Introduction
Acute brain injuries triggered by head trauma, intracranial aneurysm rupture, cardiac arrest, and stroke among others are an enormous medical, social, and economic problem. Acute traumatic brain injury (TBI) is the most common cause of death and disability in children and young adults. Cardiac arrest kills about 300,000 individuals annually in the United States, and even successful resuscitation of out-of-hospital cases leads to neurologic dysfunction in the vast majority of instances ( www.ctsnet.org/doc/2988 ). Approximately 800,000 coronary artery bypass surgeries are performed yearly in the United States, and it is estimated that as many as 80% are associated with postsurgical impairments in neurologic or cognitive function. More than 750,000 strokes occur in the U.S. annually, and treatment with clot-dissolving drugs is indicated for only a small proportion of cases. About 30,000 cases of aneursymal subarachnoid hemorrhage (SAH) occur per year, and severe aneurysmal SAH carries a high risk of brain damage and neurologic dysfunction, especially when accompanied by cerebral vasospasm.
Treatments conducted in the neurocritical care unit (NCCU) and emergency department are directed at the pathophysiologic processes that occur with acute brain injuries. Progress is being made, in part through the use of neuromonitoring and neuroimaging to better understand these pathophysiologic processes, identify deleterious risk factors, improve preservation of brain tissue, and reduce mortality, morbidity, and long-term brain dysfunction. However, it has been difficult to assess in a rigorous fashion the relative merits of the many treatment options available in the NCCU, and considerable strides still need to be made to identify patients at risk for irreversible brain damage, detect early and potentially treatable stages before the onset of irreversible injury, rapidly identify patients who most benefit from specific treatment measures to reduce subsequent neurodegeneration and brain dysfunction, and optimize treatment parameters for reducing brain damage and improving outcome. Furthermore, despite 25 years of extensive basic and clinical research into intraneuronal signaling mechanisms for neuron death and pharmacologic strategies for neuroprotection, there still are no U.S. Food and Drug Administration (FDA)–approved neuroprotective therapies for any form of acute brain injury.
Recent multidisciplinary workshops (e.g., Classification of TBI for Targeted Therapies, October 2007; Common Data Elements workshop for Research and Psychological Health in TBI, March 2009, Comparative Effectiveness Research in TBI, June 2010) have identified important reasons for the discordance between laboratory advances and clinical success particularly in TBI. Included among the recommendations to address this is the application of emerging technologies (e.g., biomarkers, genomics, and advanced magnetic resonance imaging [MRI]) to better understand pathophysiology, help classify disease heterogeneity, and examine treatment effects. Simple diagnostic and prognostic tests sensitive to the onset and magnitude of brain damage could have widespread and far-reaching applications in the NCCU, and in particular help identify patients at risk of irreversible brain injury and dysfunction. In TBI, computed tomography (CT) and MRI can confirm the diagnosis of moderate to severe brain injury. However, CT scanning does not efficiently diagnose mild or repetitive mild TBI, which can lead to neurodegeneration and neurologic dysfunction, and MRI has suboptimal sensitivity. Similarly, the impact of mild stroke or coronary bypass surgery remains difficult to quantify with routine neuroimaging methods. Prognostic tests that define patients at high risk for death or disability and sensitive tests to detect secondary injuries also could guide the direction and aggressiveness of patient care. There is a variable risk for ischemic brain damage after cardiac arrest, and currently there are no methods to identify the subset of patients susceptible to irreversible brain injury that would benefit most from neuroprotective treatment. The significance of this shortcoming is heightened with the success of induced hypothermia after cardiac arrest. Although this helps preserve short- and long-term brain function, the treatment is complex, expensive to administer, efficacious for only a subset of resuscitated patients, and dependent on treatment variables that have yet to be optimized.
