Chapter 28 Body Temperature, Sleep, and Hibernation
Introduction
Animals increase survival by residing in a safe sleeping site, and have used sleep to maximize energy savings by reducing body and brain energy consumption and to conduct a variety of recuperative processes.1,2 Knowledge about thermophysiology and its relation to sleep leads to the hope that temperature related interventions can alleviate sleep disturbances and be helpful to cure certain aspects of sleep and alertness problems in the general population.
A vast amount of knowledge is found in the literature on the variability in rest–sleep states and on thermophysiology across the animal kingdom.1,2 In order to limit the scope of this chapter, only findings from humans, rats, ground squirrels, and hamsters have been reviewed.
Circadian Regulation of Core Body Temperature
Fifty years ago, Aschoff3 showed that the human body consists of two thermophysiological compartments, the heat producing, homeothermic core, and the heat-loss regulating, poikilothermic shell. The size of the latter is largely dependent on environmental temperature. In a warm environment the shell is small, in a cool environment large, and thus acts as a buffer to protect the core from dangerous cooling. All peripheral tissues such as fat, skin, and in particular skeletal muscles of the legs and arms can contribute substantially to the size of the shell, provided that peripheral blood flow is low. Therefore, rates of blood flow through muscles and skin are the main determinants of shell size variability and hence of peripheral insulation. The distal skin regions, in particular fingers and toes, are our main thermoeffectors to lose body heat as they possess the physical and physiological properties to best serve the function of heat loss. They have ideal surface shapes (round, small radius) for good heat transfer to the environment—the surface-to-volume coefficient increases from proximal to distal skin sites. Therefore, the distal skin temperatures provide a good measure of the shell size.
CBT comprises the temperature of the brain and the abdominal cavity, including inner organs (e.g., liver, heart, kidney).3 In most placental mammals CBT is regulated around 37° C, whereas the brain is the main target for homeothermy allowing control of all behavioral and physiological processes over a broad environmental temperature range. A detailed description of the thermoregulatory system can be found elsewhere.4
CBT is regulated between thermoeffector thresholds, which are subject to circadian oscillations.5 Circadian rhythms in mammals are generated by the self-sustaining central pacemaker localized in the suprachiasmatic nuclei (SCN) of the hypothalamus and are usually entrained to the 24-hour solar day mainly by the synchronizer light.6 A rostral projection from the SCN to the preoptic-anterior-hypothalamus (POAH) conveys the circadian signal to the thermoregulatory system.6 The regulation of CBT results from the concerted action of the homeostatic and circadian processes. In humans, the daily decline of CBT in the evening results from a regulated decline in the thermoregulatory thresholds of heat production and heat loss; the inverse happens in the morning. When heat production surpasses heat loss, body heat content increases, and vice versa. Approximately 70% to 90% of body heat content is located in the body core, depending on environmental temperature; therefore, changes in CBT reflect to a great extent changes in body heat content. Heat production and heat loss are modified by activities such as muscular exertion and fluid and food intake that are not randomly distributed over the circadian cycle. These behaviors induce so-called masking effects, and differentially modify the endogenous rhythm of CBT.7
In order to disentangle circadian from masking effects of an overt diurnal pattern, the constant routine (CR) protocol has been developed in humans.8 With this protocol it was shown that the time course of heat production precedes heat loss, and CBT varies as an intermediate resultant.8 Heat production and heat loss are not only separated in time but also in space in the body.3 Under resting conditions, about 70% of heat production depends on the metabolic activity of inner organs, whereas body heat loss is initiated via heat redistribution from the core to the shell through blood flow to the distal skin regions.3 Thermoregulatory distal skin blood flow is regulated by the autonomic nervous system via constriction or dilatation of arteriovenous anastomoses. These are shunts between arterioles and venules, exclusively found in distal skin regions (e.g., toes, fingers).3 When they are open, warm blood flows rapidly and directly from arterioles to the dermal venous plexus enabling an efficient heat exchange from the core to the distal skin. The exact neural process by which this regulation is achieved is still a matter of debate9; however, sympathetic nerve activity seems to be crucial for peripheral vasoconstriction. The endogenous time course of distal skin temperatures (hands and feet), measured during a CR, exhibits an inverse circadian rhythm