Atlantoaxial Stabilization: C1-C2 Instrumentation Techniques
Christopher A. Iannotti
Langston T. Holly
The treatment of cervical spine instability has undergone major advances in recent decades. In 1891, the era of internal spinal fixation was born when Hadra (1) used a wire to secure adjacent cervical vertebrae, which were unstable secondary to trauma and Pott’s disease. This represented a major advance in the treatment of spinal instability through the use of internally applied, nonbiologic materials to achieve stability prior to fusion. The first successful treatment of atlantoaxial instability was performed by Mixter and Osgood (2) in 1910. In this procedure, the posterior arch of the atlas was fixed to the spinous process of the axis with a silk thread.
Atlantoaxial instability has historically been treated using various dorsal C1-C2 autologous graft and wiring techniques. Three dorsal wiring techniques are commonly used—the Gallie, Brooks, and Sonntag techniques. The Gallie technique for dorsal graft/wire fusion was described in 1939 and involved placing an onlay graft from the C1 ring to the C2 lamina, securing it with a midline wire. In 1976, Fielding et al. (3) reported results of 46 Gallie-type fusions for atlantoaxial instability. The fusion rate after 2 weeks of postoperative bracing, traction, or halo treatment was 93%. In 1978, Brooks and Jenkins (4) introduced a wedge compression technique for atlantoaxial arthrodesis. Their technique involved the placement of bilateral rectangular interposition grafts between C1 and C2. The grafts were secured and placed under compression by using dual bilateral wires. All patients were treated with postsurgical rigid orthosis, and a 96% fusion rate was achieved in 30 patients. In 1991, the Dickman et al. (5) fusion technique was introduced. This technique involves a single interposition graft placed between the C1 and C2 laminae, with compression applied to the graft by the use of a looped wire. All patients underwent halo immobilization for 12 weeks after surgery, followed by 4 to 6 weeks of treatment with a Philadelphia collar—resulting in a 97% fusion rate.
Dorsal wire stabilization and structural bone grafting techniques do not provide sufficient immobilization of the atlantoaxial complex, resulting in nonunion rates up to 30% even with adjunctive halo immobilization. In addition, the use of sublaminar wires or cables carries the risk of spinal cord injury, especially in the case of a nonreduced C1-C2 subluxation. Recently, more refined methods of C1-C2 fixation have been developed using screws, screwplate, and screw-rod systems. The transarticular screw fixation technique was first described by Magerl and Seeman (6) in 1987 and resulted in significant improvements in fusion rates. This technique requires reduction of C1 on C2 before screw placement. Achieving proper placement of the screws and avoiding injury to the vertebral artery are technically demanding. Approximately 20% of patients requiring atlantoaxial fusion show anatomic variations in the course of the vertebral artery or in bony anatomy on at least one side, precluding screw placement. To maximize stability, transarticular screw fixation is combined with a Gallie, Brooks, or Sonntag fusion. This increases the risk of neural injury caused by the passage of wires or cables into the spinal canal. In addition, this procedure requires the use of structural bone grafting as well as an intact arch of C1. A different approach to achieve posterior atlantoaxial stabilization has been described using segmental fixation of C1 and C2 with C1 lateral mass screws and C2 pedicle screws. The so-called C2 pedicle screws are placed through the pars interarticularis and into the C2 pedicle. This technique is associated with high fusion rates in the 95% to 98% range, is biomechanically equivalent to transarticular screw placement, and carries a decreased risk of injury to the vertebral artery.
Atlantoaxial instability may be defined in terms of the atlantodental interval (ADI), with an ADI of greater than 5 mm in adults generally accepted as radiographic evidence of an unstable C1-C2 articulation. An unstable atlantoaxial motion segment may result from traumatic, congenital, neoplastic, degenerative, infectious, or iatrogenic etiologies. Indications for surgical fusion for atlantoaxial instability include (a) atlantoaxial subluxation secondary to transverse ligament rupture or laxity caused by trauma, rheumatoid arthritis, or infection; (b) type II odontoid fractures with greater than 6 mm of displacement; (c) chronic nonunion or failure of conservative treatment of odontoid fractures; (d) symptomatic os
odontoidium or odontoid hypoplasia/aplasia; (e) rotatory subluxation; (f) instability after transoral odontoidectomy; and (g) destruction of the dens by tumors. The common end result of each of these pathologic processes is narrowing of the spinal canal resulting from intermittent chronic neural compression or impingement of neural elements, or damage to structural elements resulting in neurologic deterioration, deformity, and/or pain.
odontoidium or odontoid hypoplasia/aplasia; (e) rotatory subluxation; (f) instability after transoral odontoidectomy; and (g) destruction of the dens by tumors. The common end result of each of these pathologic processes is narrowing of the spinal canal resulting from intermittent chronic neural compression or impingement of neural elements, or damage to structural elements resulting in neurologic deterioration, deformity, and/or pain.