Following severe SAH or TBI, monitoring often generates data about the onset, severity, and location of secondary pathologic events through measures of blood flow, energy metabolism, local oxygen partial pressure, and electroencephalographic activity. However, there are no direct indices or surrogate measures for the onset and magnitude of irreversible brain damage. There are many treatment interventions that still need to be evaluated fully or optimized in controlled clinical trials, for example, the relative merits of mannitol or hyperosmotic saline; determining the optimal osmolarity or target pressures when using hyperosmolar therapy; the role of induced normothermia; finding the optimal glucose or hemoglobin; different ventilation strategies; and how best to prevent venous thrombosis, among many others that have to be answered in the NCCU. These questions can be difficult to study in a controlled trial, in part because of the sample size needed, the complexity of patient care, and the insensitivity and imprecision of commonly used functional outcome measures. Surrogate markers for irreversible acute brain damage could play a major role in helping to answer these questions in the NCCU. The clinical evaluation of potential neuroprotectant drugs also often requires large and expensive trials that can present a barrier for pharmaceutical and biotechnology companies with promising but unproven experimental therapeutics. Surrogate measures for efficacy that reduce the complexity and cost while increasing the discriminative power of early-stage drug trials would facilitate clinical research for novel neuroprotectants and help drug discovery and development. In addition, use of state-of-the-art biomarker platforms may help during the recovery phase of any acute brain injury to identify effective therapies to enhance recovery in a targeted and individualized fashion. This concept may apply not only to strategies used in rehabilitation after moderate or severe TBI but also to managing the cognitive or neurobehavioral sequelae even after mild injury with techniques such as evaluation of micro-ribonucleic acid (RNA) species in peripheral blood mononuclear cells.
There are many potential uses for surrogate markers for brain damage in preclinical studies. This is especially true for markers that relate directly and mechanistically to the underlying molecular pathways for neurodegeneration. Rather than relying solely on neurologic tests that reflect in complex and often indirect ways the anatomy, pathology and physiology of brain damage, or histopathologic methods that restrict analyses to the time of sacrifice, it may be possible to examine mechanisms of neurodegeneration and assess their temporal characteristics by serial sampling in living animals using surrogate markers. These markers also could help identify optimal dosing, evaluate specific neurodegenerative mechanisms, or define efficacy of neuroprotective therapeutics over time in living animals. Furthermore, a surrogate marker with the sensitivity to detect mild brain damage may facilitate mechanistic and experimental therapeutic studies of both single and repetitive mild insults, and of factors that regulate the threshold for brain damage. Finally, comparative analyses of a panel of potential surrogate markers for brain damage in human clinical samples and preclinical experimental models should provide new information about the clinical relevance of various animal model systems, none of which have been validated pharmacologically because of the lack of clinically proven neuroprotective drug treatments.
Desirable Characteristics of a Surrogate Marker for Brain Damage
Biomarker (biologic marker) was introduced in 1989 as a Medical Subject Heading (MeSH) term. In 2001 a National Institutes of Health (NIH) working group standardized the definition of a biomarker as “a characteristic that is objectively measured and evaluated as an indicator of normal biologic processes, pathogenic processes, or pharmacologic responses to a therapeutic intervention.” The working group also described different types of biomarkers including that measured on a biosample (e.g., blood, urine, cerebrospinal fluid [CSF], or tissue), a recording obtained from a person (e.g., blood pressure, electrocardiogram [ECG]), or an imaging study (e.g., MRI or CT scan). This chapter focuses on biosamples. Several recent developments have stimulated the biomarker field: first, the completion of the Human Genome Project and the growth of microarrays, proteomics, and nanotechnology provides new methods to develop sensitive high-throughput assays. Second, advances in bioinformatics and cross-disciplinary collaborations have enhanced the ability to retrieve and analyze large quantities of data. Third, there now is understanding that diseases develop from the dynamic dysregulation of several gene regulatory networks, proteins, and metabolic alterations that reflect complex perturbations (genetic and environmental) of the “biologic system.” Best practice guidelines to standardize the quality and accessibility of biosamples and so realize the full potential and accelerate genomic, proteomic, and metabolomic research in acute brain injury have been published. It is beyond the scope of this chapter to review the many technical, financial, legal, and regulatory hurdles that have to be overcome to develop clinically acceptable and validated biomarkers that are commercially produced and FDA proved.
