Author, reference
Study design
Stroke
Cell dose
Manipulation
Mode of application
Efficacy
Adverse effects
Safety test
Autologous bone marrow mononuclear cells
Suarez-Monteaqudo, 2009 [40]
No control group Treatment, n = 5
1 year f/u
Chronic Ischemic or ICH
N/A
Isolation using normal saline
Intracerebral
N/A
None
N/A
Battistella, 2011 [41]
No control group Treatment, n = 6
6 months f/u
Subacute MCA infarct
1–5 × 108
Isolation using human albumin-containing normal saline
Intra-arterial
N/A
Seizure after 200 days
N/A
Savitz, 2011 [42]
No control group Treatment, n = 10
6 months f/u
Acute (24–72 h) Large MCA infarct
1 × 106 /kg
Intravenous
mRS 1 shift vs. historical control
None
Cell viability, MSC surface markers, bacteria, fungi, mycoplasma
Friedrich, 2012 [43]
No control group Treatment, n = 20
6 months f/u
Acute (3–7 days) Non-lacunar infarct
2.2 × 108
Intra-arterial
mRS 0–2 in 40 %
None
Cell viability
Li, 2013 [44]
Control, n = 40 Treatment, n = 60
6 months f/u
Acute (5–9 days) ICH
1.33 × 1013
Isolation using normal saline
Intracerebral
NIHSS and Barthel index improved
None
Cell viability
Autologous bone-marrow-derived mesenchymal stem cells
Bang, 2005 [9]
Control, n = 25 Treatment, n = 5
1 year f/u
Subacute Large MCA infarct
1 × 108
Ex vivo culture expansion using fetal bovine serum
Intravenous
Barthel index improved at 3 months
None
Cell viability, MSC surface markers, bacteria, fungi, viral, mycoplasma
Lee, 2010 (STARTING trial) [10]
Control, n = 36 Treatment, n = 16
5 years f/u
Subacute Large MCA infarct
1 × 108
Intravenous
mRS 0–3 increased in MSC but not in control group
None
Honmou, 2011 [11]
No control group Treatment, n = 12
1 year f/u
Subacute to chronic variable
1 × 108
Ex vivo culture expansion using autologous serum
Intravenous
Improvement in daily rate of NIHSS changes
None
Cell viability, MSC surface markers, bacteria, syphilis, fungi, viral, mycoplasma, endotoxin
Bhasin, 2011 [45]
Control, n = 6 Treatment, n = 6
24 weeks f/u
Chronic Ischemic or ICH
5–6 × 107
Ex vivo culture expansion using serum-free media (stem Pro)
Intravenous
Modest increase in Fugl-Meyer and modified Barthel index
None
Cell viability, mycoplasma, endotoxin
We have reported the results of the stem cell Application Research and Trials In NeuroloGy (STARTING) trial, a randomized controlled trial of intravenous application of autologous MSCs, culture expanded with fetal bovine serum, in the subacute phase of stroke [10]. In this study, patients were randomly allocated to one of two groups, those who received intravenous autologous ex vivo cultured MSCs (n = 16) or those in the control group who did not (n = 36). Intravenous autologous MSC transplantation was found to be safe for stroke patients through a long-term period (~ 5 years), and may improve recovery, as evaluated via observer-blind evaluation of modified Rankin scores (mRSs) (Fig. 3.1). More recently, Honmou et al. described dramatic neurological improvements after administration of autologous MSCs, culture expanded with autologous serum, in 12 patients with chronic stroke [11]. However, the mechanisms by which transplanted MSCs result in functional benefits after stroke are unclear. Clinical trials have shown that functional improvement occurs shortly after cell therapy and diminishes with time, suggesting that the effects of MSCs are mediated via trophic support, rather than replacement of damaged cells [9].