in comparison with CBT, whereas the former is phase advanced by about 100 minutes8 (i.e., in the evening distal skin temperatures rise before CBT declines). The amplitude of distal skin temperatures rhythm is about three times larger than those of CBT.8,10 In contrast, temperatures of proximal skin regions (e.g., thigh, infraclavicular region, stomach, forehead) follow a parallel change to CBT and the amplitudes are of similar magnitude.8,10 This inverse relation between distal and proximal temperature rhythms reflects the differences in thermophysiological regulatory mechanisms as described above.3 The distal minus proximal skin temperature gradient (DPG) provides therefore a selective measure of distal skin blood flow, and hence body heat loss via the extremities.3
Nocturnal secretion of the pineal hormone melatonin, which is under control of the SCN, plays a crucial role in the endogenous downregulation of CBT in the evening.11 Administration of melatonin in the afternoon, when endogenous melatonin levels are low, provokes exactly the same thermophysiological effects as observed naturally in the evening.11 Whether melatonin induces distal vasodilatation in humans by acting directly on blood vessels receptors or indirectly via modulation of sympathetic nerve activity, or both remains to be determined.11 In addition, both subjective ratings of sleepiness and level of activity in the electroencephalographic (EEG) theta and alpha range as an objective outcome of sleepiness–wake state, are increased.11 Moreover, it is noteworthy that the rise in melatonin secretion in the evening belongs to a well-orchestrated circadian physiological regulation controlled by the SCN, which in turn downregulates CBT, increases sleepiness, and promotes sleep.
Relationship Between the Sleep Regulatory and the Thermoregulatory System
The most evident explanation whether and why the sleep regulatory and thermoregulatory systems are interrelated is a teleological one: sleep is for energy conservation.2,12,13 All species sleep or rest when their energy expenditure is low. Rest or quiet wakefulness, is a prerequisite for sleep in all species.1,12,13 These observations represent the starting point of all energetic explanations why we sleep. Human sleep evolved from ancestral sleep and it is quite possible that earlier forms of sleep were linked to energy conservation in ancestors with a smaller body size.
There are two mechanisms that enable sleep to conserve energy. One is that sleep reduces energy expenditure indirectly by reducing activity. This mechanism would also be active when animals only exhibit quiet wakefulness. Alternatively sleep induces an additional decline in energy expenditure below that accomplished by quiet wakefulness by a change in physiology. Human sleep is only accompanied by a modest decline in energy expenditure below the level of quiet waking.13–15 However, energy conservation may be particularly important in small animals and infants.2,13,16 Their high surface-to-body-mass ratio is ideal to dissipate heat and renders energy conservation achieved by sleep highly adaptive.13 When body size increases and sensory-motor systems mature in the course of infant development, a parallel decrease in sleep time occurs.2,16
Co-variation of Sleep and Thermophysiological Variables
Baseline Conditions
In order to compare the sleep and thermoregulatory systems it is crucial to separate circadian from masking components of an overt diurnal pattern. This is much easier to accomplish in humans than in animals. In spite of this advantage, the most neglected factor in human research is the so-called lying down effect. A change from standing to supine body position induces redistribution of heat from the core to the periphery, thereby increasing skin temperatures, decreasing CBT, and in parallel increasing sleepiness.17 This effect lasts about 1 to 2 hours,17 and significantly confounds the endogenous time course of CBT in a classical sleep recording protocol where lying down occurs about 0.5 hours before lights off. The temporal relationship between thermophysiological variables, heart rate, subjective ratings of sleepiness and salivary melatonin secretion under CR conditions before habitual bedtime and for the following sleep episode is summarized in Figure 28-1. The only thing that changed during this protocol was that the low intensity lights were switched off with the implicit permission to fall asleep. Before lights off the previously described endogenous pattern of CBT down regulation is visible. In the evening, heart rate (an indirect measure of intrasubject variation of heat production) declined first, followed by heat loss, and finally by a decrease in CBT. Subjective ratings of sleepiness increased in parallel with distal proximal gradient (DPG) and salivary melatonin levels. The proximal skin temperature exhibited a similar pattern as CBT. Immediately after lights off, and before sleep stage 2, distal and proximal skin temperature increased and heart rate declined.18 In addition, an increase in sweating rate is often observed, depending on CBT.19
