Artificial Disk Surgery of the Cervical Spine: Biomechanics of Cervical Disk Prostheses
Juan M. Raposo
Bruce V. Darden II
BACKGROUND
Anterior arthrodesis for the treatment of cervical radiculopathy and myelopathy is one of the most successful procedures performed in the field of spine surgery today. Despite this exemplary track record, several long-term clinical studies have reported high rates of postoperative adjacent level disk degeneration that often requires additional surgical treatment (1,2). While the exact mechanism for this transitional deterioration remains uncertain, one theory supported by numerous biomechanical investigations suggests that abnormal kinematics and mechanical forces on adjacent levels are the result of nonphysiologic restraint of the prior mobile spine segment (3,4). This derangement may hasten the degenerative process and be partly responsible for this observed clinical phenomenon.
The biomechanical theory for adjacent level degeneration following anterior arthrodesis of the cervical spine has thus fueled enthusiasm for motion-preserving technologies such as cervical disk arthroplasty. This chapter reviews the relevant anatomic and kinematic features of the cervical spine, discusses biomechanical considerations in implant design, and summarizes pertinent basic science research and clinical studies that relate to biomechanical function of these prostheses and their effect on the cervical spine.
RELEVANT ANATOMY AND KINEMATICS OF THE NATIVE CERVICAL SPINE
The goal of cervical disk arthroplasty is to reproduce quasi-physiologic kinematic and mechanical properties of a cervical motion segment while maintaining the inherent stability of the osseoligamentous architecture, which is critical to protecting nearby neural elements. Due to differing regional anatomic characteristics and mechanical functions, the cervical spine is commonly divided into the atlantoaxial (C1-C2) and subaxial cervical spine (C3-C7). Since current motion-preserving implants are designed for use solely in the mid and lower cervical spine, this discussion is limited to the biomechanical principles applicable to the subaxial region of the cervical spine.
Cervical kinematics are influenced by the complex interplay occurring among three distinct articulations (the disk anteriorly and two facets posteriorly) at each motion segment. This anatomic arrangement allows for motion to occur in multiple directions (flexion, extension, translation, right/left lateral bending, and right/left axial rotation) at each level. Due to the inclination of the facet joints, oriented approximately 45 degrees from the horizontal plane, the dominant pattern of motion in the subaxial cervical spine is in the sagittal plane. Approximately 60% of all cervical flexion and extension occurs from C3 to C7, with normal physiologic range of motion varying from 14 to 22 degrees for each corresponding segment (5, 6, 7, 8 and 9).
Flexion and extension moments are accompanied by concomitant translational movements between adjacent vertebra, forming coupled motions. With increasing degrees of forward flexion, unshingling of the overlapping posterior articular facets ensues, allowing for anterior translation of the cephalad vertebrae relative to its caudal counterpart. Conversely, with extension moments, posterior translation will occur. Coupled motion also occurs with lateral bending moments, which are associated with axial rotation. Such coupled motions result in slight migration of the center of rotation throughout the normal range of motion.
Spondylitic changes in the cervical spine impart notable changes on the kinematic and mechanical properties of the affected motion segment. The intervertebral disk undergoes dehydration and fissuring, causing a decrease in its elastic properties and overall disk height. As a result, the uncovertebral joints bear increased load demands and form osteophytes. The altered stress distribution also
creates increased demands on the facet joints and joint capsules leading to subtle derangements in the couple motion patterns. Ultimately, this degenerative cascade will adversely affect range of motion, which may be further hampered by pain inhibition caused by the nociceptive response to the diseased segment.
creates increased demands on the facet joints and joint capsules leading to subtle derangements in the couple motion patterns. Ultimately, this degenerative cascade will adversely affect range of motion, which may be further hampered by pain inhibition caused by the nociceptive response to the diseased segment.
IMPLANT DESIGN CONSIDERATIONS AND BIOMECHANICAL RATIONALE
For a joint-replacing implant to be clinically successful, it must be (a) safe and easy to use, (b) durable without significant, deleterious short- or long-term effects to the patient, (c) effective at treating the underlying clinical disorder, while providing long-term pain relief, and (d) maintain or enhance existing motion. Previously successful endeavors in joint motion-preserving technologies have been predicated on a thorough understanding of normal joint kinematics, sound biomechanical design rationale, and rigorous in vitro testing and analysis for implant design and function. This section focuses on critical kinematic principles and mechanical factors that present special challenges in the design and development of these implants.