To evaluate patients with potential acute brain injuries, the measurement of biochemical markers from a CSF source are useful in many settings, whereas serum measures would have broader utility. Studies also suggest that use of microdialysis (i.e., from brain interstitial fluid) to recover some biomarkers may be feasible. An ideal CSF or serum marker should have several characteristics, including: (1) high degrees of sensitivity and specificity for brain damage, (2) appearance only after irreversible degeneration of brain tissue, (3) rapid transit to the bloodstream in the case of serum markers, and (4) correlations with the time course and extent of brain injury. There should be low variability in the measurement of an ideal marker to guarantee a predictable relationship between its concentration in CSF, serum, or urine and the degree of brain injury. The ideal marker should provide information that is unavailable from other clinical data alone, for example, rapid diagnosis of at-risk patients, and levels should be responsive to treatment interventions that improve brain function, thereby providing a pharmacodynamic measure of irreversible brain damage. Finally, there should be a reliable and facile assay for the ideal marker with the simplicity, speed, and low cost needed to quickly inform and direct patient care and achieve widespread and routine application.
Current Status of Surrogate Markers
A surrogate measure for brain damage that meets the stringent requirements of the ideal marker has not emerged yet. However, several biochemical markers have demonstrated promise in preclinical and clinical studies and so raise the possibility that a simple cerebrospinal fluid, blood, or urine test for acute brain damage is feasible. Examples of clinical studies are summarized in Table 18.1 . Conceptually, proteins that are expressed primarily in the nervous system could appear outside the central nervous system (CNS) preferentially under pathologic neurodegenerative conditions that are accompanied by disruption of the blood-brain and blood-cerebrospinal fluid barriers. Consistent with this concept, it is well-established that TBI, ischemia, stroke, or SAH among others cause localized, reversible breakdown of the blood-brain barrier that, although transient, can persist for several hours or occur even days following the insult. In addition, certain nervous system–enriched proteins are detectable in human CSF and serum following brain injury or in association with chronic neurodegenerative disease. So far the development of clinically useful surrogate markers has been most successful in the prion disorder Creutzfeldt-Jakob disease, for which CSF measurement of the brain-enriched 14-3-3 protein family is accepted into clinical practice as a diagnostic marker for the disorder.
Biomarkers | Source | Key Finding | Reference |
---|---|---|---|
TRAUMATIC BRAIN INJURY | |||
S100β, NSE | Serum | Prognostic for poor neurologic outcome | |
Tau | CSF | Prognostic for long-term outcome | |
α-Spectrin | CSF | Related to CT findings, long-term outcome | |
Cleaved tau | CSF | Prognostic for elevated intracranial pressure and long-term outcome | |
SUBARACHNOID HEMORRHAGE | |||
NFL | CSF | Prognostic for long-term outcome | |
S100β, GFAP | Serum | Associated with hemorrhage type, neurologic dysfunction, intracranial pressure | |
pNFH | CSF, serum | Associated with vasospasm | |
CARDIAC ARREST WITH RESUSCITATION | |||
S100β | Serum | Prognostic for mortality | |
S100β, NSE | Serum | Prognostic for mortality (both) and memory dysfunction (S100β) | |
STROKE | |||
S100β, GFAP | CSF | Predictive of infarct volume | |
S100β | Serum | Prognostic for long-term neurologic outcome and related to infarct volume | |
Tau | CSF | Prognostic for long-term neurologic outcome and related to infarct volume |
Glial Proteins
S100β
Several non-neuronal proteins whose expression either is brain enriched or induced in the brain in response to neuronal injury have been evaluated in preclinical and clinical studies as potential surrogate markers for acute brain damage. The most extensively studied is S100β, an astrocyte-enriched protein. S100β expression is increased with the astroglial hypertrophic response to brain injury, and increases are detectable based on two-antibody sandwich immunoassay in CSF and serum of patients following TBI, SAH, cardiopulmonary bypass surgery, cardiac arrest with resuscitation, carbon monoxide poisoning, and ischemic or hemorrhagic stroke. Many of these studies demonstrate a prognostic relationship between serum or CSF level of S100β and neurologic outcome, mortality, or secondary cerebral insults (e.g., elevated intracranial pressure), although there is no consensus on cutoff values or time of sampling. Furthermore, there is a suggestion that S100β elevation may be an indicator of impending deterioration, for example, an increase in intracranial pressure (ICP) or of when to obtain a head CT scan in mild TBI. In severe TBI, maximal serum levels of S100β are associated with poor long-term function assessed by the Glasgow Coma Scale, and some studies suggest that after multivariable analysis models that include mass lesion, pupils, S100β and another astrocyte marker, glial fibrillary acidic protein (GFAP), best predict death and unfavorable outcome. In SAH, admission serum level of S100β is associated with short-term survival, injury severity as assessed by neuroimaging, and poor outcome at 1 year, whereas therapeutic intervention with external ventricular drainage is associated with reduced serum S100β level.
