51CHAPTER 4
Artifacts of Neuromonitoring
Jason Siegel and David Freeman
INTRODUCTION
The neurocritical care unit (NCCU) houses patients with the ability for rapid neurological decompensation, often requiring continuous monitoring with clinical and neurophysiologic monitoring technology. In addition to standard patient monitors such as continuous invasive blood pressure, central venous pressures, cardiac output, and ventilator management, the NCCU employs highly specialized neurological monitors. These monitors collect information on intracranial pressure (ICP), cerebral blood flow (CBF), cerebral spinal fluid (CSF) analysis, brain tissue oxygen monitoring, and electrophysiology. As with any type of biological monitoring, interpretation relies not only on knowledge of normal and pathological signals but also on the presence of artifacts. Deviation in measurements obtained by technological support supplied by a monitor may represent neither normal neurophysiology nor pathology. This chapter will discuss several types of invasive and noninvasive techniques for NCCU neurophysiologic monitoring, with relevant examples of common and rare artifacts.
INTRACRANIAL PRESSURE
ICP monitoring is pertinent for a variety of diseases, including malignant edema in large territorial ischemic strokes, mass effect from hemorrhagic strokes and tumors, and global cerebral edema from hypoxic brain injury, severe hepatic encephalopathy, and traumatic brain injury.
According to the Monro–Kellie doctrine, there is a fixed volume of intracranial space that is occupied by the brain, CSF, and blood. When a new component enters this space, one of these must be sacrificed (usually CSF first, then brain or blood) (1). Because cerebral perfusion pressure (CPP) is the difference between mean arterial pressure (MAP) and ICP, as the ICP increases, the CPP decreases, further limiting blood flow to the brain and subsequently causing secondary ischemia and edema, which in turn further increases ICP.
Depending on the underlying disease, availability, comorbidities, and goals of monitoring, there are many choices for ICP monitoring.
Invasive ICP Monitoring
There are several options available for invasive ICP monitoring. These are placed by neurosurgeons, or experienced neurocritical care physicians (Figure 4.1).
External Ventricular Drainage
The external ventricular drain (EVD) is the gold standard for ICP monitoring. An added benefit to ICP monitoring is the ability to drain CSF to therapeutically relieve ICP. A catheter is typically placed into the right lateral ventricle in the brain, and is connected to sterile tubing that meets a three-way stopcock on the collection apparatus. The stopcock allows the system to either be opened to the collection bag or opened to the electronic transducer, which reports the ICP and waveform via continuous electronic display. The ICP and waveforms generated can also be deduced by the features of the CSF that are present in the tubing between the stopcock and the collection bag. Before the tubing drains into the bag, it runs vertically along a graduated scale for measurement. The level where the CSF equilibrates along the scale represents the tidal ICP. One can also appreciate the rhythmic variations in CSF level, which correspond to the waveform.
52
FIGURE 4.1: (A) Types and placement of invasive ICP monitors. These include intraparenchymal, subarachnoid, intraventricular, and epidural. EVD is considered the gold standard. (B) Typical setup and positioning of a patient with an EVD (note the patient does not need to remain in the lateral decubitus position after insertion, as along as the stopcock is leveled at the tragus).
CSF, cerebral spinal fluid; EVD, external ventricular drain; ICP, intracranial pressure.
Normal CSF waveforms have three peaks (Figure 4.2). The percussion wave (P1) represents the arterial pulsation transmitting through the choroid plexus into the ventricle. The tidal wave (P2) reflects the venous compartment, translating into the compliance of the ventricular system. The P2 wave should be 80% of the height of P1. Finally, the dicrotic wave (P3) represents the aortic valve closure.
Normally, the P1 is the highest point, followed by P2 and P3 (2). Peaked P2 waves could be a sign of poor ventricular compliance due to increased ICP. Alternatively, this pattern can be seen in jugular venous compression (which can occur during central line placement or incorrect head or neck positioning), the Valsalva maneuver (in an incompletely sedated patient who is agitated, or in a constipated patient who is straining to have a bowel movement), or in an underventilated patient with carbon dioxide 53accumulation. Broad-based, low-amplitude waveforms with indistinct P waves should raise the concern of drainage occlusion either from cellular debris, blood clots, tissue fragments, or as an artifact from kinking of the catheter (3). However, a flat line may mean severe pathology such as the absence of CBF and brain death. The EVD only transduces a wave form if it is not draining into a collection bag. Therefore, if a flat line is seen on the monitor, check to make sure the EVD is draining properly. If it is not draining, stop drainage and reassess the monitor. Finally, sharp, rapid wave forms that are asynchronous with heart rate imply a movement artifact of the EVD system or the monitor (Figure 4.2A–E).