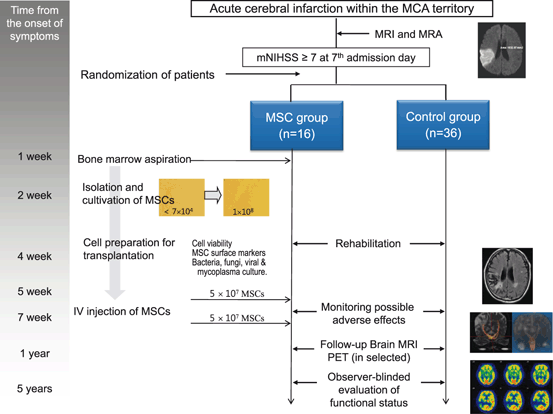
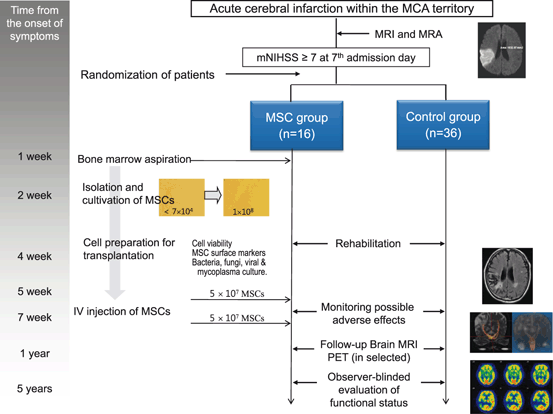
Fig. 3.1
Study protocol for the stem cell Application Research and Trials In NeuroloGy (STARTING) trial. mNIHSS modified National Institutes of Health Stroke Scale, PET positron emission tomography, MRA magnetic resonance angiography
A cost-effectiveness analysis showed that the societal value of stem cell therapy was US$ 166,500, and that therapy was cost-effective under a wide range of assumptions, such as the size of the effects, age, and functional status at discharge [12]. However, it should be noted that none of the studies aimed to evaluate the efficacy of MSC therapy in stroke patients. All of the studies aimed to assess the feasibility and safety of stem cell treatments, compared to conventional treatments, in patients with ischemic stroke. Most studies were small series, and several studies did not even include a control group. Moreover, assessments for functional improvement varied among studies. Therefore, it is too early to determine whether MSC therapy can improve functional outcomes in patients with stroke [13]. In the cardiology field, a recent meta-analysis concluded that transplantation of adult bone marrow cells improved left ventricular function, infarct size, and remodeling in patients with ischemic heart disease compared with standard therapies, and that these benefits persisted. A total of 50 studies (enrolling 2625 patients) were included in this analysis and patients received echocardiographic evaluations and long-term follow-ups in order for researchers to arrive at this conclusion [14]. Interestingly, a recent study showed that patients have unfounded expectations that stem cell therapy will improve function [15]. These fantasies may be a result of inappropriate media coverage and lack of information, and could be a hurdle in conducting randomized control trials of stem cell therapy in stroke patients.
In terms of safety, no adverse cell-related events have been reported in clinical adult stem cell trials in stroke patients. A recent meta-analysis of prospective clinical trials that used intravascular (intravenous or intra-arterial) MSC delivery, which included 1012 participants with ischemic stroke, Crohn’s disease, cardiomyopathy, myocardial infarction, graft versus host disease, and healthy volunteers, showed that MSC therapy was not associated with any systemic complication, infection (except transient fever), malignancy, or death [16].
Considerations for Clinical Trials of MSC Therapy in Stroke Patients
For a stem cell therapy to be effective in augmenting recovery after stroke, the following criteria should be satisfied [17]: therapy should be both safe and effective; applicable to a broad spectrum of stroke patients, in terms of stroke subtypes (ischemic/hemorrhagic), time (acute/chronic), and vascular territories; and it should be inexpensive and cost-effective.
At this time, rigorous reasoning is required to replicate experimental results in stroke patients. The Stroke Treatment Academic Industry Roundtable (STAIR) recommendations were developed to improve the quality of preclinical and clinical research on neuroprotective and neurorestorative drugs, and to reduce the divide between them [18]. Based on these recommendations, studies should be randomized, controlled trials. After randomization, experimental procedures may not be blinded, as bone marrow sham aspiration should not be performed in control patients. A MSC dose equivalent to that used in animal studies (1 × 105–3 × 106 cells/rat), and based on mean body weight, should be used. Patient selection should be based on severity and location of lesions, and time of application. Although the optimal time window for administration is undecided, subacute periods of stroke should be the first candidate time period tested, i.e., after the acute period, when patients are likely to experience rapid worsening/improvement, and before the blood–brain barrier (BBB) closes and chemokine expression (i.e., stromal cell-derived factor 1α) in the infarcted brain disappears. As all preclinical studies were conducted in animal models of middle cerebral artery occlusion, patients with middle cerebral artery territory (or anterior circulation) stroke should be selected. Patients with moderate to severe neurological disabilities could be ideal candidates. Patients suffering from very severe strokes are likely to have poor outcomes regardless of intervention, whereas patients with minor strokes are not suitable for these potentially risky experimental treatments. In addition to the clinical outcomes measured, laboratory and neuroimaging findings should be used as surrogate markers of efficacy. Lastly, patients should be followed for more than 90 days. However, long-term monitoring (> 6 months) is likely unnecessary because autologous MSCs are a clinical cell line and die within days/weeks of administration [19].
Recently, the Stem Cell Therapies as an Emerging Paradigm in Stroke (STEPS) committee suggested guidelines for both preclinical and clinical trials on stem cell therapies in stroke. The committee has suggested guidelines for bridging basic and clinical studies [20], early stage clinical trials [19], and phase II/III trials [21].
