Application of Electroencephalography in the Diagnosis of Epilepsy
David R. Chabolla
Gregory D. Cascino
The electroencephalogram (EEG) is the most frequently performed neurodiagnostic study in patients with a seizure disorder (1) and, despite its introduction more than 60 years ago (2), still has important clinical and research applications. Because of the paroxysmal nature of epilepsy (1,3), an EEG is usually obtained between seizure episodes. The interictal EEG may be useful in confirming the diagnosis of epilepsy, classifying seizure type, and monitoring and predicting response to treatment (1,4, 5, 6, 7, 8, 9, 10, 11, 12, 13). Localized electrographic alterations such as continuous focal slowing and certain epileptiform discharges may even “suggest” the presence of underlying pathology (14,15). Correlation of the ictal and interictal EEG changes with ictal semiology underlie the current classification of seizures (1,16).
When routine interictal EEG recordings prove diagnostically inadequate (14,17), long-term EEG studies may be used to improve the yield of EEG abnormalities and to localize the epileptogenic zone (14,17).
This chapter discusses the relationship between extracranial EEG studies and epilepsy and the clinical applications of interictal and ictal EEG recordings.
HISTORICAL PERSPECTIVE
In 1933, Berger (18) published his observations of EEG changes in patients during “convulsions” but failed to recognize the tremendous potential of these studies in epilepsy. EEG findings appeared to validate Jackson’s earlier hypothesis that epilepsy was caused by a “discharging lesion” (19). In 1935, Gibbs and colleagues (20) documented the association of specific interictal and ictal EEG alterations in patients with seizures. They also indicated that interictal EEG findings may localize the epileptogenic zone (21). Various interictal EEG patterns in patients with partial and generalized epilepsy were subsequently recognized, and attempts were made to classify seizures according to electroclinical correlations (20). Penfield and Jasper (9) later revealed the importance of electrocorticography in recording interictal EEG abnormalities during focal cortical resective surgery for intractable partial epilepsy.
CLINICAL APPLICATIONS
Rationale
Despite impressive technical advances, the purpose of the EEG has not changed since the days of Gibbs and Gibbs (21). As the cornerstone of the evaluation of patients with episodic symptoms and recurrent behavioral alterations (1,16), the EEG identifies specific interictal or ictal abnormalities that are associated with an increased epileptogenic potential and correlate with a seizure disorder (16). A persistently normal EEG recording does not exclude the diagnosis of epilepsy (3), and epileptiform alterations may occur without a history of seizures (22), although this happens rarely. Ultimately, EEG findings must be correlated with the clinical history.
The patient with recurrent clinical symptoms, for example, staring spells with behavioral arrest, presents a diagnostic
challenge. A variety of physiologic and psychological disorders may mimic epileptic seizure activity (1). The sensitivity and specificity of the EEG in this setting depends on the classification of the seizure disorder, seizure type and frequency, and localization of the epileptic brain tissue (3,17). Epileptiform discharges are usually highly specific in the patient with episodic behavioral alterations; unfortunately, the EEG has variable sensitivity in epilepsy (3), and identification of the ictal patterns may be needed to clarify the underlying etiology. The absence of interictal epileptiform alterations does not distinguish a nonepileptic disorder from epilepsy, and false interpretation of nonspecific changes with hyperventilation or drowsiness may lead to an error in diagnosis and treatment. In patients who present with a behavior consistent with epilepsy, an EEG can elucidate the classification of a partial or generalized disorder that often cannot be determined by ictal semiology alone. The appropriate classification affects subsequent diagnostic evaluation and therapy, and may have prognostic importance. For example, recurrent secondarily generalized tonic-clonic seizures and anterior temporal lobe interictal spike discharges would be classified as a partial seizure disorder and set the stage for magnetic resonance imaging and antiseizure drug therapy.
challenge. A variety of physiologic and psychological disorders may mimic epileptic seizure activity (1). The sensitivity and specificity of the EEG in this setting depends on the classification of the seizure disorder, seizure type and frequency, and localization of the epileptic brain tissue (3,17). Epileptiform discharges are usually highly specific in the patient with episodic behavioral alterations; unfortunately, the EEG has variable sensitivity in epilepsy (3), and identification of the ictal patterns may be needed to clarify the underlying etiology. The absence of interictal epileptiform alterations does not distinguish a nonepileptic disorder from epilepsy, and false interpretation of nonspecific changes with hyperventilation or drowsiness may lead to an error in diagnosis and treatment. In patients who present with a behavior consistent with epilepsy, an EEG can elucidate the classification of a partial or generalized disorder that often cannot be determined by ictal semiology alone. The appropriate classification affects subsequent diagnostic evaluation and therapy, and may have prognostic importance. For example, recurrent secondarily generalized tonic-clonic seizures and anterior temporal lobe interictal spike discharges would be classified as a partial seizure disorder and set the stage for magnetic resonance imaging and antiseizure drug therapy.