The typical increase in distal skin temperature, as shown in Figure 28-1, is caused by heat redistribution from the core to the shell. Similar findings at sleep onset have been described in the lower leg.20 However, CBT exhibited only a slight but significant increase in the rate of change after lights off,3,21 leading to approximately 0.3° C lower CBT values during sleep compared to quiet wakefulness.22 In contrast to the fast changes in skin temperature, the decline in CBT is slow, which can be explained by the reduced cardiac output during sleep initiation impeding a faster heat loss during the sleep episode, under thermoneutral conditions.18 The magnitude of the decrease in CBT is negatively correlated with environmental temperature.23 A DPG of 0° C indicates that during sleep, the thermoregulatory shell has disappeared, therefore resembling a state similar to that of the human body in awake state in a warm environment (e.g., 35° C).3 Heat redistribution from the core to the shell is completed within approximately 1 hour after lights off. Such a completely relaxed one-compartment body is prone to a fast cooling when sleep occurs in a cool environment. In normal sleep environment, CBT is protected during sleep because humans and animals try to occupy a sleep berth in a comfortable thermal environment.13 When sleep is initiated outside the natural temporal niche by taking an afternoon nap, similar thermophysiological changes occur right after lights off and before the initiation of sleep stage 2.24 There are subjects, mostly women, exhibiting a proneness to cold hands and feet and, therefore, to a large shell.25,26 These subjects show a significant co-morbidity for prolonged sleep stage 2 onset latency (SOL2).25,26 In fact, it has been shown that subjects with sleep onset insomnia respond to a mild heating with reduced thermoregulatory heat loss from their fingers.27
After a sleep episode, transition to waking is accompanied by an inverse thermophysiological pattern.24 This period is named sleep inertia; after awakening it takes a certain time interval to recover all physiological and cognitive functions.24 During that time, a similar but inverse time course in distal vasoconstriction is observed.24 It is noteworthy and of clinical relevance that similar thermophysiological effects as seen during sleep initiation can be observed after administration of benzodiazepines28 and with certain relaxation techniques like yoga, autosuggestion of warmth, autogenic training, and meditation without falling asleep.3,28–30 These techniques induce a withdrawal in muscular and cutaneous sympathetic nerve activity, which leads to increased distal skin temperature and to a reduction in heart rate, energy expenditure, and CBT.28,29 Therefore, distal vasodilatation followed by a drop in CBT appears to be a thermophysiological event, which is primarily related to relaxation occurring before sleep onset.31
Studies carried out with humans to describe changes in thermophysiological variables show that changes in CBT, proximal and distal skin temperature related to the NREM–REM sleep cycle are very small.32,33 Heart rate is clearly increased shortly before and during REM sleep, relative to NREM sleep, which is, however, reflected only in a minor increase in energy expenditure during REM sleep.15 Extensive studies on thermophysiological alterations regarding the NREM–REM sleep cycle, concluded that changes in brain heat production are practically not relevant for changes in brain temperature.34 To our knowledge, only one human study recorded brain temperature together with sleep-EEG data, but no significant systematic changes regarding the NREM–REM sleep cycle were found.35
One of the advantages of animal research is the parallel recording of body and brain temperature. In many small mammals (rabbit, rat, Djungarian hamster) NREM sleep is associated with a decrease in brain temperature, whereas REM sleep and waking are associated with an increase in brain temperature (Fig. 28-2).36,37
In an elegant study performed with rats it was shown that heat is redistributed across the body when vigilance states change.38 At the initiation of NREM sleep, the brain and intraperitoneal temperature decreased whereas the tail skin temperature increased. The opposite occurred at the transition from NREM sleep to awake. At transitions from NREM sleep to REM sleep, brain temperature rose slightly, whereas intraperitoneal and tail temperatures did not change. These data are in accordance with those obtained in humans. Heat is redistributed from the core to the shell at the onset of sleep. Humans thermoregulate by vasodilatation and vasoconstriction of blood vessels within the skin of extremities; in rats similar changes are observed in the tail. The main difference lies in the timing of the redistribution of heat relative to the onset of sleep and waking. In humans changes are visible several hours before sleep onset; in the rat the same changes occur at the immediate onset of sleep. This difference is probably related to the smaller body size and to the ultradian sleep–wake pattern in the rat that renders a time-lag of several hours to be nonfunctional.