CENTER OF ROTATION
The center of rotation is not a fixed point. Rather, the instantaneous axes of rotation for a cervical motion segment through a physiologic range of motion are within 1 to 2 mm of each other for a given motion segment. Penning first calculated centers of rotation for cervical motion segments based on measurements made from flexion and extension radiographs (7). His findings were later refined by more sophisticated experimental designs, which established normalized instantaneous centers of rotation (ICR) for each cervical motion segment in a sample patient population, including a subset of individuals with spondylosis (9). The center of rotation for the middle and lower cervical spine can be approximated to be just posterior to the midaxis of the caudal end plate (superior end plate of the lower cervical vertebrae) for a given motion segment (Fig. 94.1A,B).
While the ICR can be grossly approximated to be just posterior and inferior to the center of the caudal vertebral end plate in the lower cervical spine (C5 to C7), this rule is less applicable to the upper cervical motion segments at C3-C4 and C4-C5 (Fig. 94.1A). At these levels, the mean instantaneous axis of rotation will deviate more posteriorly and caudal relative to the disk space. The reason for this variability lies in the differing morphologic characteristics of the facets in the upper and lower cervical spine. As classically described by Lysell (10), the segmental arc of motion in the upper segments where the facets are oriented more horizontally results in a flatter arc of motion, whereas in the lower segments, owing to the more vertical nature of the facets, the motion arc is sharper (Fig. 94.2). Consequently, the larger radius of curvature in the upper region places the ICR farther down than in the lower region, where the ICR is closer to the moving vertebra (11). The varying inclination of the facet joints also creates regional differences in the coupled motion patterns observed within the cervical spine, characterized by a 2:3 ratio in degrees of axial rotation to lateral bending (0.67) seen at C2-C3 compared to a ratio of 1:7.5 (0.13) at C6-C7 (10). These interregional differences in cervical spine kinematics add further complexity to the task of optimal disk arthroplasty design and implantation.
Restoring the center of rotation is vital to maintaining proper joint kinematics. In a recent long-term follow-up series of patients implanted with a mobile bearing designed lumbar artificial disk, 11% of patients experienced facet pain-related complications related to abnormal displacement of the center of rotation, defined as greater than or equal to 4 mm from the center of the vertebral body (12). While obvious differences exist between the cervical and lumbar spine in terms of physiologic load transmission and stress distribution, this finding nonetheless underscores the importance of properly restoring the center of rotation within the anterior column so as not to disturb the mechanical functions of the intact posterior facets in a functional spine unit consisting of three separate articulations.
With regard to implant designs, prostheses with ball-and-socket surface geometries will have a predetermined center of rotation at the center of the conceptual sphere formed from the convex surface (Fig. 94.3). This feature has important clinical implications, as greater precision in the placement of ball-and-socket implants is necessary in order to replicate the physiologic center of rotation and minimize strain on the facet joints and posterior ligamentous structures.
CONSTRAINT
With respect to cervical disk replacement where the dominant plane of motion is sagittal, constraint may be defined as the limitation of pure anteroposterior or lateral translational intervertebral motion (13). An unconstrained implant would allow for unrestricted coupled motions to occur, whereas a fully constrained device would solely permit uniplanar rotation (e.g., flexion and extension) without any translation. Compared to implant designs for peripheral joints such as the knee or elbow where there is a wide variance from the most to the least constrained device, the difference among total cervical disk replacements currently available in the market is rather modest (Table 94.1).
