hippocampus has been longitudinally studied by Mathern et al.133 and correlated with concomitant histologic changes. Similar asymmetric changes occur with unilateral hippocampal injections, which more accurately reproduce the predominantly unilateral condition of human mesial temporal lobe epilepsy.25 On the basis of the clinical and electrophysiologic course, four phases have been recognized as sequentially occurring after kainic acid injection at the following approximate time intervals in days: acute (0–10), active (10–30), latent (30–90), and chronic. The earliest seizures characterizing the acute phase occur within 1 hour after hippocampal injection and consist of behavioral arrest; the association of sniffing and facial myoclonus is ascribed to the frontal spread of the discharge. After several hours, the seizures increase in duration and may eventually evolve into a partial status characterized by simple staring. The active phase is characterized by short-duration seizures (<1 minute) with generalized motor clonus and very rapid behavioral recovery. The seizures disappear during the latent period but relapse during the chronic phase, with characteristics similar to those of the acute stage: A motionless stare that may occasionally progress to a more complex phenomenology, including facial automatisms, forelimb clonus, and generalized clonic–tonic seizures. The chronic seizures do not tend to subside, but rather increase in frequency and duration and may generalize over time.133
Electroencephalogram
Mathern et al.133 and Bragin et al.25 monitored the hippocampal activity recorded by stereotaxically implanted deep electrodes and the frontal activity derived from intracortical wires.
In the acute phase, discharges of spikes and multispikes in the hippocampus are associated with behavioral arrest and spread of the discharges toward the frontal cortex with sniffing and facial myoclonus. Postictal diffuse slowing of background activity is regularly observed and is sometimes associated with periodic lateralized epileptiform discharges (PLEDs). The active phase is characterized by interictal fast activity and polyspikes limited to the hippocampus, the transition to the ictal discharge being marked by a pronounced spike activity that rapidly generalizes. During the latent phase, there is a reduction in the interictal spikes, and background activity is almost normalized; in the late part of the latent phase, however, spikes and sharp waves are increasingly recorded, and there is a marked tendency to asynchronous expression in both hippocampi. The asynchrony of interictal spikes and sharp waves becomes more and more evident during the chronic phase, when ictal discharges limited to one hippocampus reappear in association with motionless staring episodes. A bilateral spread of discharges is observed during generalized seizures (Fig. 8). In addition to the clinical seizures, more frequent electrographic seizures can also be recorded, usually during sleep.25 Whereas the EEG patterns during clinical seizures resemble the low-voltage fast ictal onset of human mesial temporal lobe epilepsy, the electrographic events resemble the hypersynchronous onsets usually associated with auras in patients.
In the acute phase, discharges of spikes and multispikes in the hippocampus are associated with behavioral arrest and spread of the discharges toward the frontal cortex with sniffing and facial myoclonus. Postictal diffuse slowing of background activity is regularly observed and is sometimes associated with periodic lateralized epileptiform discharges (PLEDs). The active phase is characterized by interictal fast activity and polyspikes limited to the hippocampus, the transition to the ictal discharge being marked by a pronounced spike activity that rapidly generalizes. During the latent phase, there is a reduction in the interictal spikes, and background activity is almost normalized; in the late part of the latent phase, however, spikes and sharp waves are increasingly recorded, and there is a marked tendency to asynchronous expression in both hippocampi. The asynchrony of interictal spikes and sharp waves becomes more and more evident during the chronic phase, when ictal discharges limited to one hippocampus reappear in association with motionless staring episodes. A bilateral spread of discharges is observed during generalized seizures (Fig. 8). In addition to the clinical seizures, more frequent electrographic seizures can also be recorded, usually during sleep.25 Whereas the EEG patterns during clinical seizures resemble the low-voltage fast ictal onset of human mesial temporal lobe epilepsy, the electrographic events resemble the hypersynchronous onsets usually associated with auras in patients.