The EEG has fundamental value in evaluating surgical candidacy and determining operative strategy (23) in selected patients with intractable partial epilepsy (24). In these individuals, interictal epileptiform alterations identified on EEG provide only limited information about lateralization and localization of the epileptic brain tissue (3,14,17), as the diagnostic yield depends on the site of seizure onset (3,17,25). Identification of ictal EEG patterns, performed in an inpatient unit with concomitant video recordings (3,14,17,23,25,26), is necessary to localize the epileptogenic zone preoperatively.
Methods
Recordings should be performed according to the methodology established by the American Clinical Neurophysiology Society (formerly the American Electroencephalography Society) (27). Standard activation procedures such as hyperventilation and photic stimulation should be included. The recording of drowsiness and nonrapid eye movement (NREM) sleep, facilitated by sleep deprivation, may increase the sensitivity of the EEG to demonstrate interictal epileptiform alterations, especially in patients with partial epilepsy (28,29). Adequate levels of sleep may be attained after administration of chloral hydrate. Benzodiazepines should not be used as a sedative because of their associated increase in β activity and the possible masking of epileptiform alterations. During the recording, the EEG technologist should obtain information about seizure manifestations, time of the latest seizure, current medications and antiepileptic drug levels, and precipitating events.
Interictal Recording
The recording of interictal epileptiform activity depends on the seizure type, localization of the epileptogenic zone, recording methodology, age at seizure onset, and frequency of seizure activity (1,10,28, 29, 30, 31, 32). The diagnostic yield of the interictal EEG can be increased by performing multiple EEG recordings (30), increasing the duration of the EEG, and timing a study shortly after a seizure, because interictal epileptiform discharges may be potentiated following an attack (31, 32, 33).
Ictal Recording
Recognition of ictal EEG patterns is indicated in patients with medically refractory partial epilepsy being considered for surgical treatment. The effectiveness of scalp-recorded ictal EEG for identifying the seizure onset zone may be enhanced by altering the recording technique (3,6,14, 15, 16, 17, 23,26,34,35). Closely spaced scalp electrodes increase the diagnostic accuracy of such monitoring (36). With application of the standard extension of the 10-20 Electrode Placement System, as outlined in the American Clinical Neurophysiology Society guidelines, inferolateral temporal electrode positions, namely, F9, F10, T9, and T10, may be used to record epileptiform activity of anterior temporal lobe origin (34). Special extracranial electrodes also may improve diagnostic effectiveness. Sphenoidal electrodes may reveal the topography of interictal and ictal epileptiform discharges in patients with temporal lobe seizures and indicate the mesial temporal localization of the epileptogenic region (14). Supraorbital electrodes, which record from the orbitofrontal region, may be useful in patients with partial epilepsy of frontal lobe origin (3). Digital EEG acquisition and storage in a format suitable for subsequent remontaging and filtering have improved the speed with which interpretable ictal recordings may be obtained over paper recordings.
Limitations of Extracranial Recordings
Several limitations of scalp-recorded, or extracranial, EEG affect the interpretation of these studies in patients with epilepsy (8). Epileptiform activity generated in cortex remote from the surface electrodes, for example, amygdala and hippocampus, may not be associated with interictal extracranial EEG alterations (8). Attenuation of spike activity by the dura, bone, and scalp also limits the sensitivity of these recordings (1), which may be degraded further by muscle artifacts. Approximately 20% to 70% of cortical spikes are recorded on the scalp EEG (37). Patients with seizure disorders may have repetitively normal interictal EEG studies (1,8,30). Extracranial EEG recordings may also inaccurately localize the epileptogenic zone (17). For example, interictal scalp EEG may fail to detect specific alterations arising from the amygdala only to reveal distant,
more widespread cortical excitability (8,17). Localization of the epileptogenic zone as suggested by interictal specific EEG patterns may also be discordant with ictal extracranial or long-term intracranial monitoring (17,38).
more widespread cortical excitability (8,17). Localization of the epileptogenic zone as suggested by interictal specific EEG patterns may also be discordant with ictal extracranial or long-term intracranial monitoring (17,38).
Pitfalls in Interpretation
Differentiating artifact from electrical activity of cerebral origin is challenging (1), and both the EEG technologist and the electroencephalographer must be constantly alert. Various types of artifacts can superficially mimic interictal and ictal epileptiform patterns. Artifacts can be related to extrinsic factors (such as the electrical interference generated by power cables and fluorescent lights), biologic factors (for example, myogenic and eye movement artifacts), and technological factors (such as poor electrical impedance causing an electrode “pop”). Changes during drowsiness, hyperventilation, photic stimulation, and arousal from sleep can be particularly confounding in pediatric patients. Thus, knowledge of the normal EEG background for age is critical to appropriate interpretation.
