Artificial Disk Surgery of the Cervical Spine: Material and Design Characteristics
Wellington K. Hsu
Paul A. Anderson
The goal of spinal arthroplasty is to reconstruct the functional spinal unit with a motion-sparing prosthesis following discectomy for treatment of radiculopathy and myelopathy. Both surgeons and patients have an intuitive understanding that the maintenance of motion is theoretically better than fusion in accordance with the fundamental principle of Hippocrates’ teaching that “motion is life.” However, the scientific basis for this justification remains controversial.
Although ventral cervical fusion is a well-recognized operation with a high degree of patient satisfaction and excellent outcomes, cervical total disk arthroplasty (TDA) offers an alternative treatment that aims to maintain motion following discectomy. For single-level conditions, about 90% of patients experience clinically significant improvement after fusion (1); however, complications such as pseudarthrosis exist despite the use of various bone graft alternatives and internal fixation. Furthermore, there is strong clinical evidence that degeneration occurs at spinal segments adjacent to fused levels in the cervical spine, but its exact cause, whether related to the biomechanical effect of fusion or natural history of the condition, is currently controversial. The primary rationale for cervical disk arthroplasty is to prevent this complication, commonly referred to as “adjacent segment degeneration.”
Cervical disk arthroplasty maintains physiologic motion, restores disk height, and some viscoelastic properties of the spinal segment. Randomized controlled trials have also demonstrated that artificial disk implants decrease the morbidity of fusion and allow earlier return to function. Several distinctions from lumbar disk arthroplasty are important. Cervical disk arthroplasty is indicated as a reconstruction after neurologic decompression, whereas lumbar disk arthroplasty is performed for the treatment of chronic low back pain. Direct neural decompression is more likely to lead to improved patient outcomes, making results of cervical arthroplasty better than those in the lumbar spine. Furthermore, the decreased loads in the cervical spine allow different materials to be used that can be magnetic resonance imaging (MRI) compatible for future evaluation. Finally, despite the possibility of greater ramifications from neurologic injury, the ventral cervical spine allows not only for a technically easier approach but also revision surgery with less life-threatening complications.
Rigorous mechanical, animal, and clinical testing of multiple artificial disk implants has led to the FDA approval of three devices to date. Furthermore, at least five other devices are currently in clinical trials in the United States and many more are currently in use worldwide. Although clinical trials studying the outcomes of cervical disk arthroplasty are in its infancy, preliminary evidence suggests excellent early outcomes with the potential to reduce the number of secondary operations (2).
By their nature, cervical arthroplasties have bearing surfaces subject to wear. The science behind the wear patterns of prostheses may be unfamiliar to spine surgeons but essential to understand when implanting such devices. Additionally, the material and design characteristics of individual implants are important to consider when predicting the long-term impact of artificial disk implants on spinal biomechanics and the critical surrounding structures. The purpose of this chapter is to review the important design concepts, material characteristics, and wear-debris patterns of available cervical arthroplasty implants.
DESIGN CONSIDERATIONS
Since the first concepts of total joint arthroplasty introduced by Sir John Charnley in 1960, the principles behind implant design have been studied in many joints throughout the human body including the hip, knee, shoulder, ankle, and elbow. In each anatomic area, the concepts remain similar: provide a safe, stable, durable, and biomechanically sound artificial joint that allows for the resection of degenerative bearing surfaces that serve as significant
pain generators. Modern arthroplasty techniques result in some of the most satisfied patients following any surgery.
pain generators. Modern arthroplasty techniques result in some of the most satisfied patients following any surgery.
However, unlike appendicular arthroplasty, TDA in the cervical spine presents a number of unique considerations. Because bone stock in the cervical spine is significantly limited, there is less flexibility in bone removal and preparation to accommodate a prosthesis. Moreover, in contrast to the knee and hip joint, the intervertebral disk serves as a significant contributor to spinal stability by restoring balance to the anterior and middle columns in a three-column spine model. The complete resection of this disk material can have a destabilizing effect that must be accounted for by any implant.
Furthermore, cervical disk arthroplasty must account for the remaining structural components of the spinal segment. In general, the stability of the prosthesis design requires a relatively intact ligament structure including the dorsal osteoligamentous complex, the lateral masses and facet articulations, and lateral annulus. Some prostheses may also require intact uncovertebral joints to provide stability in the coronal plane or posterior longitudinal ligament (3,4). Finally, the disk prosthesis must function in conjunction with the dorsal facet articulations to allow proper motion.
Similar to the knee joint, the functional spinal unit has coupled motion where angular and translation movements can be simultaneous along multiple axes. In the intact cervical spine, this characteristic affects the center of rotation that is just inferior to the disk space and slightly dorsal from the midline in the flexion-extension motion plane. Because of coupled translation and angulation, the center of rotation is mobile during normal physiologic range of motion.