However, not all studies have found that use of S100β is reliable to discriminate severity of injury or prognosis. Several limitations also are associated with S100β as a biomarker for acute brain injury. First, high serum concentrations of S100β are observed in association with chronic diseases unrelated to acute brain damage ; this can occur in humans even in the absence of brain injury and may be released after injury by tissue other than the brain. Second, not all types of brain damage are associated with measurable increases in serum concentration of S100β Third, S100β elevations are not always predictive of long-term neurologic dysfunction and have shown inconsistent prognostic value in mild forms of acute brain damage. In children, these inconsistencies may be associated with patient age. Fourth, the data that demonstrate a positive relationship between serum and CSF levels of S100β and patient prognosis have been largely derived from retrospective analysis, that is, cutoff biomarker levels that relate to distinct outcomes all have been selected from observing the data post-hoc. Consequently, multiple, reproducible studies have yet to establish definitively the clinical utility of S100β as a diagnostic or prognostic measure of acute brain injuries, or as a pharmacodynamic tool to measure treatment effectiveness. Finally, methodologic considerations add variance to the clinical measure of S100β that will require both independent confirmation and interlaboratory standardization to promote the prognostic utility of the biomarker. In summary, although certainly worthy of study, S100β is not yet established as either a specific and broadly applicable surrogate marker for acute brain damage, or a highly reproducible and consistent index of short-term and long-term prognosis that may be used in a defined clinical application in a prospective fashion across multiple laboratories.
Other Glial Proteins
There have been fewer studies on other glial-specific proteins that show promise as candidate biomarkers for acute brain injuries; these include the astroglial GFAP and the oligodendroglial myelin basic protein (MBP). In SAH, serum levels of GFAP on hospital arrival are associated with scales of neurologic dysfunction and CT Fisher grade scores, and increase in patients with rebleeding or ischemic events. Serum GFAP levels at admission are associated with abnormal head CT findings, mortality, and poor long-term outcome following severe TBI; 20- to 30-fold increases are observed in patients with poor outcome. In pediatric TBI, initial serum MBP and S100β levels correlate with poor outcome, although the relationship is stronger for children 4 years or younger. Serum and CSF GFAP levels also are elevated in the severe pediatric TBI. Although this elevation is associated with Pediatric Cerebral Performance Category scores at 6 months, the GFAP increase does not discriminate admission injury severity or differentiate therapeutic strategies. In children who receive extracorporeal membrane oxygenation, peak GFAP levels are higher in children with brain injury than those without. It remains uncertain, however, whether these glial markers can guide therapy.
Neuronal Proteins
Several nervous system–enriched proteins expressed predominantly or exclusively in neurons have been studied as potential surrogate markers for acute brain injuries, including tau, neuron-specific enolase (NSE), neurofilament polypeptides, and α-spectrin (see Table 18.1 ).