Perspectives in Stem Cell Therapy for Stroke
Although, to date, clinical trials of stem cell therapy have focused on the feasibility and safety of application of autologous MSCs or bone marrow cells in stroke patients, further trials to understand the therapeutic effects of stem cells are needed. Although the proportion of patients in the STARTING trial with a mRS of 0–3 significantly increased following MSC treatment, many patients in the MSC group remained significantly disabled [10].
The primary hurdles for current stem cell therapies include: the long culture period required to obtain sufficient stem cells, the relatively small number of MSCs that migrate into the injured brain, the death of stem cells within a toxic environment, limited trophic support by transplanted stem cells, and the use of xenogenic serum, with concomitant risk of transmission of prion diseases and zoonoses [22]. The following issues should be addressed in order to overcome these hurdles.
Allogenic Cells
Allogenic MSCs could be a good alternative to autologous MSCs for a number of reasons. First, stroke commonly occurs in advanced age, often in conjunction with chronic illness, and MSCs from these patients can show reduced growth rates. In addition, although titers of hematopoietic bone marrow stem cells remain constant throughout life, the number of MSCs decline dramatically with age [23]. Second, the earlier the MSCs can be administered, the more the MSCs can migrate to the infarcted brain. The use of allogenic MSCs reduces the time required to obtain a sufficient number of cells. Finally, MSCs are both immunosuppressive and immunoprivileged, expressing little to no major histocompatibility class II or costimulatory molecules [24]. However, it has been reported that after contact with serum, allogenic MSCs can be injured by complement, and that viability of allogenic MSCs after infusion is greatly reduced compared to autologous MSCs [25].
Mode of Treatment
The mode of application of MSCs may significantly influence the number of cells delivered to target regions, as well as the incidence of adverse effects. A major problem in introducing stem cells systemically is that cells may become trapped within organs that filter the bloodstream (first-pass effect). To avoid this, strategies to minimize lung adhesion and improve homing of systemically introduced cells are used, including the use of vasodilators, reduction in number of cells administered, or different routes of administration. For example, an intra-arterial approach can bypass pulmonary circulation; intra-arterial transplantations resulted in superior delivery and sustained presence of stem cells in the ischemic brain compared to intravenous infusions [26]. However, an arterial approach may cause arterial occlusion, resulting in stroke, and is reportedly not superior to intravenous approach for recovery after stroke [26, 27]. There have been relatively few studies directly comparing the efficacy of intravenous and intra-arterial delivery of MSCs. Although both intravenous and intra-arterial administration of MSC leads to functional recovery, the method of delivery may fundamentally alter their mechanism of action [28].
Extracellular Vesicles
There may be problems associated with stem cell therapy including tumor formation, vascular occlusion causing infarcts, large cells resulting in limitations in crossing the BBB (especially during the chronic stage of stroke), and zoonosis by internalization of xenogenic serum (fetal bovine/calf serum) by MSCs during ex vivo culture expansion [22]. Stem cells secrete extracellular vesicles (EVs; e.g., microvesicles, exosomes) as well as soluble factors (e.g., trophic factors). Cell therapy using EVs derived from stem cells could avoid the cell-related problems described above, and could represent a new, clinically feasible and relatively safe paradigm. We have recently reported that strokes in humans trigger the mobilization of MSC-derived EVs [29]. The numbers of circulating MSC-derived microvesicles increased in patients with extensive ischemic stroke. However, the role of these microvesicles, and their biodistribution during stroke, is unclear. We and others have shown that intravenous administration of EVs derived from MSC culture media promote functional recovery and neurovascular plasticity after stroke in rats [30].
BBB Manipulation
Molecules larger than 400 Da cannot pass through the BBB, which may affect the efficacy of cell therapy in stroke patients. Coadministration of stem cells and mannitol, an osmotic agent that shrinks and opens the BBB, may improve outcomes in stroke patients. In a preclinical study, BBB manipulation using intravenous mannitol prior to MSC treatment resulted in increases in trophic factors in the infarcted brain [31]. As mannitol is already widely used in clinical practice, BBB manipulation using mannitol should be considered in the future clinical trials.
Culture Expansion Conditions
Current culture methods need to be improved. During a long period of ex vivo culture expansion, MSC characteristics may change significantly [5]. Several methods of culture expansion that improve the proliferation, survival, and trophic support of MSCs, and reduce senescence have been reported. First, hypoxic conditions (i.e., conditions similar to bone marrow) are beneficial to MSCs and may stimulate MSCs to exhibit adaptive responses. Preconditioning with hypoxia (0.1–2 % O2) [32–36] reportedly increases anti-apoptotic gene expression, trophic factor release, ischemic tolerance, and C-X-C chemokine receptor type 4 expression. Second, treatment with trophic factors may alter MSC characteristics. We have shown that ex vivo trophic factor treatment during MSC cultivation enhances trophic support in the ischemic brain and further increases the production of trophic factors by MSCs, suggesting autocrine regulation of MSCs [37].