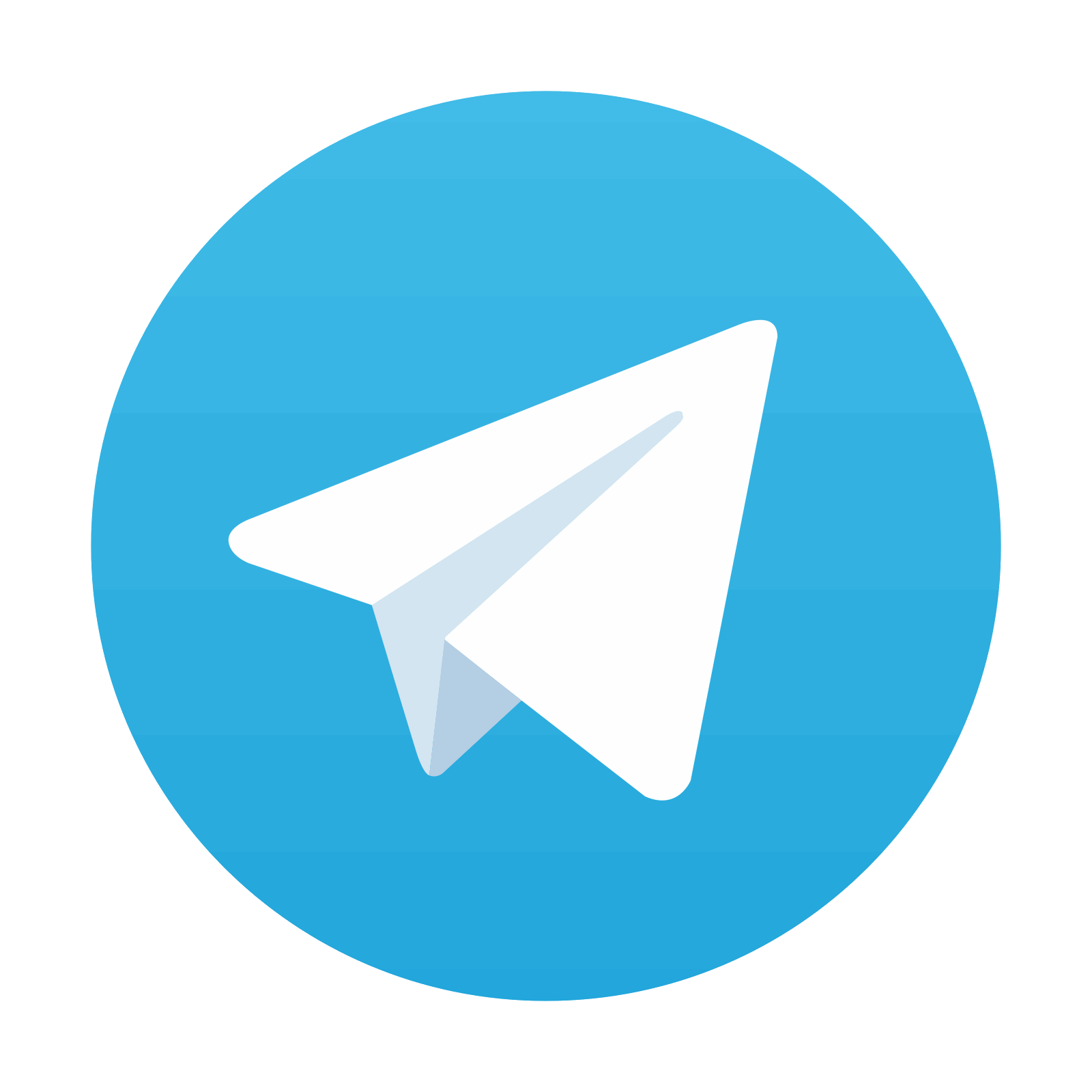
Stay updated, free articles. Join our Telegram channel

Full access? Get Clinical Tree
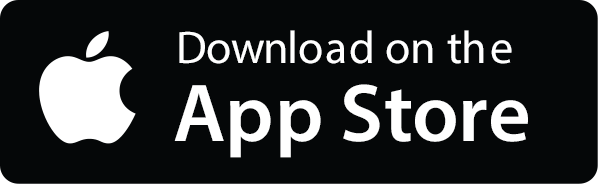
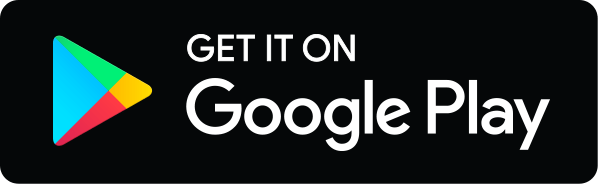