In cases of atlantoaxial cervical instability, the primary goal of all the procedures described in this chapter is to restore and preserve alignment, decompress the neural elements, resist forces in all axes of movement, and provide the optimal environment for bone fusion to occur. Nonsurgical management of atlantoaxial instability is mainly in the form of halo bracing. However, long-term halo bracing is not well tolerated by most patients, is associated with significant segmental movement at C1-C2, and results in relatively low fusion rates when used alone. In order to facilitate higher rates of fusion and allow for rehabilitation, internal fixation using a variety of instrumentation methods is generally used. Over the last several decades, anterior and dorsal techniques for stabilization of the C1-C2 segments have been developed. Most surgeons routinely use one of several common dorsal stabilization options— sublaminar wiring techniques, interlaminar clamps, and C1-C2 instrumentation (transarticular screw or segmental fixation). However, in the absence of adequate bone fusion, all instrumentation constructs ultimately fail. This chapter reviews the surgical indications and contraindications, operative techniques, and advantages and disadvantages of these commonly used posterior C1-C2 techniques.
HALO BRACING
The cranial halo device was first introduced by Perry and Nickel (7) in 1959 for the management of severe cervical instability secondary to poliomyelitis. In 1968, Nickel introduced the halo ring for use as a traction device. Cranial halo traction devices have supplanted Gardner-Wells tongs in many centers treating spinal disorders, as most halo rings that are part of a halo vest system can be used as traction devices. If the spine surgeon plans to maintain the patient in a halo vest (as the primary method of fixation or after a surgical stabilization procedure), the initial use of halo traction will obviate the need to change from Gardner-Wells tongs to cranial halo ring during assembly of the halo vest system. Cranial halo traction provides four-point rigid skeletal fixation through a circumferential steel ring and, when attached to a body jacket with metal uprights, allows for early mobilization. Specific indications for application of halo vest include C1 burst fracture (Jefferson fracture), C2 hangman’s fracture, and facet dislocations.
INDICATIONS
Halo traction can be used to stabilize fractures as well as reduce fracture dislocations and dislocations of the upper and lower cervical spine in the setting of trauma, degenerative disease, infection, inflammation, rheumatologic disorders, and tumor. Halo vest immobilization may also be used postoperatively to facilitate fusion following various C1-C2 instrumentation techniques (e.g., interspinous graft and wiring). The halo vest provides good stabilization of the upper cervical spine (C1 and C2), as upper cervical spine movement has been reported in the range of 4% to 30% in patients wearing halo vests. However, purely ligamentous injuries do not heal well with a halo vest. Halo vests do not immobilize the subaxial cervical spine as well as the upper cervical area.
HALO PLACEMENT
There are a variety of halo vest devices available on the market, and the spine surgeon should become familiar with several of these. When used as cervical traction, the halo ring is placed with the anticipation that it will be eventually secured to a jacket/vest to allow early mobilization. The patient may be positioned either supine or, if not contraindicated, upright. Halo rings are available in various sizes, and a halo to skull clearance of 1.0 to 1.5 cm should be obtained. The hair is clipped at the planned pin sites and the skin is prepared with Betadine and infiltrated with local anesthetic. Four proposed pin sites are chosen. The ventral pin sites are located approximately 1 cm above the lateral third of the eyebrow, avoiding injury to the supraorbital nerve. The dorsal pins are placed in a channel diagonally opposite the ventral pins and situated approximately 1.5 cm above the ears. Before the halo ring is placed, it is held in place by an assistant. Current systems have suction-tipped pins to hold the halo in position until the skull pins are placed, thus permitting one person to place the halo safely. Hexagonal lock nuts are loosely placed on each pin outside the ring prior to advancing the pin. The pins should be placed so that they are perpendicular to the skull. The skull pins are then gently advanced until the outer layer of dermis is penetrated. The ventral and diagonally opposed dorsal pins are simultaneously tightened by hand, then are tightened to a maximum of 6 kg using the torque screwdriver. Alternate tightening of opposed skulls pins prevents asymmetric displacement of the halo ring. The pins should penetrate only the outer table of the skull. Once the pins are properly placed, the hexagonal nut is tightened against the halo ring, thus securing the position of the pins and preventing their backing out. Twenty-four hours later, the hexagonal nuts should be loosened and the skull pins tightened to 6 kg of pressure with the torque screwdriver. The hexagonal nuts are then retightened, and no further tightening of the pins is necessary as this risks inadvertent penetration of the inner table of the skull. A cervical spine radiograph should be obtained to check spinal alignment. The halo ring may be used to provide cervical traction if needed and may be converted at an appropriate time to a halo vest device.