FIGURE 4.2: (A) Normal wave forms with P1 (arterial pulsation), P2 (venous compartment), and P3 (aortic valve closure) waves. (B) Peaked P2 waves, which can symbolize jugular compression artifact or Valsalva physiology. (C) Broad-based, low-amplitude waves, which suggest drainage occlusion artifact. (D) A flat line represents a severe occlusion artifact, or that the 3-way stopcock is closed to the transducer and is draining CSF. (E) Fast, sharp waveforms which do not correlate with respiration or heart rate suggest movement of the patient or transducer system.
CSF, cerebral spinal fluid; ICP, intracranial pressure.
Transduced and tidal ICPs that are inconsistent with the patient’s clinical situation (normal or low ICP in a patient with hydrocephalus, or high ICPs in a patient who is awake, neurologically intact, and without headache) should raise the question of incorrect transducer positioning. The transducer should be leveled at the tragus of the ear, which represents the foramen of Monroe. If the transducer is too high, the ICPs will be falsely low, and vice versa.
Noninvasive Monitoring
Optic Nerve Sheath Diameter
The pia and arachnoid meningeal layers surround the optic nerve (ON). When the ICP increases, we can noninvasively observe the effects on the ON (4). The ON is typically 3 mm in diameter, and is surrounded by 0.1 to 0.2 mm of subarachnoid space, and a 0.3-mm-thick optic nerve sheath (ONS) (5,6). An ONS diameter of less than 5 mm is considered normal, but the sensitivity, specificity, and reproducibility are variable (6–9).
Measurements of the ONS diameter are obtained 3 mm posterior to the back wall of the globe, in the axial plane of the ON (5,10). The anatomical structures of the ON complex should be clearly identified, including retina; lamina cribrosa (hyperechoic); the ON fibers (hypoechoic); the subarachnoid space (hyperechoic); the ONS (hypoechoic); and the central retinal artery, which can be identified (Figure 4.3) using Doppler ultrasonography (11). The lamina cribrosa is a dense fiber layer that is hyperechoic and can cast a shadow artifact, mimicking the hypoechoic ON (Figure 4.4). The central retinal artery runs through the center of the ON and will show the true direction of the ON despite lamina cribrosa artifact.
Time may be a further source of artifact as it has been suggested that there is a delayed reversal of nerve sheath distension, especially in patients with rapidly fluctuating ICP (commonly seen in patients with poor compliance) (12).
FIGURE 4.3: (A) Normal ON anatomy. (B) Labeled figure with retina, lamina cribrosa, ONS, ON, and subarachnoid space (CSF).
CSF, cerebral spinal fluid; ON, optic nerve; ONS, optic nerve sheath.
Transcranial Doppler Ultrasound
Transcranial Doppler ultrasound (TCD) uses low-frequency Doppler over four thin acoustic windows: transtemporal, suboccipital, transorbital, and submandibular (13). TCDs can be useful in identifying increased ICP, in addition to cerebral artery vasospasm (VSP), a condition that commonly occurs after aneurysmal subarachnoid hemorrhage (aSAH). When the intracranial vessels are isonated through these bone windows, the peak systolic velocity (PSV) and end diastolic velocity (EDV) can be directly measured (Figure 4.5). From these measurements, a variety of other indices can be calculated, including mean flow velocity (MFV), the Gosling pulsatility index (PI), the Pourcelot resistivity index (RI), and the Lindegaard ratio (LR) (13–16).
The most common source of artifact is poor bone windows, which occur in 10% to 15% of patients (6) and are due to thickness of the cancellous component of the temporal bone (17). The thickness prevents efficient transduction of ultrasound waves, decreasing the clarity of the cerebral vasculature (Figure 4.6). Poor bone windows are more common in the elderly and women (18) and can cause significant error in velocity interpretation (19).
54
FIGURE 4.4: (A) The hypoechoic streak behind the globe appears to be the ON. (B) The central retinal artery tracks medially, revealing the true route of the ON and that the hypoechoic streak is a shadow artifact from the lamina cribrosa.
ON, optic nerve.
Another well-described ultrasound artifact is known as a twinkling artifact. A twinkling artifact is manifested by a rapidly changing mixture of red and blue Doppler signals, appearing as rapid changes in flow direction in relation to the TCD probe (20). Twinkling artifacts have been seen next to strongly reflecting structures such as parenchymal calcifications and cerebral aneurysm clips and coils (20,21). It is important to be aware of and recognize this artifact as aSAH patients typically have aneurysms secured by one of these devices, and many undergo routine TCD monitoring for VSP.
TCD is user dependent and only experienced ultrasonographers should perform the test for clinical assessment. A steady hand is required to both find and maintain arterial pulsations and appropriate wave forms. Even small, abrupt movements of the probe can cause small, abrupt changes in isonation, which may appear and sound like the short bursts of noise heard when microemboli travel through the arteries. An unsteady hand may create false-positive signs for cerebral microemboli, and should be avoided.
FIGURE 4.5: Normal TCD waveform of the left proximal MCA. The PSV (arrow) = 107.2 cm/s. EDV (arrow head) = 53.6 cm/s.
