properties such as solubility and mechanical strength are altered because cross-links are formed nonenzymatically, as compared to enzymatically mediated pyridinoline cross-linking found in young, healthy disks (28,29). Compared to the relatively constant collagen content, PG content in the NP decreases noticeably with age and degeneration (30, 31, 32 and 33), ultimately diminishing the waterholding capacity of the IVD and reducing its ability to distribute loads effectively. In the AF, however, the water content remains relatively constant, and chemical mediators may play a more significant role in annulus degeneration (34). Importantly, enzymatic by-products of degeneration, such as fibronectin and aggrecanase fragments, may further promote the degeneration process in both NP and AF tissues(35,36).
PG synthesis, with more pronounced effects in the NP compared to AF. Since the Thompson et al. (55) landmark report, several studies have demonstrated the importance of biologic factors in IVD homeostasis (49,50) (Table 99.1). Below, we review several pertinent biologic factors that have been shown to slow or reverse disk degeneration in vitro and/or in vivo, elucidating the significant potential for clinical application of biologic factors in the future.
synthesis of PGs and collagens in a dose-dependent manner with an associated increase in the expression of mRNA for aggrecan and collagen type II. In addition, continuous treatment with rhOP-1 stimulated the accumulation of ECM as well as an increase in cell number, suggesting that the stimulation of matrix synthesis is associated with the retention of newly synthesized matrix molecules. Although data suggest that the capacity of NP and AF cells to respond to growth factors diminishes with age (61), it has been found that the responsiveness of IVD cells to OP-1 is independent of age (60). Further, Imai et al. (65,66) reported that OP-1 enhanced the in vitro production of PG by human NP and AF cells cultured in alginate beads. Similar to rabbit cells, OP-1 also enhanced the accumulation of PG in the matrix. Interestingly, AF cells, which are more fibrochondrocytic than NP cells, strongly responded to OP-1, suggesting that OP-1 might be beneficial not only for nucleus repair but for annulus repair as well (65).
TABLE 99.1 In Vitro and In Vivo Studies of the Effects of Biologic Factors on IVD Homeostasis | ||||||||||||||||||||||||||||||||||||||||||||||||||||||||||||||||||||||||||||||||||||||||||||||||||||||||||||||||||||||||||||||||||||||||||||
---|---|---|---|---|---|---|---|---|---|---|---|---|---|---|---|---|---|---|---|---|---|---|---|---|---|---|---|---|---|---|---|---|---|---|---|---|---|---|---|---|---|---|---|---|---|---|---|---|---|---|---|---|---|---|---|---|---|---|---|---|---|---|---|---|---|---|---|---|---|---|---|---|---|---|---|---|---|---|---|---|---|---|---|---|---|---|---|---|---|---|---|---|---|---|---|---|---|---|---|---|---|---|---|---|---|---|---|---|---|---|---|---|---|---|---|---|---|---|---|---|---|---|---|---|---|---|---|---|---|---|---|---|---|---|---|---|---|---|---|---|
|
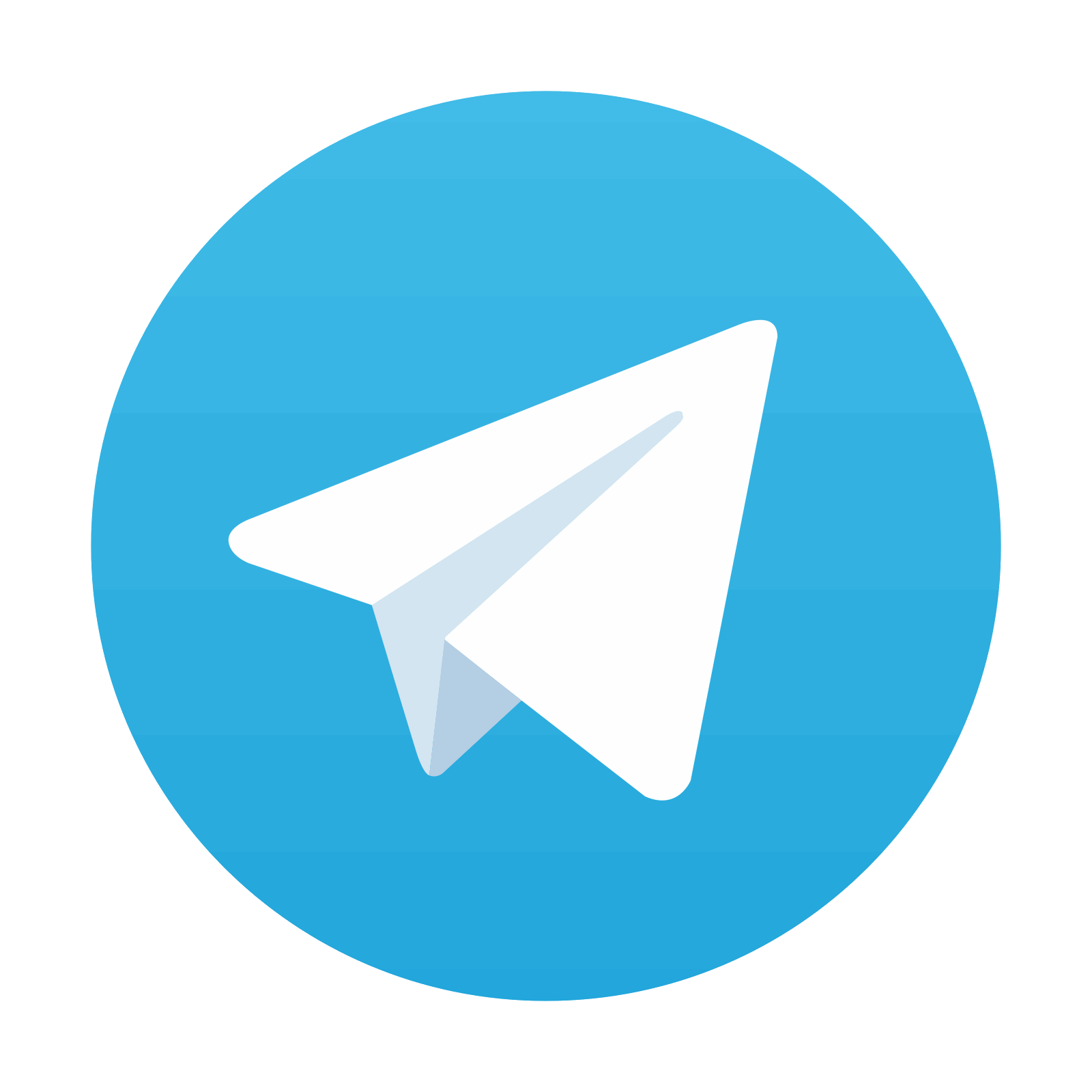
Stay updated, free articles. Join our Telegram channel

Full access? Get Clinical Tree
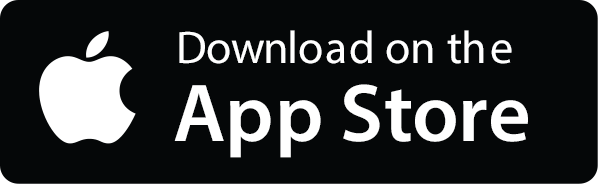
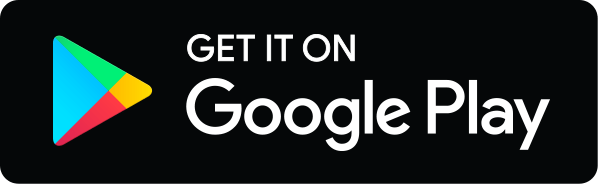