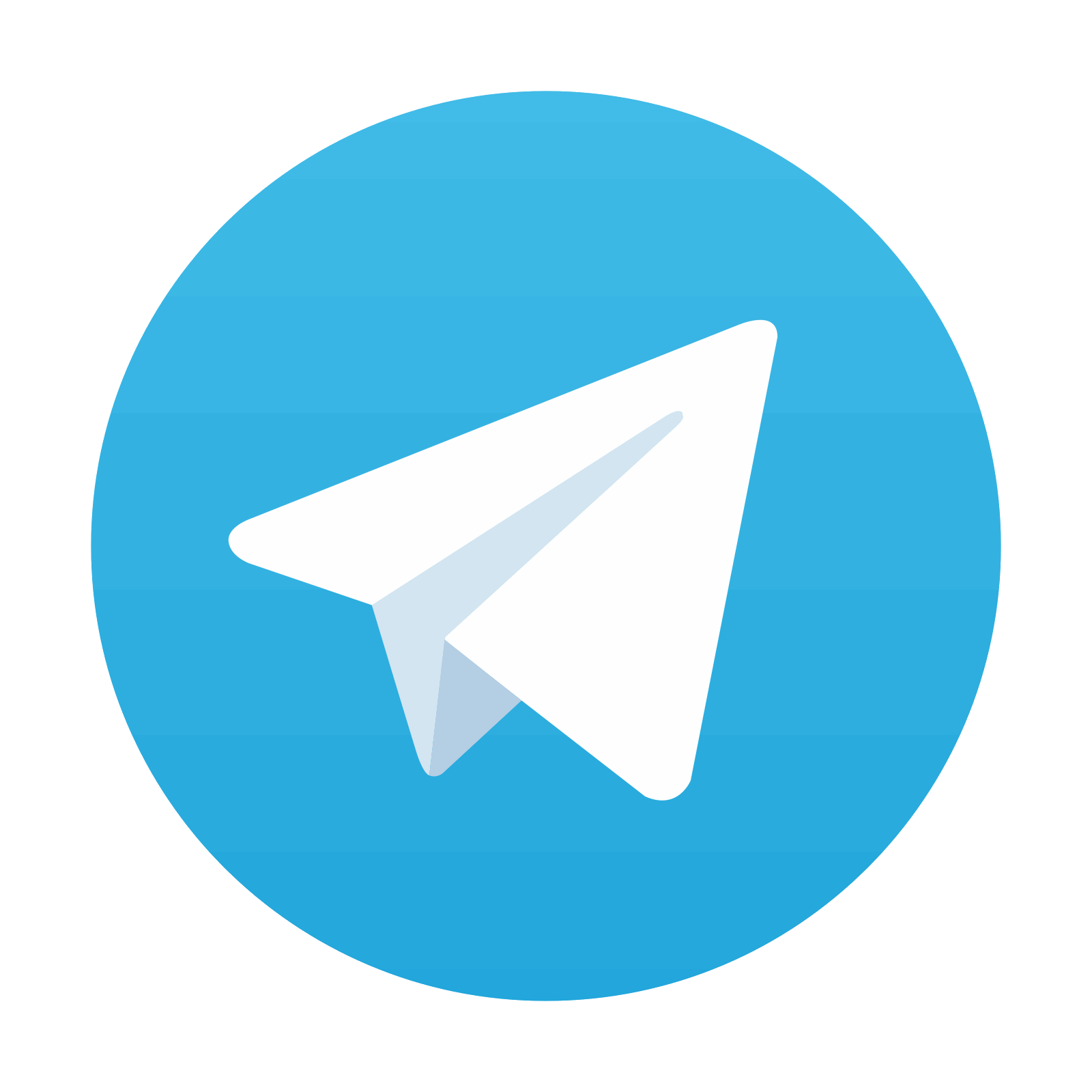
Stay updated, free articles. Join our Telegram channel

Full access? Get Clinical Tree
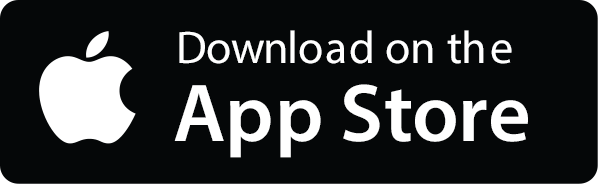
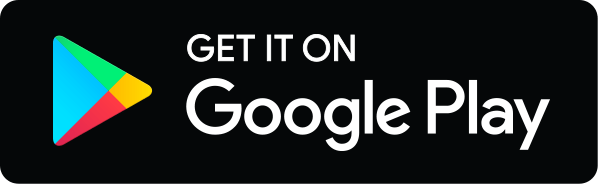