Tau
The microtubule-associated protein tau is increased in CSF and serum of severely head-injured patients primarily as tau proteolytic fragments of approximately 30 to 50 kDa of indeterminate sequence rather than the intact protein and may serve as indicators of outcome. Zemlan and colleagues developed a monoclonal antibody preferentially reactive with these unidentified tau fragments and established a two-antibody immunoassay to provide evidence for measurable elevations in serum tau concentrations in association with human TBI. However, serum-cleaved tau was not detected in several head-injury cases, and without an unambiguous characterization of these tau fragments, it is difficult to relate their appearance in body fluids to mechanisms that underlie neurodegeneration.
Neuron-Specific Enolase
NSE is another neuronal protein that has been studied extensively as a surrogate marker for acute brain damage. The results, however, are mixed. In some studies the magnitude of serum NSE elevation is associated with long-term brain functional outcome following cardiopulmonary resuscitation. However, the cutoff serum NSE level for maximal prognostic sensitivity and specificity was selected in a post-hoc fashion. Thus it remains to be determined whether serum NSE measures have sufficient reliability and robustness for prospective applications in routine clinical practice. In severe pediatric TBI, serum NSE changes have a poor association with outcome in children less than 4 years old or in victims of inflicted TBI. In severe adult TBI, both NSE and S100β serum levels correlated with contusion volumes or episodes of reduced cerebral perfusion pressure (CPP), but their respective release patterns over time differ for cases of primary cortical contusion, diffuse axonal injury, and cerebral edema without focal mass lesions. Increases in NSE have been observed in boxers with predominantly blows to the head rather than the body and after an extended resting period that suggests repetitive head trauma results in sustained release of this brain-specific protein to the peripheral circulation. In SAH, serum NSE levels are not always elevated. Consequently, whereas NSE has received increased attention as a marker for brain damage associated with cardiac arrest and resuscitation, its use in other acute brain injuries appears thus far to be limited.
Neurofilaments
Neurofilaments are the intermediate filaments of neurons, and because of their unique protein composition the constituent neurofilament triplet polypeptides neurofilament heavy chain (NFH), neurofilament middle chain (NFM), and neurofilament light chain (NFL) have been evaluated as markers for acute brain damage in several studies. In SAH, CSF levels of a particular phosphoform of NFH are increased in patients with poor 3-month outcome, whereas serum NFH phosphoform levels increase when there is cerebral vasospasm. A particular hypophosphorylated form of NFH is expressed in neurons predominantly within axons, and CSF alterations in this phosphoform have been proposed as an index of axonal damage. CSF NFL levels also have been shown to increase following severe TBI or SAH and reportedly correlate with long-term outcome following both cardiac arrest and resuscitation and SAH. However, other studies suggest that although CSF NFL concentrations are elevated in SAH, especially those with acute ischemia, there is no association with outcome suggesting that neurofilament as a biomarker may be better suited to TBI when there is axonal injury. Consistent with this, the level of neurofilament protein heavy chain in serum is not associated with brain ischemia on routine brain imaging techniques after carotid endarectomy. Proteolytic breakdown products of neurofilament heavy chains can be recovered using microdialysis, and these brain extracellular fluid markers might be of prognostic value.
Spectrin
Another cytoskeletal protein that is expressed in neurons and shows promise as a systemic marker for acute brain damage is the actin-binding protein spectrin. The alpha subunit of the nonerythroid form of spectrin, referred to as αII , although expressed in a diverse number of cell types, is expressed at high levels in neurons. Levels of αII-spectrin and spectrin breakdown products (SBDP145 produced by calpain, and SBDP120 produced by caspase-3) increase in CSF following severe TBI, SAH, and cardiac arrest with resuscitation, and the increases are associated with various measures of patient outcome. In addition is a correlation between patients with longer ICP elevations and CSF αII-SBDPs. The specific nature of these breakdown products and their relevance to the pathophysiologic processes that underlie acute neurodegeneration are described further in the following section.