Two basic designs to preserve physiologic range of motion in the cervical spine are available: fixed or singleaxis of motion and variable axis of motion. The variable axis devices allow coupled translation with rotation in during motion. Examples to accomplish this are a mobile bearing and a ball-and-trough design such as that seen in the Prestige ST Cervical Disc System (Medtronic Sofamor Danek, Inc., Minneapolis, MN) (Fig. 93.1). These designs allow for the center of rotation to move dorsally during extension of the spinal unit. Each implant is different in how much coupled angulation and translation it allows. The single-axis motion prostheses are ball-and-socket designs where no coupled translation is allowed (ProDisc-C Cervical Implant, Synthes, Inc., West Chester, PA) (Fig. 93.2). These devices provide greater inherent stability by resisting excessive motion and preventing translation during rotation. However, the normal sliding motion of the facet joints in flexion and extension cannot occur with fixed axis devices. All current devices available are unconstrained in the physiologic range of motion (neutral zone) in the cervical spine; that is, they provide little or no restraint to motion. At the extreme ends of motion, the devices experience edge contact, which can lead to abnormal wear patterns. While it is currently unknown what the long-term clinical implications of a fixed versus mobile center of rotation from an artificial disk are, a number of arthroplasty implants attempt to replicate this normal condition.
The intervertebral disk functions as a viscoelastic tissue transferring loads and controlling motion. Because this property has important implications in the transfer of stresses to adjacent spinal segments, two studies have examined the ability of prostheses to absorb vibrational and impact loads by measuring differences at adjacent segments (5,6). Dahl et al. (5) applied vibrational stress and a single impact load on energy absorption. He compared the intact spine, a compliant cervical prosthesis, and fusion. He found no difference between the intact spine and the
prosthesis. However, fusion significantly increased disk pressures at the adjacent segments during loading. In the prostheses tested, the energy absorbed at the treated level was dissipated through motion of the arthroplasty devices rather than absorbed by deformation. LeHuec et al. (6), in a similar study design, compared a metal-on-metal to a metal-on-plastic lumbar device. They found no difference in intervertebral disk pressures at adjacent segments between either device compared to intact, indicating that the difference in viscoelastic properties of the prostheses were minimal as long as motion was maintained. Although design attempts to maintain viscoelastic properties of prostheses exist, it does not appear to have substantial value as long as motion is restored. Therefore, using materials that can perform under physiologic loading may not be important, especially considering that these materials tend to have poorer durability and wear resistance.
prosthesis. However, fusion significantly increased disk pressures at the adjacent segments during loading. In the prostheses tested, the energy absorbed at the treated level was dissipated through motion of the arthroplasty devices rather than absorbed by deformation. LeHuec et al. (6), in a similar study design, compared a metal-on-metal to a metal-on-plastic lumbar device. They found no difference in intervertebral disk pressures at adjacent segments between either device compared to intact, indicating that the difference in viscoelastic properties of the prostheses were minimal as long as motion was maintained. Although design attempts to maintain viscoelastic properties of prostheses exist, it does not appear to have substantial value as long as motion is restored. Therefore, using materials that can perform under physiologic loading may not be important, especially considering that these materials tend to have poorer durability and wear resistance.
BIOMATERIALS
Choices in the material used in cervical disk arthroplasty are critical to long-term performance and are similar to those that have been studied extensively in joint arthroplasty. The important material considerations are durability, incidence of fatigue or fracture, and wear characteristics. Furthermore, one must consider properties such as stiffness, biocompatibility, and resistance to corrosion. Unique to the cervical spine, the choice of MRI-compatible materials to allow for follow-up evaluation of the neural elements is also relevant.
Stainless steel is the most inexpensive and widely available material but has mechanical properties inferior to that of other metals. Steel also has greater ductility but less biocompatibility than comparative metals. Steels have a long track record in a variety of orthopaedic implants in different anatomic sites. Consistent imaging artifacts with both CT and MRI make long-term evaluation difficult (7). A number of alloys have been developed that contain iron, carbon, chromium, nickel, and molybdenum to improve upon the material properties of steel alone. The Prestige ST implant, which is currently FDA-approved, is constructed of articulating stainless steel components.
Cobalt-chromium alloy contains molybdenum, which decreases the grain size of alloy and leads to improved mechanical properties. Of the most common metals used in joint arthroplasty, cobalt-chromium alloys have the highest Young’s modulus and hardness (Table 93.1). Other additives are common, such as nickel and chromium oxide, on the surface to resist corrosion. Wear properties of cobalt-chromium bearing surfaces have been studied extensively in both knee and hip arthroplasties with excellent long-term wear profiles. However, MRI quality in the presence of cobalt-chrome is significantly deteriorated in comparison to titanium alloys (7). The ProDisc-C and Porous-Coated Motion (PCM) device (Cervitech, Rockaway, NJ) implants have cobalt-chrome components.