Applying a CR in rodents is not possible. However, on the basis of the relationship between brain temperature and vigilance states, it was possible to subtract the influence of vigilance state changes on brain temperature, rendering a mathematical CR.39 This study concluded that, in the rat, approximately 90% of the variance in brain temperature is caused by changes in vigilance. A recent study confirmed that vigilance state–related changes in brain temperature are independent of the functioning of the circadian clock because they remained intact after removing the SCN.40
Taken together, there are robust thermoregulatory effects induced by lying down and the relaxing sleep behavior, however, the NREM–REM sleep cycle seems to have minor thermoregulatory function in humans. The thermoregulatory mechanisms, which are active during the wake–sleep transition, redistribute heat from the core to the shell and induce a decline in heart rate, energy expenditure,14,15 and CBT. Relaxing behavior before sleep belongs inseparably to sleep; therefore, these data are not in contradiction with the energy conservation hypothesis of sleep. The accompanying thermoregulatory effects in humans may be a remnant of their evolutionary past.
Changed Circadian Conditions
It has been observed that subjects living under normal conditions choose their bedtime (lights off) at the maximal rate of decrease in their CBT rhythm.41 However, when subjects are living on self-selected sleep–wake schedules in a time-free environment, bedtime is phase delayed close to the CBT minimum, which is an indication that the sleep–wake cycle and the circadian rhythm of CBT are separate but usually entrained (synchronized) oscillatory systems.42 Unfortunately, neither direct nor indirect measurements of heat loss and heat production were carried out in parallel in these studies. Therefore it is possible that CBT is not the crucial variable for sleep induction, but rather one of its determinants, that is, heat loss. Because heat loss seems to be closely linked to sleep initiation, it may be speculated that the circadian rhythm of heat loss is phase delayed under free-run conditions. The duration of sleep episodes was maximal when initiated at the time when CBT reaches its maximum and, at the opposite, minimal sleep lengths occurred when sleep was initiated during the rising phase of the CBT rhythm.16
There is also a reproducible and robust circadian rhythm in sleep onset latency to sleep stage 2 (SOL2), which is closely related to the circadian CBT rhythm and thermoregulatory effects as described previously.43 In forced desynchrony studies (i.e., living on a scheduled 28-hour day including a 9.33- to 18.66-hour sleep–wake cycle) it was shown that SOL2 is longest at the circadian phase where CBT reaches its maximum, that is, 1.5 hours before CBT starts to decline and melatonin secretion rises.44 At this circadian phase, named the “wake-maintenance zone,”45 inner heat conduction is lowest as indicated by largest difference between CBT and distal skin temperature, as well by largest negative DPG values. Thereafter, SOL2 declines rapidly and is minimal around the time when CBT reaches its circadian trough, when inner heat conduction is largest (distal skin temperature is highest and the difference between CBT and distal skin temperature is lowest). However, it remains to be determined whether thermal interventions, like lower leg warming, at the wake-maintenance zone are successful to reduce SOL2, as melatonin administration was shown to do.46
Under most experimental conditions, REM sleep propensity exhibits a strong circadian pattern with a peak approximately 1 to 2 hours after CBT has reached its circadian minimum.44 The circadian rhythm of REM sleep propensity is closely phase locked with the circadian rhythm of CBT with a phase lag of 1 to 2 hours.