COMPLICATIONS
Most complications associated with the use of both cranial tongs and halo ring devices are related to the skull pins. With proper initial placement of the halo ring and pins, and meticulous daily care of pin sites, most of the risks can be prevented. Potential risks include penetration of the inner table of the skull with resultant intracranial hemorrhage, brain injury, meningitis, or brain abscess.
Other complications include pin site infection (soft tissue or osteomyelitis), nerve injury, frontal scarring, pin site loosening, and pin site bleeding. Infection at the pin site may present as local pain, drainage, or as a loose skull pin. For a pin site infection, the skull pin should be removed and a new sterile pin should be placed at a new site. Soft tissue infections usually respond to local care and antibiotics, while osteomyelitis may require debridement. Placement of the pins in the fronto-orbital crest avoids risk of injury to the supraorbital and supratrochlear nerves located more medially. Pin loosening has been reported in 36% to 60% of patients, is usually associated with pain in the absence of infection, and may be due to a fall or blunt trauma. If cranial traction is used with a halo ring device, frequent neurologic examination should be performed, along with cervical spine x-rays to check alignment and determine the need for additional distraction, as overdistraction may result in neurologic injury. Similarly, overly vigorous attempts to maintain axial distraction using a halo vest system can result in overdistraction, with resultant neurologic injury, dysphagia, and/or pain.
Other complications include pin site infection (soft tissue or osteomyelitis), nerve injury, frontal scarring, pin site loosening, and pin site bleeding. Infection at the pin site may present as local pain, drainage, or as a loose skull pin. For a pin site infection, the skull pin should be removed and a new sterile pin should be placed at a new site. Soft tissue infections usually respond to local care and antibiotics, while osteomyelitis may require debridement. Placement of the pins in the fronto-orbital crest avoids risk of injury to the supraorbital and supratrochlear nerves located more medially. Pin loosening has been reported in 36% to 60% of patients, is usually associated with pain in the absence of infection, and may be due to a fall or blunt trauma. If cranial traction is used with a halo ring device, frequent neurologic examination should be performed, along with cervical spine x-rays to check alignment and determine the need for additional distraction, as overdistraction may result in neurologic injury. Similarly, overly vigorous attempts to maintain axial distraction using a halo vest system can result in overdistraction, with resultant neurologic injury, dysphagia, and/or pain.
SUBLAMINAR WIRING (GALLIE, BROOKS, AND SONNTAG FUSION)
Cable fixation of C1-C2 was first described by Hadra (1) in 1891 and later by Cone in 1937. More recently, three basic sublaminar wire or cable fixation techniques, the Brooks, Gallie, and Sonntag techniques, are commonly used for C1-C2 fixation. Sublaminar wiring techniques share the advantages of surgeon familiarity with these approaches, as well as fusion rates reported in the range of 60% to 100%. The main disadvantages of the procedure are the risk of neurologic injury resulting from sublaminar wire placement and the lack of biomechanical stability attainable when these techniques are used alone. An additional fixation method is required in the form of a hard cervical collar, a halo orthosis, or rigid internal fixation with transarticular screws to achieve improved stability and bony fusion.
SURGICAL TECHNIQUES
The patient is carefully intubated and general anesthesia administered with the patient supine on a stretcher adjacent to the operating table. Meticulous care is required during intubation to prevent flexion or extension of the head and neck. Fiberoptic intubation may be used— allowing for better visualization during intubation. In patients with atlantoaxial instability, awake fiberoptic intubation is recommended to allow continuous monitoring of patients’ neurologic status. Electrophysiologic monitoring with somatosensory evoked potentials and motor evoked potentials should be employed. Baseline potential recordings may be obtained prior to intubation, or alternatively, the patient may be intubated fiberoptically and a wake-up test performed immediately after intubation and once again after positioning.