More recently, microelectrode recordings from unilateral intrahippocampal kainic acid–treated rats have led to the discovery of 150- to 500-Hz “Fast Ripple” oscillations identical to those recorded from human epileptic hippocampus.25 Fast Ripples can also be recorded shortly after kainate injection and predict which rats will develop spontaneous seizures.26 The significance of this novel finding is discussed in Chapter 13.
Pharmacology
Drug responsiveness of the kainate model is essentially the same as that for the pilocarpine model.
Pathophysiology
![]() FIGURE 10. Different susceptibility to electrically induced afterdischarge in the cortex of monkey. Stimulation thresholds in volts (V) in different areas are indicated by the three coded patterns. (Originally from French et al.80 From Ajmone Marsan C. Focal electrical stimulation. In: Purpura DP, Penry JK, Tower D, et al., eds. Experimental Models of Epilepsy. A Manual for the Laboratory Worker. New York: Raven Press; 1972:147–172; with permission.) |
The early seizures occurring during the acute phase are attributed to the direct effect of the excitatory amino acid receptor agonist kainic acid, which is especially prominent in the hippocampus due to the particularly high concentration of kainic acid receptors in this region. The permanent changes in hippocampal excitability underlying chronic seizures is ascribed to neuronal damage of the vulnerable hilar mossy cells due to kainic acid excitotoxicity or the ensuing sprouting of mossy fibers. The first effect is thought to lead to a selective denervation of the neurons that mediate granule cell inhibition (Fig. 9), thus increasing their excitability.199 On the other hand, the newly formed recurrent mossy fiber collaterals could re-excite the dentate granule cells through newly formed synapses.215 The role of these two putative epileptogenic mechanisms will be further discussed later.
Electrical-Stimulation–Induced Focal Epilepsies
Self-Sustained Status Epilepticus
The possibility of eliciting epileptic manifestations by means of the repeated electrical stimulation of discrete regions of the central nervous system has been known since the nineteenth century following the experimental studies of Fritsch and Hitzig,81 Ferrier,73 and Luciani.128 The analysis of the electrographic correlates of stimulation-induced seizures showed that the associated discharge may outlast the end of the stimulation train.3 Although self-sustained, this electrically evoked afterdischarge is still a stimulation-dependent acute epileptic phenomenon. Later, Alonso-De Florida and Delgado5 discovered that appropriate paradigms of repeated stimulation may induce permanent changes in excitability and lead to the recurrence of spontaneous seizures. Electrogenic models of SE are discussed later in the SE section.
Methods
The optimal stimuli for the production of afterdischarges are 4- to 6-second trains of repeated (25–60 Hz) 2.5- to 8.0-mA, 2- to 5-msec diphasic square pulses.4 After a first effective train, a second afterdischarge can be obtained only after a delay of at least 15 seconds. Using more prolonged and/or intense stimulating trains, Lothman et al.126 and Handforth and Ackermann93 overcame this refractory period and reduced the intertrain interval to 0.5 second by using a protocol currently defined as “continuous” hippocampal stimulation. Focal electrical stimulation has been found to be effective in all studied species, including humans. The greatest susceptibility is in the hippocampus and amygdala. All of the neocortical areas can generate afterdischarges, the most and least prone regions being the precentral motor area and the temporal gyri (Fig. 10).4
Neuropathology
By definition, afterdischarges are evoked in normal tissue, which should not be directly damaged by the stimulation procedure, provided appropriate parameters are used. However, Sloviter and Damiano201 and Sloviter198 found that indirect damage to hilar mossy cells can be induced by the stimulation of a perforant path capable of inducing repetitive discharges in their target granule cells, which innervate the hilar mossy cells themselves. In addition, there is evidence that, during repeated stimulation, use-dependent structural rearrangements of the involved neuronal network occur (e.g., mossy fiber sprouting) that are similar to those observed in other chronic models of hippocampal epilepsy.17
Natural History and Clinical Phenomenology
The stimulation-induced afterdischarge can be purely electrical or associated with clinical manifestations appropriate to the functional properties of the involved areas. Continuous hippocampal124 or amygdala stimulation93 leads to self-sustained epileptic states defined as “immobile, exploratory, minor convulsive, and clonic” according to a behavioral-electrographic hierarchy of severity.94 After a latent period, as
with pilocarpine and kainic acid, animals exhibit spontaneous seizures.
with pilocarpine and kainic acid, animals exhibit spontaneous seizures.