In summary, there are a number of glial- and neuron-enriched proteins and markers from brain endothelial cells that have shown promise as diagnostic or prognostic indicators of acute brain damage deserving of further study, but unfortunately none has emerged yet as a validated and reliable prospective marker with routine clinical applicability. In addition, whether a combination of markers is more valuable than single markers is still to be validated. Moreover, the CSF and serum elevations in the most widely studied biomarkers have yet to be related to underlying pathophysiologic brain processes or brain regions and neural pathways being impacted, and with the exception of a single NFH phosphoform, to neuronal subcellular elements that may be preferentially affected.
Proteolytic Mechanisms for Acute Neurodegeneration That Could Be Exploited to Develop Surrogate Markers
The biochemical signaling pathways that underlie neurodegeneration offer the prospect to develop mechanism-based markers for brain damage potentially measurable in accessible body fluids. In particular, apoptotic and necrotic neurodegenerative signaling are characterized by the activation of distinct families of cysteine proteases, and the proteolytic “fingerprints” of degraded protein substrates for these cell death–activated proteases have been used widely as tissue markers for experimental neurodegeneration. Most modes of apoptotic neuronal death are triggered by the activation of caspases, cysteine proteases with the unusual specificity for cleavage of protein substrates on the carboxyl side of aspartate residues. Caspase activation and neuronal apoptosis have been demonstrated as contributing factors in numerous studies of acute brain damage after TBI, global and focal cerebral ischemia, and hypoxia-hypoglycemia. Eleven human caspases have been identified, at least 8 of which are expressed in the brain and may be activated during apoptosis. These proteases are synthesized as inactive zymogens and require proteolytic processing and oligomeric assembly for their activation. Consequently, cleavage site-specific antibodies have been prepared that react preferentially with activated, processed subunits of many of the caspases, and these have facilitated the biochemical quantitation and histochemical localization of the activation of caspases 3, 6, 8, and 9 that occur during acute brain damage. Furthermore, the substrate specificity of caspases is determined primarily by short domains of four or five amino acids that end in an aspartate residue, making it possible to design and prepare cleavage site-specific antibodies that react specifically with preferred caspase recognition motifs. Cleavage site-specific antibodies reactive with caspase-derived proteolytic fragments of lamin A, αII-spectrin, polyADP-ribose polymerase, actin, and other caspase substrates have been useful tissue markers for caspase-mediated apoptosis. Thus far the only caspase substrates evaluated as potential CSF and serum markers for acute brain injury have been the caspase-1 products interleukin-1β and interleukin-18. However, specificity of these proinflammatory cytokines as markers for brain damage has been limited by their abundant expression in hematopoietic cells that mediate diverse inflammatory processes throughout the body. Recent clinical studies however suggest serum interleukin-1β and to a lesser extent tumor necrosis factor alpha (TNFα) may show promise as markers of poor outcome or intracranial hypertension after TBI. The identification of additional caspase substrate proteins that are expressed at high levels predominantly in the brain, degraded during apoptotic neurodegeneration in association with acute brain damage, and leaked into the CSF or blood in an injury-specific manner could lead to the identification of a mechanism-based surrogate marker for the apoptotic component of acute brain injury.
Activation of the calpain family of calcium-activated cysteine proteases has been linked in numerous studies to necrotic neurodegeneration. Calpains are inactive at normal, physiologic intraneuronal free calcium concentrations, but are activated by the intraneuronal calcium overload that accompanies excitotoxicity; global and focal cerebral ischemia; traumatic injury to the brain, spinal cord, and peripheral nerves; hypoxia-hypoglycemia; status epilepticus; oxidative and nitrative stress; and mitochondrial respiratory failure. Calpains also are activated in some instances of apoptosis, and very brief episodes of calpain activation can occur without neurodegeneration. However, strong and sustained calpain activation without an accompanying caspase activation is diagnostic for neuronal necrosis. One of the most abundant high-affinity calpain substrate proteins is the alpha subunit of spectrin, and cleavage site–specific antibodies reactive with calpain-derived fragments of α-spectrin, but not with caspase-derived fragments or the spectrin holoprotein, have been used widely as cell and tissue markers for calpain activity. They have linked sustained calpain activation with the necrotic neurodegeneration that accompanies TBI, global and focal cerebral ischemia, and hypoxia-hypoglycemia. Similar to the caspase-derived protein fragments, the immunodetection of calpain substrate protein fragments that are generated from brain-enriched proteins during neuronal necrosis and enter the CSF or bloodstream with acute brain damage could be valuable mechanism-based surrogate markers for the necrotic component of acute brain injuries.