Once adequate general anesthesia is obtained, a threepoint Mayfield head fixation device is applied to provide rigid head and neck stabilization intraoperatively. The patient is then carefully log rolled onto the operative table in the prone position. The headholder is then affixed to the operating table with the neck in neutral position and slight flexion of the head. Confirmation of proper vertebral alignment using fluoroscopy or static lateral cervical spine x-ray is essential. The skin is then prepped and draped from the occiput to the mid- or lower cervical spine in the standard fashion. Prior to skin incision, the skin may be infiltrated with 1% lidocaine with 1:100,000 epinephrine to facilitate hemostasis. A midline incision is then made from the subocciput to the fourth or fifth cervical vertebrae. Using monopolar cautery and sharp dissection, the midline incision is carried deeper, staying within the avascular intermuscular septum or ligamentum nuchae. The spinous process and lamina of C2 are exposed in a subperiosteal fashion, taking care not to expose the articular processes below C2. The paraspinal muscles are retracted laterally. The dorsal arch of the atlas is then exposed by subperiosteal dissection. Blunt subperiosteal dissection with a Cobb or periosteal elevator should be used along the lateral portions of the C1 arch to avoid injury to the vertebral artery and venous plexus. Similarly, bipolar cautery should be used, and monopolar cautery avoided, when exposing the lateral portions of the C1 arch. The posterior caudal aspect of C1 arch and the dorsal rostral surface of the axis are curetted to remove any soft tissues. The occiput should not be extensively exposed or decorticated, thereby avoiding inadvertent fusion to the skull base (Fig. 138.1).
SUBLAMINAR WIRE PLACEMENT
The C1 dorsal arch and C2 lamina are exposed circumferentially with curettes to facilitate wire passage. A 20-or 24-gauge stainless steel wire or braided titanium cable (either single wire or a “looped” double wire) is passed under the C1 ring in the caudal-rostral direction. This is accomplished using a right-angled clamp or, alternatively, by tying the leading loop of wire or cable to a suture and then passing the blunt end of the suture needle in a sublaminar fashion. Safe passage of the loop may be performed in the midline directly under the spinous process, as there is substantial space in this region. An adequate removal of ligamentum flavum should be performed prior to passage of the sublaminar cable. Similarly, the bone surfaces should be decorticated prior to cable passage in order to allow for bone fusion without risking damage to the cables with the air drill. Depending on the technique used, the loop may then be cut into two single strands, which are then placed laterally. Careful dissection of the atlantoaxial membrane facilitates moving the cables to each side, and great caution must be exercised during this maneuver to avoid injury to the dura and spinal cord.
BONE GRAFT HARVEST
Autologous bone graft is harvested from the dorsal iliac crest superficial to the sacroiliac joint. The posterior superior iliac spine (PSIS) is palpated and prepared as a separate surgical region for any posterior cervical procedure. A diagonal or vertical incision approximately 2 to 3 cm in length is made centered over the PSIS well medial to the
superior cluneal nerves. Horizontal incision risks injury to the superior cluneal nerves. Cortical bone overlying the iliac wing is exposed subperiosteally using monopolar cautery and an elevator. The gluteal musculature is then carefully dissected in a subperiosteal fashion from the dorsolateral surface of the iliac bone. Great care should be taken to preserve the gluteal musculature, as minimizing gluteal dissection decreases postoperative graft site pain. An osteotome or oscillating saw is used to harvest a tricortical graft, and the rostral cortical margin is then removed to create a bicortical graft. The two cortices will interface the inferior rim of the C1 dorsal arch and the rostral margin of C2 lamina so that the bone graft and C1-C2 cancellous regions are closely approximated. The surgeon needs to ensure that the height of the graft is adequate for optimal bone graft-spine contact. Thus, many surgeons choose to oversize the initial bone harvest and then tailor the graft to optimal size. Bone graft length should also be carefully considered. For the Sonntag cable technique, the graft should be at least 4 to 5 cm in length so that it may be incorporated into the construct. For interlaminar clamp technique, the graft must be shorter because the clamps attach lateral to the graft. Regardless of the dorsal fixation technique used, additional bone graft may be morselized and placed between C1 and C2 lamina into the lateral aspects of the construct.