Electroencephalogram
The electrographic activity characterizing typical afterdischarges becomes evident 1 to 2 seconds after the end of the stimulating train. It begins as 15- to 30-Hz low-voltage oscillations, which progressively increase in amplitude and decrease in frequency, leading to large voltage oscillations (Fig. 11). The episode lasts from 10 to 90 seconds and ends abruptly, giving way to a postictal depression lasting from 2 to 15 seconds.4
Pharmacology
The effect of antiepileptic drugs can be tested on the threshold or duration of the afterdischarge. Carbamazepine, phenobarbital, and diazepam, but not sodium valproate, ethosuximide, or phenytoin, have been found to be effective on the maximal dentate activation obtained by means of contralateral region stimulation.209
Pathophysiology
The self-sustained character of the afterdischarge has always been attributed to a failure in inhibitory mechanisms,4 a view that was subsequently supported by the perforant path stimulation experiments of Sloviter and Damiano201 and Sloviter.198 The hyperexcitable state, leading to the facilitation of afterdischarges, electrogenic status epilepticus,93,126 and, eventually, the epileptogenic process, is the result of complex changes involving cell excitability and circuitry rearrangements that are discussed for other models (see also next section on kindling).
Kindling
Although physiologic psychologists carrying out electrical stimulation studies in rats had noted for some time that a few eventually developed seizures, this was considered a nuisance because it disrupted their research paradigms. Goddard, however, recognized this to be an interesting phenomenon in itself and developed the concept of kindling.90,91 Electrical kindling refers to the process of brief subthreshold brain stimulation that, when repeated, gradually results in ictal behaviors. Stimulation-induced seizures then persist indefinitely after the kindling process is discontinued. Electrical kindling, therefore, was an ideal mechanism for bringing the process of epileptogenesis under laboratory control, and it rapidly became the most commonly used animal model for studying basic mechanisms of epilepsy. Kindling as usually practiced, however, is not a model for chronic epilepsy because seizures do not occur spontaneously but need to be provoked by electrical stimulation. Although animals kindled for prolonged periods of time after maximal seizures occur eventually do develop spontaneous seizures,165 this model of chronic epilepsy is rarely used because of the time required and the difficulty in maintaining viable animals. Consequently, although kindling remains a useful model for the study of epileptic phenomena, particularly with respect to the limbic system,49,50,230,231,232,233,234 it has now been largely replaced by the excitotoxic and stimulation-induced self-sustained status epilepticus models that more faithfully
reproduce human mesial temporal lobe epilepsy with hippocampal sclerosis.
reproduce human mesial temporal lobe epilepsy with hippocampal sclerosis.
“Chemical kindling” refers to the process of repeated treatments with chemoconvulsants such as metrazol or carbacol, which, like electrical kindling, eventually results in epileptic seizures in response to the chemical treatment.87 Although chemical kindling presumably produces many of the same epileptogenic plastic changes induced by electrical kindling, the former models have been less well studied.
Methods
The classical kindling model involves electrical stimulation of the amygdala, although stimulation of hippocampus, piriform cortex, and other limbic structures results in the same epileptogenic phenomenology.145 The time course of kindling differs depending on the site of stimulation, stimulus parameters, interstimulation intervals, and species. Kindling can also be achieved with stimulation of nonlimbic brain areas such as neocortex, thalamus, and caudate, but this is associated with a different behavioral evolution and less consistent progression than limbic kindling. Many other structures, such as brainstem and cerebellum, however, do not support kindling.