Intervention Studies in Humans
Effects of thermal interventions (heating or cooling) on sleep are not easy to investigate. Thermal intervention, either applied passively or actively by physical exercise, induces significant changes in skin temperatures and CBT.14,16,47,48 Not only is the intensity of a thermal intervention crucial, but also the skin region selected and the time of application. During sleep only passive thermal loads can be applied. It has been shown that sleep reduces the thresholds and gains of the autonomic temperature defense mechanisms and expands the inter-threshold zone (the temperature range for activation of metabolic heat production or evaporative heat loss).16,48,49 These threshold changes are modest in slow-wave sleep (SWS), but much stronger in REM sleep.16,48 As a consequence, CBT and skin temperatures are more sensitive to changes in environmental temperature. Maximal total sleep time (TST) is found in the thermoneutral zone (the range of ambient temperature at which temperature regulation is achieved solely by vasomotor responses), whereas REM sleep is more vulnerable to thermal interventions than is SWS.16,48 Too powerful of thermal interventions induce arousals and awakenings, which in turn can change thermoregulatory effects, for example, elevating CBT.48 When a thermal load is given repeatedly, the thermoregulatory system can adapt and the effects on sleep are changed; for example, the arousing effects are reduced. Aborigines in the Central Australian desert and nomadic Lapps in Arctic Finland were experiencing comparable degrees of cold exposure during the night and both showed lower thermoregulatory thresholds for shivering before modern technology arrived.50,51 As a consequence, CBT was more reduced during sleep and undisturbed sleep occurred at a lower environmental temperature. Many modalities of thermal interventions on sleep are understudied. In addition, the impact of thermal interventions on the sleep of normal and sleep-disturbed subjects may differ.
Changing Temperatures
It has been shown that ambient temperature, especially in combination with high humidity, is of importance for both quantity and quality of sleep.48 When sleep occurs in warm environmental temperature (between 31° C and 38° C) duration of wakefulness increases and, at the opposite the duration of REM sleep and NREM sleep decreases.14,16,47,48 Also cold exposure (21° C) induced more awakenings, less time in sleep stage 2 and less TST; however, without affecting duration of other sleep stages, marked thermoregulatory effects were induced under such manipulations.14 The decrease in CBT observed during the night episode was larger at 21° C compared to a thermoneutral 29° C condition. During REM sleep, forehead temperature and oxygen consumption increased and feet temperature decreased in comparison to SWS with cold exposure. Therefore, cold-exposed humans may not exhibit a complete inhibition of thermoregulation during REM sleep as it has been observed in small mammals.
When during sleep the ambient temperature is gradually decreased, an earlier CBT nadir and an advanced peak for REM sleep propensity was obtained.52 It was also shown that duration of sleep stage 4 is increased when the normal nocturnal decrease of CBT is augmented by a constant and mild reduction in ambient temperature, in spite of decreased sleep efficiency.52 Similarly, after a reduction in ambient temperature by 2° C during sleep, it was observed that the increase in SWS occurred with the rise in slow-wave activity (SWA; EEG power density between ~1 to 4 Hz) without any change in sleep efficiency and reduced amount of REM sleep.53 The thermal manipulation reduced not only leg skin temperature but also CBT and heart rate. Taken together, the augmentation of heat loss leading to reduced CBT during sleep seems to be the crucial variable for increased SWS.