Novel Approaches to Identify Potential Surrogate Markers for Brain Damage
Enormous strides have been made with proteomics methods to identify proteins in complex biologic samples, and these methods now are being applied to define CSF and serum proteomes and analyses of changes in the levels of specific proteins in association with disease states. Protein separation and identification are performed by either two-dimensional polyacrylamide gel electrophoresis (2D PAGE), liquid chromatography (LC), mass spectrometry (MS), or combinations of 2D PAGE, LC, and MS. Although it may be theoretically possible to measure a protein within protein-rich serum whose levels change in relation to the time course and extent of brain damage, no such marker protein has been identified yet by proteomics methods. The sensitivity of detection by 2D PAGE or MS approaches may need to improve to detect the expected minute (attomolar [10 –18 molar]) quantity of brain-enriched proteins that may circulate in the blood post injury. The complexity of methods for MS and their considerable expense are further barriers to their adoption into routine clinical practice. Although proteomics approaches to identify and quantitate surrogate markers for acute brain damage have promise, it remains to be seen whether they offer sufficient simplicity, sensitivity, and specificity to identify and quantify surrogate markers for brain damage that will have broad clinical applicability.
Unlike proteomics methods, immunoassays may offer the simplicity, sensitivity, and specificity that would be required of a clinically useful surrogate marker for brain damage. Rather than evaluating target antigens one by one as potential bases for a surrogate measure for brain damage in accessible body fluids, as has been conducted so far, a biologically based approach to identify numerous potential surrogate markers could expedite the development and validation of a clinically useful immunoassay. The Siman laboratory initiated a global, systematic analysis of protein release by degenerating neurons maintained in primary culture to establish a neurobiologic approach to identify new potential surrogate markers. The value of this neurobiologic approach is supported by the finding that proteins released preferentially by degenerating cultured neurons also are increased markedly in CSF and serum following TBI or cerebral ischemia in rodent experimental models (see following section). This neurobiologic approach can identify new markers and provide immunoassays for them with the potential to aid in diagnosis, prognosis, and therapeutic evaluation of acute brain damage.
The identification of a large number of novel candidate markers for acute brain injuries would foster development of a panel of markers that in turn can be expected to have diagnostic and prognostic sensitivity and specificity beyond that of individual markers. Indeed, clinical practices in oncology and cardiology have benefited considerably from the availability of multiple markers to classify tumors and detect and characterize myocardial infarction. In addition, a neurobiologic approach to identify surrogate markers for brain damage based on underlying proteolytic pathways for necrotic and apoptotic neurodegeneration would be valuable to define further the biochemical mechanisms for brain damage both in patients and preclinical experimental models.