Typically, limbic kindling begins with a stimulus intensity that produces afterdischarge without behavioral effects. With repeated stimulation, the afterdischarge duration increases and ictal behaviors begin with arrest, ipsilateral eye blinking, and chewing (stage 1), followed over time by head bobbing (stage 2), then forelimb clonus (stage 3), rearing (stage 4), and falling with generalized tonic–clonic movements (stage 5).176 It is also possible to begin kindling with subthreshold stimulation intensity (below that necessary to induce afterdischarges), in which case repeated stimulation eventually produces afterdischarges, which then reiterate the electrographic and behavioral epileptogenic progression.175 The usual stimulation parameters are 60 to 100 Hz delivered for 1 second, although slower frequencies and different durations are also effective. The time required for kindling to stage 5 depends on the area stimulated and the interval between stimulations. A typical paradigm involves stimulation for 1 second once a day, which requires approximately 2 weeks for amygdala kindling and 4 weeks for hippocampal kindling.
Neuropathology
Although limbic kindling is associated with mossy fiber sprouting, resembling this pathologic finding in human hippocampal sclerosis and chronic animal models of this disorder,210 cell death, particularly in the dentate hilus, is minimal. Neurogenesis and astrocytic proliferations have also been reported, but there are no neuropathologic findings specific to kindling.211
Natural History and Clinical Phenomenology
Kindling is not a single phenomenon, but a series of phenomena that underlie a progressive epileptogenic process. With subthreshold kindling, for instance, there are changes that occur only at the tips of the stimulating electrodes that eventually result in the appearance of afterdischarge. As stimulation continues, or with classical kindling, the increase in duration of afterdischarge also involves epileptogenic plastic changes at the electrode tips, but most likely in surrounding tissue as well. The appearance of clinical signs reflects propagation of epileptiform afterdischarge to distant structures, requiring transsynaptic alterations. This process evolves to recruit primary motor cortex and other brain areas, eventually engaging brainstem systems responsible for tonic–clonic seizures. The progressive transsynaptic recruitment is not merely a reflection of more intense afterdischarge at the electrode tips, but true synaptic plasticity, because stage 5 seizures, once achieved, almost always occur with any stimulation at the kindling site capable of inducing afterdischarge. Many widespread areas of the brain, therefore, develop an enduring epileptogenic potential with focal kindling. If kindling proceeds for months, spontaneous seizures eventually appear, and these typically do not originate at the site of kindling stimulation but from their efferent projection areas.165
Other enduring distant effects of kindling also occur. For instance, “transfer” refers to the fact that whereas it may take 2 weeks of daily stimulation to reach a stage 5 seizure with unilateral amygdala kindling, stage 5 seizures can then be provoked with only a few stimulations of the contralateral amygdale.144 On the other hand, contralateral kindling has an interhemispheric seizure-suppressing effect, in that it might take several additional days of stimulation to reestablish a stage 5 seizure with stimulation of the primary site.144 Ipsilateral seizure facilitating and seizure-suppressing effects can also be demonstrated with electrical kindling. Other evidence of kindling-related seizure suppression also exists during the postictal period. There is a refractory period after kindled seizures during which time another stimulation will not generate a seizure. Animals that have been subjected to frequent seizures during a relatively short period of time develop postictal seizure refractoriness that can last weeks.155 Investigations into this phenomenon could provide insights into natural homeostatic mechanisms that protect against recurrent seizures.