superior cluneal nerves. Horizontal incision risks injury to the superior cluneal nerves. Cortical bone overlying the iliac wing is exposed subperiosteally using monopolar cautery and an elevator. The gluteal musculature is then carefully dissected in a subperiosteal fashion from the dorsolateral surface of the iliac bone. Great care should be taken to preserve the gluteal musculature, as minimizing gluteal dissection decreases postoperative graft site pain. An osteotome or oscillating saw is used to harvest a tricortical graft, and the rostral cortical margin is then removed to create a bicortical graft. The two cortices will interface the inferior rim of the C1 dorsal arch and the rostral margin of C2 lamina so that the bone graft and C1-C2 cancellous regions are closely approximated. The surgeon needs to ensure that the height of the graft is adequate for optimal bone graft-spine contact. Thus, many surgeons choose to oversize the initial bone harvest and then tailor the graft to optimal size. Bone graft length should also be carefully considered. For the Sonntag cable technique, the graft should be at least 4 to 5 cm in length so that it may be incorporated into the construct. For interlaminar clamp technique, the graft must be shorter because the clamps attach lateral to the graft. Regardless of the dorsal fixation technique used, additional bone graft may be morselized and placed between C1 and C2 lamina into the lateral aspects of the construct.
GALLIE TECHNIQUE
Described in 1939, the Gallie technique involves the placement of a wire under C1 and attached to the spinous process of C2 below with an intervening H-shaped bone graft (8). The graft is typically a corticocancellous iliac crest bone graft approximately 1.5 cm by 3 cm and notched caudally in the midline to contour the C2 spinous process during compression. Two variations of the technique exist, both of which eliminate the need for placement of sublaminar wires at C2. A 20-gauge wire is passed under the C1 dorsal arch, the two free wire ends are placed through the leading wire loop, then placed over the bone graft, and either through or under the C2 spinous process. Tightening the construct secures the onlay bone graft to the dorsal arch of C1 and the rostral laminar surface of C2. Alternatively, a doubleloop technique may be used, consisting of a sublaminar wire placed caudal to rostral around the arch of C1 with the cranial loop brought caudally to over the bone graft and under the C2 spinous process. The remaining free ends of wire are then placed transversely over the bone graft and tightened to secure the construct. The primary drawback of this technique is that it represents a single, midline point of fixation. Biomechanically, the Gallie technique is capable of preventing ventral translation and flexion but does not optimally restrict extension and rotational forces. There is also concern when more than one spinal segment is spanned using sublaminar wires, as there is increased risk of encroachment on neural elements within the spinal canal during the passage of wires, as well as the risk of chronic encroachment of the wires over time. This is particularly significant in cases in which ventral translation of the atlas results in spinal canal stenosis. Therefore, although easy to perform, this method is not very stable and requires a rigid orthosis, preferably a halo, for postoperative immobilization. Biomechanically, the Gallie technique is capable of preventing ventral translation and flexion but does not optimally restrict extension and rotational forces (Fig. 138.1).
BROOKS TECHNIQUE
In 1978, Brooks and Jenkins (4) refined the sublaminar wiring technique by using dual sublaminar wires at C1 and C2 with intervening bilateral interlaminar bone grafts. By incorporating bilateral, interlaminar bone grafts, this strategy overcame the rotational deficiencies seen with the Gallie fusion. For this technique, the dual wire is passed caudorostrally under the C2 lamina and C1 dorsal arch. Two separate rectangular corticocancellous grafts are harvested and placed between the caudal margin of the C1 dorsal arch and rostral margin of the C2 lamina. The bilateral dual wires are then tightened to compress and secure the bone grafts. Biomechanical studies have shown that this technique is superior to the Gallie technique in resisting extension and axial rotational forces. However, the Brooks technique carries the additional risk of wire passage at two levels instead of a single level (Fig. 138.1).
SONNTAG TECHNIQUE
In the Dickman et al. modification of the Gallie technique, the use of sublaminar wires under C2 is eliminated and, instead, the spinous process of C2 serves as the fixation point. Intraoperatively, the C1-C2 interlaminar space is widened. The caudal surface of the C2 spinous process is notched, and a loop of wire is then passed sublaminarly in the caudorostral direction under the C1 lamina. A single corticocancellous autograft or allograft strut is used, similar to the Gallie procedure. The wire is then passed over the graft, which can be notched to accept and secure the wire, and is then secured to the undersurface of the C2 spinous process. This secures the bone graft in the interlaminar space. The free ends of the wire are then brought over the C2 laminar surface and secured either by twisting or with a crimp device after adequate tightness has been achieved. The use of a braided multistrand wire facilitates safe wire passage because of its decreased rigidity, resulting in a lower rate of morbidity and allowing for greater tightening and improved strength and fixation (Fig. 138.1).
