Biomechanics of Injury
David Fowler
The biomechanics of head injury is a complex interaction of energy and protective structures. Added to this is the change in anatomy during the human child’s development from the neonate to adult. The interplay of these multiple variants therefore creates a situation in which the forensic pathologist must integrate all the available information from the scene, autopsy, and subject matter expert (the neuropathologist). The ideal neuropathologist consultant is one who has extensive forensic and injury experience. This experience is critical in interfacing with the forensic pathologist to insure a thorough understanding of injury patterns and consistent terminology.
BASIC DEFINITION OF INJURY
Although injuries to the central nervous system (CNS) have their own issues and nuances, they follow the same general principles and mechanisms of injuries in other tissues and body systems. Therefore, a brief discussion of these general principles is appropriate.
An injury is a disruption in the normal anatomy of a structure. This anatomic change is caused by the application of energy to that structure at a rate that overcomes the structure’s ability to adapt.
The energy may be in multiple forms. Kinetic energy, the most common type of energy that causes injury, is the focus of this chapter.
FACTORS AFFECTING THE SEVERITY OF TISSUE DISRUPTION IN KINETIC ENERGY INJURIES
The biomechanical principles of head injury are similar to the host, agent, and vector principles of the disease transmission model. The host is the body or the portion of the body receiving the energy. The vector is the object carrying the energy (baseball bat, bullet, or vehicle), and the agent of injury is the energy.
Amount of Energy
The amount of energy dictates the potential for injury. Often the vector is a moving object, but other times, as in the case of falls, the host is carrying the energy and the vector (the ground) is stationary. At the site of impact, a portion of the kinetic energy of the host is transferred to a smaller area, which suffers tissue injury.
The Nature of the Transmitting Surface
All or a portion of the energy is transferred to the tissue. The proportion of transferred energy depends on the nature or consistency of the transmitting surface or vector.
A surface with a soft consistency will distort to conform somewhat to the shape of the impact site and spread the energy over a larger surface area, reducing the potential for injury. The opposite may be said of a hard surface. The CNS has the potential to be injured by energy that has passed through the overlying structures, in many cases without clinical evidence of injury to those structures.
Rate of Application of the Energy
Tissues have varying degrees of flexibility and elasticity and can resist and dissipate energy to some degree. These protective mechanisms are overpowered if the energy is applied rapidly. The firearm discharge is illustrative of this. A bullet fired from a firearm is a good example of transfer of energy from one object to another. Newton’s laws of physics apply.1 The bullet fired from a firearm has equal energy to the recoil of the firearm at the moment it leaves the muzzle. The firearm has a much greater weight than the bullet, hence greater inertia. The backward acceleration of the firearm (recoil) is therefore slower than the bullet. In the case of a handgun, the grip transfers the energy to the palm of the hand. The wrist, elbow, and shoulder joints flex to dissipate some of the energy. The result is no injury to the shooter. Newton’s third law applies, in that for every action there is an equal and opposite reaction, hence the bullet has the same energy as the recoiling firearm. The bullet, however, is small, lighter, and moving fast. As it strikes the head, the energy is focused rapidly on a small area, lacerating the scalp and fracturing the skull, allowing entry of the bullet into the cranial cavity. The absence of injury to the shooter illustrates the point that the same energy applied more slowly to a larger surface causes no injury.
Surface Area of Application
For a given amount of energy, the broader the area of its application, the less likely that the tissue will be injured. This is how a bulletproof vest works, by spreading and dissipating the energy of a projectile. The amount, surface area of application, and rate are interdependent to some extent. In head injuries, increasing amounts of energy
applied slowly over a broad area will initially allow the skull to remain intact, but at some point failure of the bone will occur as the strength and elasticity of the structure is exceeded, and the skull will fracture or be crushed. A rapidly applied and focused force will have a greater chance of causing penetration of the cranial cavity or fracturing of the skull.
applied slowly over a broad area will initially allow the skull to remain intact, but at some point failure of the bone will occur as the strength and elasticity of the structure is exceeded, and the skull will fracture or be crushed. A rapidly applied and focused force will have a greater chance of causing penetration of the cranial cavity or fracturing of the skull.