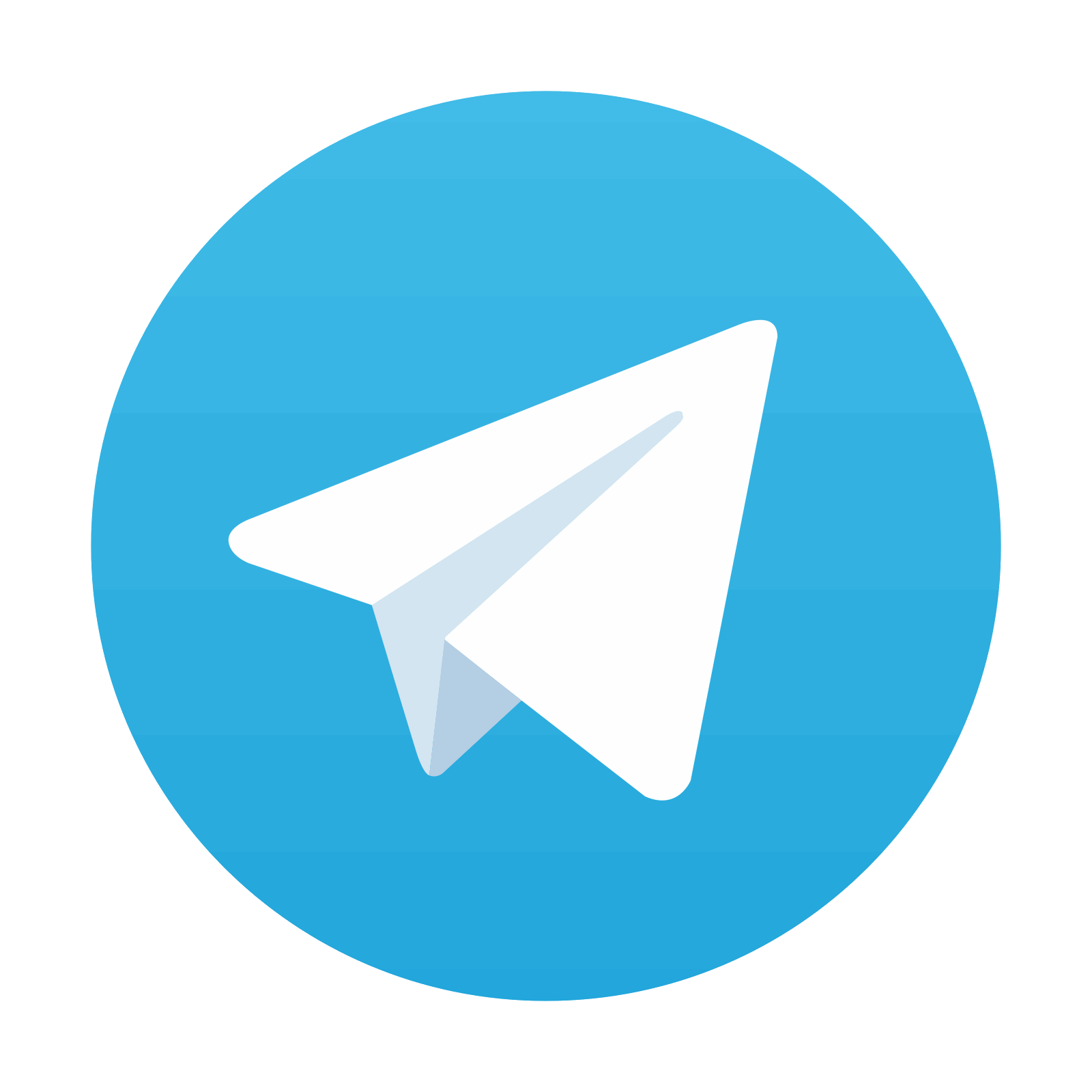
Stay updated, free articles. Join our Telegram channel

Full access? Get Clinical Tree
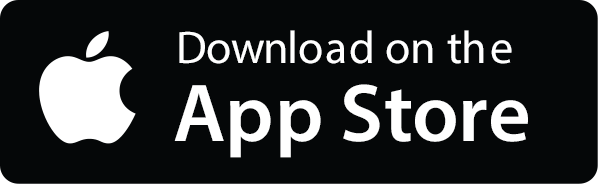
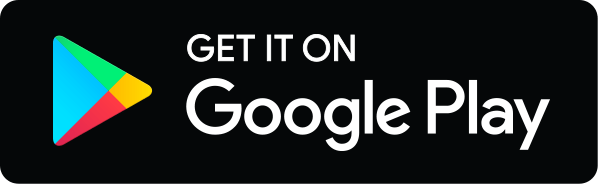