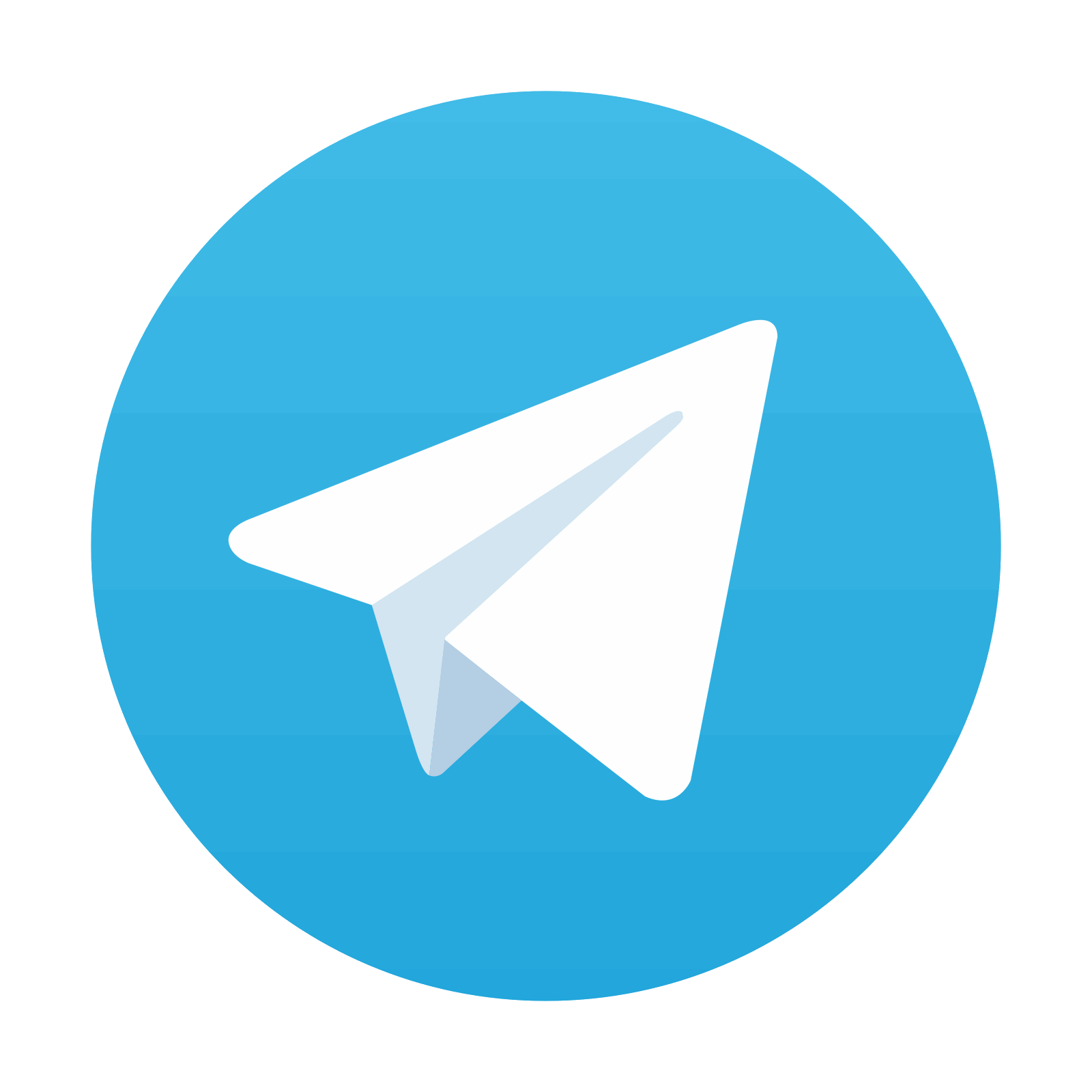
Stay updated, free articles. Join our Telegram channel

Full access? Get Clinical Tree
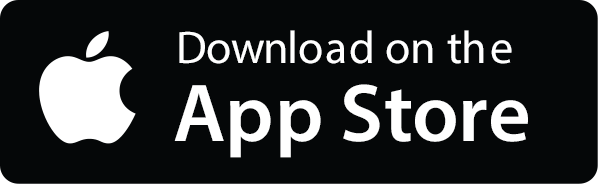
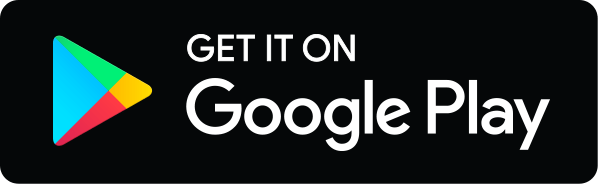