Anatomic Site of Application
Factors that affect the ability of tissues to adapt include the elasticity, flexibility, and amount of fibrous tissue, among others. Those structures containing air or gas (primarily gastrointestinal tract and lungs) can be compressed as the gas can move freely within the organ and/or be compressed by the pressure. Solid organs can tolerate far less compression without damage. The CNS, liver, and spleen are the prime examples. These more fragile organs are encased by or protected by other structures. In the case of the examples given above, the protection is provided by bone: specifically, vertebrae, skull, and ribs. The skull bones are of different thicknesses. The amount of energy to fracture or penetrate the thinner squamous portion of the temporal bone is less than the much more substantial occipital and parietal bones. The average thickness of the skull is 1 mm for a neonate, 4 mm for a young child, and 10 mm for an adult.2 The thin or absent diploe of the infant skull further increases the flexibility of the infant and young child’s skull and decreases the ability to resist injury. All tissues in the body have an ability to adapt to the applied energy to a greater or lesser amount. These so-called tissue factors vary according to the anatomic site. For example, the thickness of the skin and its resistance to injury varies considerably over the body, from the weight-bearing area of the soles of the feet to the palms of the hands to the delicate skin of the periorbital area. The diploic structure of the skull, with a thicker outer table and thinner inner table, also serves to dissipate energy by providing sequential separated layers of protection. To achieve similar impact resistance from a single layer would require a much thicker and heavier structure. The facial bones also can dissipate a significant amount of energy and reduce the potential for brain injury.
Skull fractures often follow the direction of application of the impact force, especially in high-energy motor vehicle-related impacts. The lateral impacts tend to cause fractures that cross toward the opposite side of the skull through the base of the skull. Forces may also cause the fracture at a distal site to the impact where the bone may be weaker, for example, ring fracture of the base of the skull or at a point of out-bending.3 Like any other solid material, forces cannot cross over a previous fracture line or unfused suture, thus allowing the pathologist to establish the sequence of fractures.
Direction of Energy
The direction of the applied energy is also a factor when looking at all of the above factors. The potential for anterior-posterior movement of the brain is greater than lateral movement; hence the lesser the potential for lateral falls to cause contrecoup injury. The majority of head and brain traumatic injuries entail movement of the head or an impact from a moving object, the exception being crush injuries. The movement of the head results from the application of a force. The type of the resulting movement depends on the relation of the vector of the force and the center of gravity of the head. A force for which the vector passes through the center of gravity of the head causes a translation movement, in which the motion is linear and there is little or no differential movement of adjacent brain