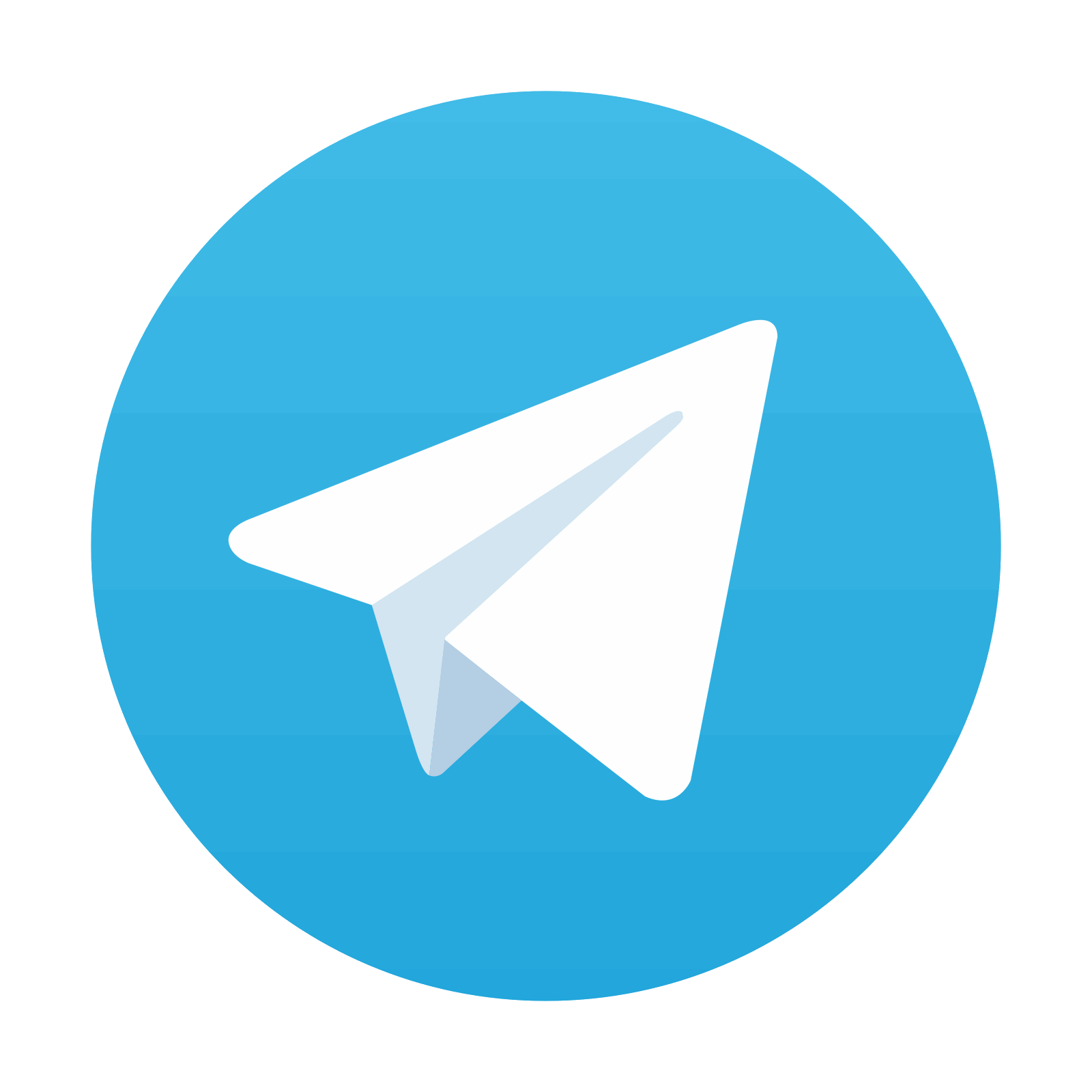
Stay updated, free articles. Join our Telegram channel

Full access? Get Clinical Tree
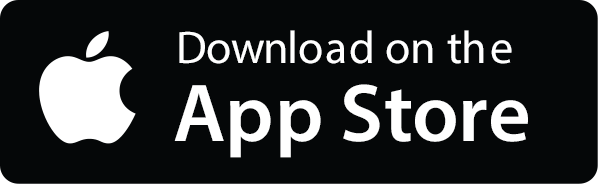
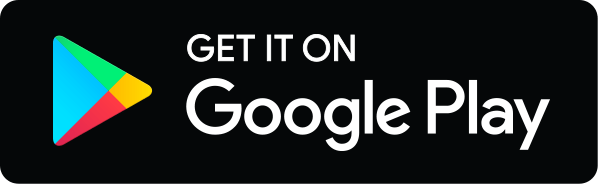