Some EEG patterns—such as small sharp spikes (see Fig. 13.4), 14- and 6-Hz positive bursts (see Fig. 13.5), 6-Hz spike and wave (see Fig. 13.6), wicket waves, rhythmic temporal θ activity of drowsiness, psychomotor variant pattern, and subclinical rhythmic epileptiform discharges of adults—are not associated with increased epileptogenic potential (11,29,39, 40, 41, 42, 43).
SPECIFIC INTERICTAL EPILEPTIFORM PATTERNS IN PARTIAL EPILEPSIES
EEG abnormalities in patients with seizure disorders may be categorized as specific or nonspecific. Specific patterns, that is, the spike, sharp wave, spike-wave complex, temporal intermittent rhythmic delta activity (TIRDA), and periodic epileptiform discharges (PLEDs), are potentially epileptogenic and provide diagnostically useful information (8,44). Nonspecific changes, such as generalized or focal slow-wave activity and amplitude asymmetries, are not unique to epilepsy and do not indicate an increased epileptogenic potential (8). Potentially epileptogenic EEG alterations identified in patients with seizure disorders are rarely detected in nonepileptic patients (22,45). Interictal epileptiform alterations identify the irritative zone that may mark the epileptic brain tissue (46). Patients with seizures beginning in childhood typically display a higher incidence of EEG abnormalities than do those with adult-onset epilepsy (30).
Spikes and Sharp Waves
The main types of epileptiform discharges are spikes and sharp waves, occurring either as single potentials or with an after-following slow wave, that is, a spike-and-wave complex. Spike discharges are predominantly negative transients easily recognized by their characteristic steep ascending and descending limbs and duration of 20 to 70 msec (47). Sharp-wave discharges are broader potentials with a pointed peak that last between 70 and 200 msec (47). These abnormalities should have a physiologic potential field and should involve more than one electrode to exclude electrode artifact. Spike-and-wave complexes consist of a spike followed by a slow wave in an isolated or repetitive fashion. The mechanisms of epileptogenesis (Chapter 6), atlas of pathologic substrates (Chapter 1), and the neurophysiologic bases of the EEG (Chapter 10) are discussed elsewhere in this volume. The morphology and localization of specific EEG epileptiform patterns may provide useful information on the predictability of seizure recurrence and may influence the selection of antiepileptic drug therapy. Unfortunately, interictal EEG may produce variable results, and during brief recording periods, specific abnormalities may not be seen despite repetition of several EEGs (30). The frequency of spiking may not be a good predictor of the seizure activity and is independent of antiepileptic drug levels (31,32).
Temporal Intermittent Rhythmic Delta Activity
The interictal potentially epileptogenic pattern TIRDA (Fig. 12.1) has been identified in patients with partial epilepsy of temporal lobe origin (11,44) and has the same epileptogenic
significance as temporal lobe spike or sharp-wave discharges (44). This pattern, most prominent during drowsiness and NREM sleep, consists of rhythmic trains of low- to moderate-amplitude δ frequency slow waves over the temporal region, unilaterally or bilaterally, without apparent clinical accompaniment (11,44). Persistent polymorphic δ frequency activity over the temporal region due to a focal structural brain lesion may not be potentially epileptogenic and should be differentiated from TIRDA.
significance as temporal lobe spike or sharp-wave discharges (44). This pattern, most prominent during drowsiness and NREM sleep, consists of rhythmic trains of low- to moderate-amplitude δ frequency slow waves over the temporal region, unilaterally or bilaterally, without apparent clinical accompaniment (11,44). Persistent polymorphic δ frequency activity over the temporal region due to a focal structural brain lesion may not be potentially epileptogenic and should be differentiated from TIRDA.
Periodic Lateralized Epileptiform Discharges
The focal or lateralized sharp-wave discharges called PLEDs may have a wide field of distribution and occur in a periodic or quasi-periodic fashion (Fig. 12.2) (48). Typically occurring at 0.5 to 2.0 Hz, they vary in amplitude and duration (100 to 200 msec) and most commonly appear as broad diphasic or triphasic waves (11,49), although complexes of repetitive discharges also may be seen (50). This EEG pattern is not specific for any one pathologic lesion and is usually transient (11); however, chronic, persistent PLEDs have been reported (51). Alteration of consciousness, focal or secondarily generalized seizures, and acute or subacute neurologic dysfunction may be associated with PLEDs (11). The prognosis depends mainly on the underlying etiology.