EDV, end diastolic velocity; MCA, middle cerebral artery; PSV, peak systolic velocity; TCD, transcranial Doppler ultrasound.
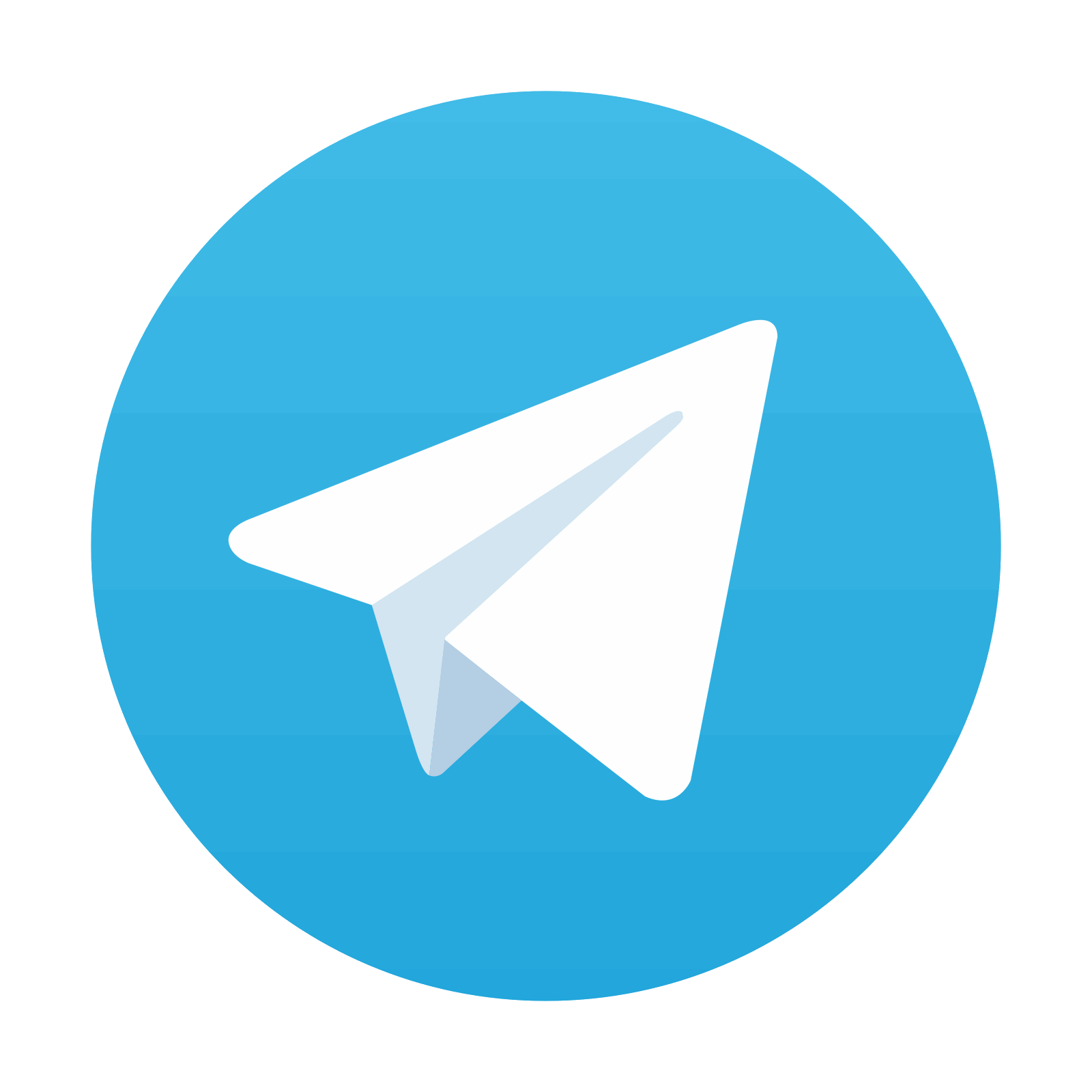
Stay updated, free articles. Join our Telegram channel

Full access? Get Clinical Tree
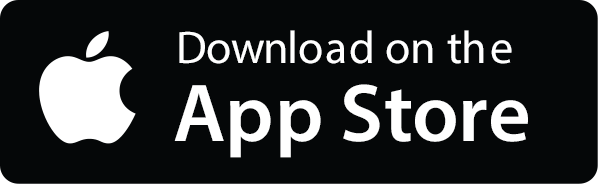
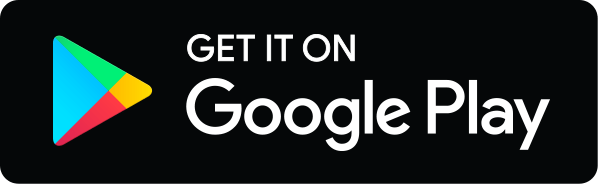