tissues to create internal shear stresses. If the vector passes outside the center of gravity, it leads to a rotation movement. Rotation produces differential displacements of brain tissues; in other words, tissues that are more peripheral, such as the cortical mantle, will move faster than structures close to the axis of rotation (occipital condyles or base of the neck). These differential displacements result in shearing of the tissues, predominantly axons and blood vessels. The rotation of the brain relative to the skull can also overstretch and tear bridging veins, causing subdural hemorrhages.
Intervening Structures
The coverings of the brain, from the hair through the scalp layers and the two-layered structure of the skull, all contribute to dissipating directly applied energy and protecting the contents. The facial bones can also be considered to be intervening structures. The facial skeleton provides significant energy-absorbing capacity; therefore, contrecoup injuries are less commonly seen on the posterior aspects of the brain.
Body Movable or Static
The application of force to the head can be static or dynamic. Static loading occurs when the head is stationary and a loading force is applied. The skull fails and injury occurs to the cranial contents. An example of static application is a crush injury of the head, which occurs in situations where the fixed head is exposed to a large applied force, for example, partial ejection in a rollover motor vehicle crash. Far more common are injuries caused by dynamic forces, which can be applied by two different mechanisms: impulsive loading (shaking) and impact loading. Examples of impulsive loading (acceleration/deceleration) are the restrained driver of a vehicle that hits a tree or a subject who is violently shaken. In these examples, the force is applied to the torso or body and transmitted through the neck to the head. The resultant injuries include lesions of the neck and spinal cord and diffuse axonal and vascular injuries. Examples of impact loading are a fall that causes the head to be hit against the floor or a blow to the head with a blunt object. The development of brain injury is related to the changes in linear and angular velocity produced by the impact. It has been proposed that an adult head can tolerate up to 4, 480 N, or 1, 000 lb of force, without serious or fatal brain injury.4 The injuries secondary to impact are different from those of impulse loading: (1) lesions at the site of contact, or coup injuries (i.e., scalp bruising, skull fracture, brain contusion); and (2) lesions away from the site of contact caused by “wave propagation, ” also known as contrecoup injuries. Contrecoup injuries include the generation and release of skull deformations at sites away from the point of contact. An example of these skull changes is contrecoup fractures of the orbital roofs. Importantly, the structure and topography of the inside of the skull (i.e., ruggedness of the anterior and middle cranial fossae) are significant determinants of the localization of coup and contrecoup injuries. This has been demonstrated by experiments on Rhesus monkeys.5, 6
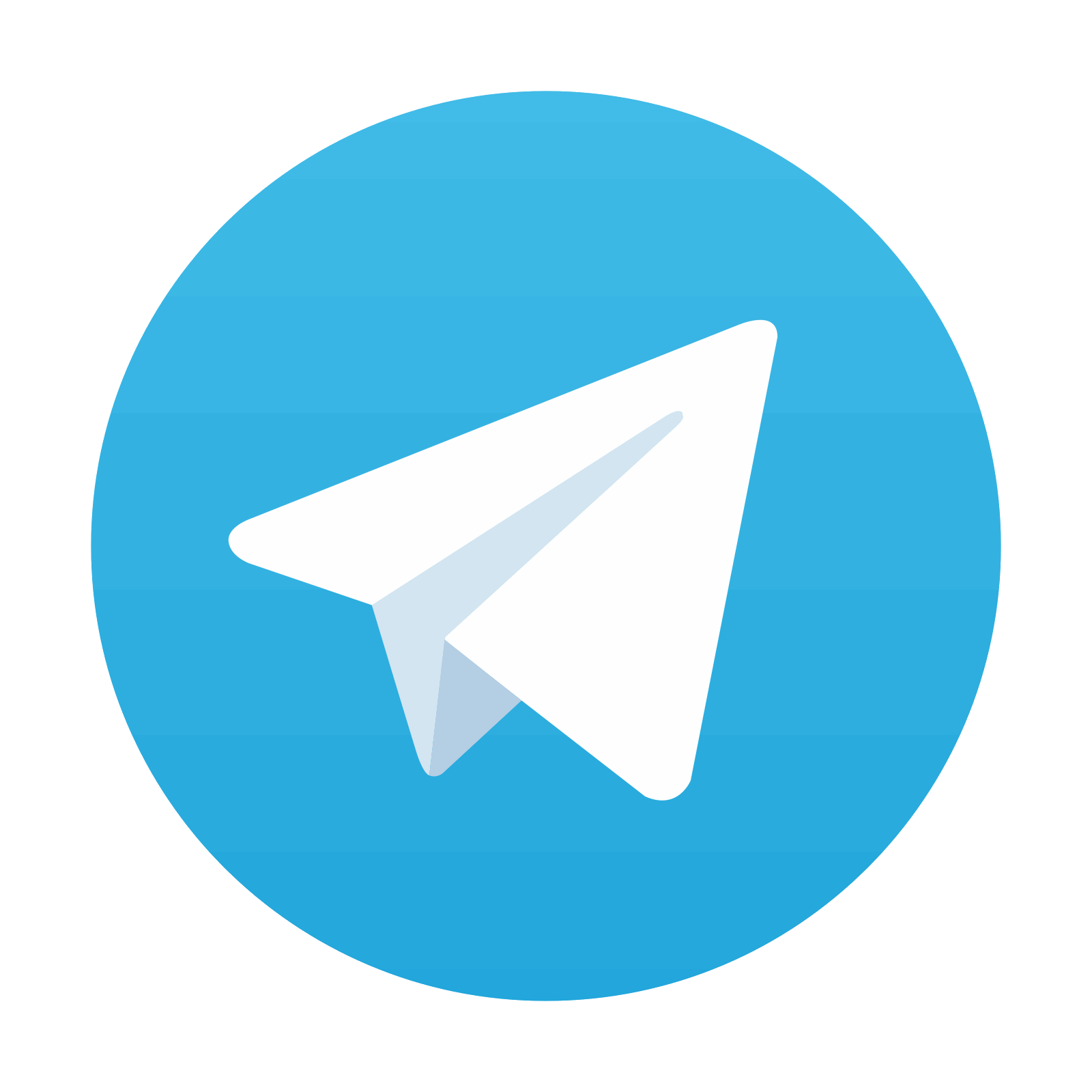
Stay updated, free articles. Join our Telegram channel

Full access? Get Clinical Tree
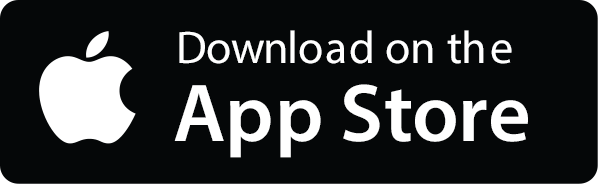
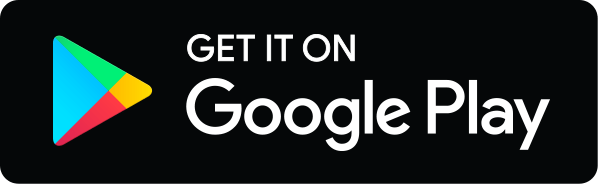