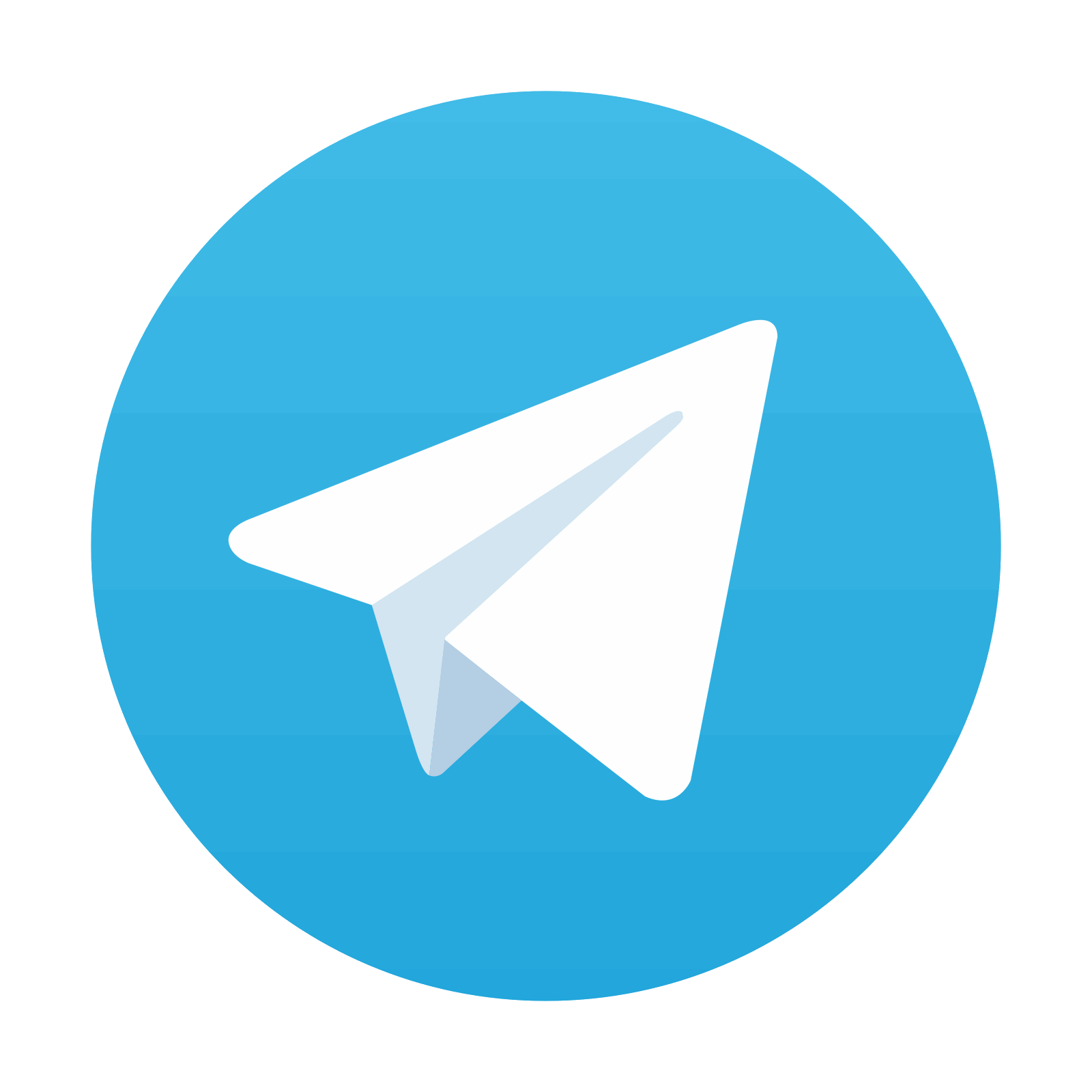
Stay updated, free articles. Join our Telegram channel

Full access? Get Clinical Tree
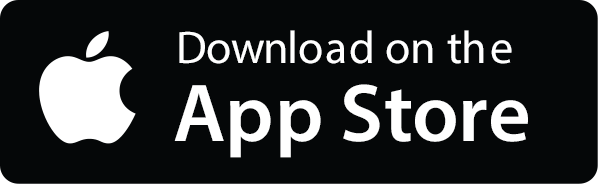
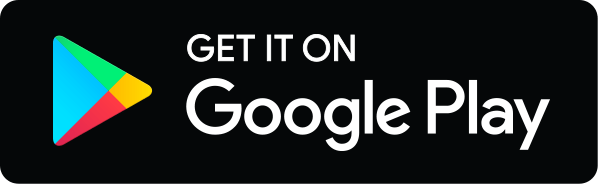