TABLE 93.1 Properties of Materials Used in Prosthetic Cervical Disksa | |||||||||||||||||||||||||||||||||||||||||||||||||
---|---|---|---|---|---|---|---|---|---|---|---|---|---|---|---|---|---|---|---|---|---|---|---|---|---|---|---|---|---|---|---|---|---|---|---|---|---|---|---|---|---|---|---|---|---|---|---|---|---|
|
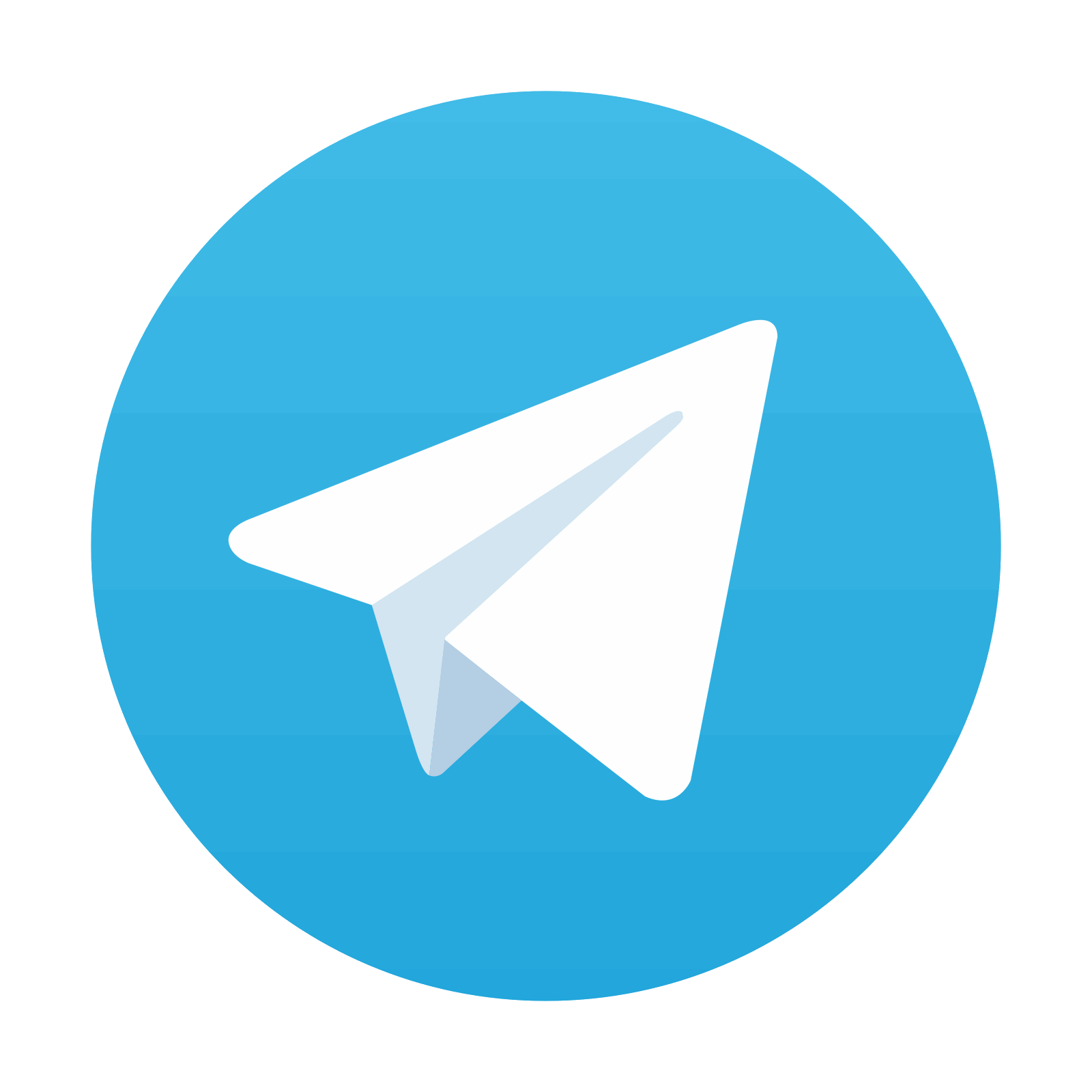
Stay updated, free articles. Join our Telegram channel

Full access? Get Clinical Tree
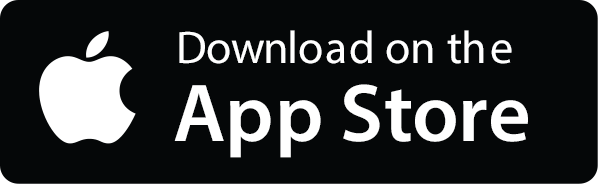
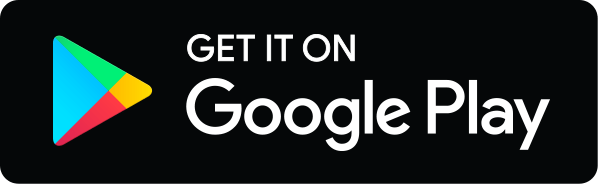