In humans, body heat content and hence CBT can also be effectively manipulated by body immersion in warm or cold baths. For instance, due to rapid conductive heat loss in a cold bath, CBT falls faster compared with that observed in air at the same temperature. Rewarming of the cool shell after cool bathing leads to a characteristic after-drop in CBT.54 Several studies showed effects of positive heat load on sleep;14,47,48 however, no study examined effects on sleep after a cold bath. In general, passive body heating (40° to 43° C for 30 to 90 minutes; CBT increase: 1.4° to 2.6° C) has a positive effect on many aspects of sleep in healthy young adults and in older and sleep-disturbed subjects. It was found that warm bathing in the evening shortened sleep onset latency, enhanced SWS duration, and sometimes reduced REM sleep duration. The increase in SWS, however, is not dependent on a reduction in REM sleep. Bathing performed in the morning or early afternoon had no effect on sleep architecture.14,47 In principle, actual levels of CBT at sleep onset or the decline in CBT afterward could be related to the amount of SWS after warm bathing.14,47 Additionally, a phase delay of the CBT nadir during the night sleep episode has been described after evening hot bathing, which correlates with increased SWS.55 All these CBT characteristics could be intercorrelated (i.e., directly after a positive heat load the velocity of CBT decline is larger, the CBT level is elevated before sleep onset and the overt CBT nadir during sleep may be delayed). However, the phase shifting effects of passive heat loads in humans have not been studied systematically. Variables other than CBT, for instance skin temperature, may play a role. The available findings are inconsistent due to the diversity in study designs and methodology and the low statistical power of many studies. In one study, hot full-body bathing and hot foot bathing were applied 35 minutes before lights off.56 Only during full-body bathing did CBT increase (by about 1° C); however, both conditions increased mean skin temperatures and reduced sleep onset latency and movement during sleep. These findings indicate that elevated skin temperature is crucial for a rapid onset of sleep and not changes in CBT. Older sleep-disturbed subjects respond to hot foot bathing with slightly reduced sleep onset latency (SOL) to sleep stage 1 and significantly decreased wakefulness in the second NREM sleep period.57 In these older subjects, not only DPG but also CBT was elevated after hot foot bathing during the first hour of sleep.
Of clinical relevance is the data describing that the use of electric heat blankets throughout the night reduced REMS duration and TST,58 suggesting that the heat load exerted through the blanket is a too-strong thermal intervention that disturbs sleep rather than supports sleep. In a series of experiments, the effects of tiny changes in skin temperatures of only 0.4° to 2° C within the thermal comfort zone, without significantly altering CBT, were investigated on several sleep parameters.59 It was demonstrated that intermittent elevation in skin temperature during the sleep episode suppresses nocturnal awakenings and triggers shifts to deeper sleep in young and older healthy subjects and in insomniac patients.59 Whole-night studies are needed to confirm that the sleep-depth enhancing effect of mild skin warming can indeed be sustained. Nevertheless, these findings support the importance of skin temperatures, primarily proximal skin temperatures (including the trunk) but also more distal skin regions, such as legs and arms, for these effects. Other studies revealed that subtle skin temperature warming was associated with a faster onset of sleep in young and older subjects and in older insomniac and narcoleptic patients.59–61 At present stage, it cannot be concluded which thermophysiological correlate represents the causal factor to increase SWS and reduce SOL. Nevertheless heat load before sleep seems to increase the propensity of SWS.
Intense exercise is a manipulation that can also raise CBT by 2° C or more. Subsequently, CBT declines as a result of the thermoregulatory heat loss drive via increased vasodilatation and sweating. A number of reproducible exercise-induced changes on sleep have been identified after exercise in the evening: shortened SOL, increased TST and SWS, longer REM sleep onset latency and less REM sleep.62,63 Exercise exhibits negative effects on sleep when performed close to the start of sleep—the optimal temporal positioning of physical activity is thought to be between 4 and 8 hours before bedtime.63 Chronic exercise studies have not provided much stringent evidence of a sleep promoting effect. Conversely, with reduced exercise load in trained athletes, SWS and REM sleep onset latency were reduced, and REM sleep duration and SOL were increased.64 Taken together, after intense exercise, sleep appears to commence faster and is deeper.
In conclusion, warm distal skin temperatures either induced by endogenous circadian heat loss regulation in the evening, homeostatic downregulation of CBT after passive and active heat load, or selective skin warming predisposes a rapid onset of sleep. More sophisticated studies with respect to skin regions are necessary to show which areas (e.g., shoulder, stomach, legs, hands, or feet) should be warmed to exhibit the strongest effects on sleep initiation and sleep architecture. The increase in skin temperatures could be the causal factor for the acceleration of sleep onset and the increase of SWS. Further studies must investigate the optimal time-interval between thermal intervention and bedtime and which physiological mechanisms are involved in the observed effects. It is possible that thermal afferents provide a signal for the sleep-inducing brain regions in the hypothalamus.28,65
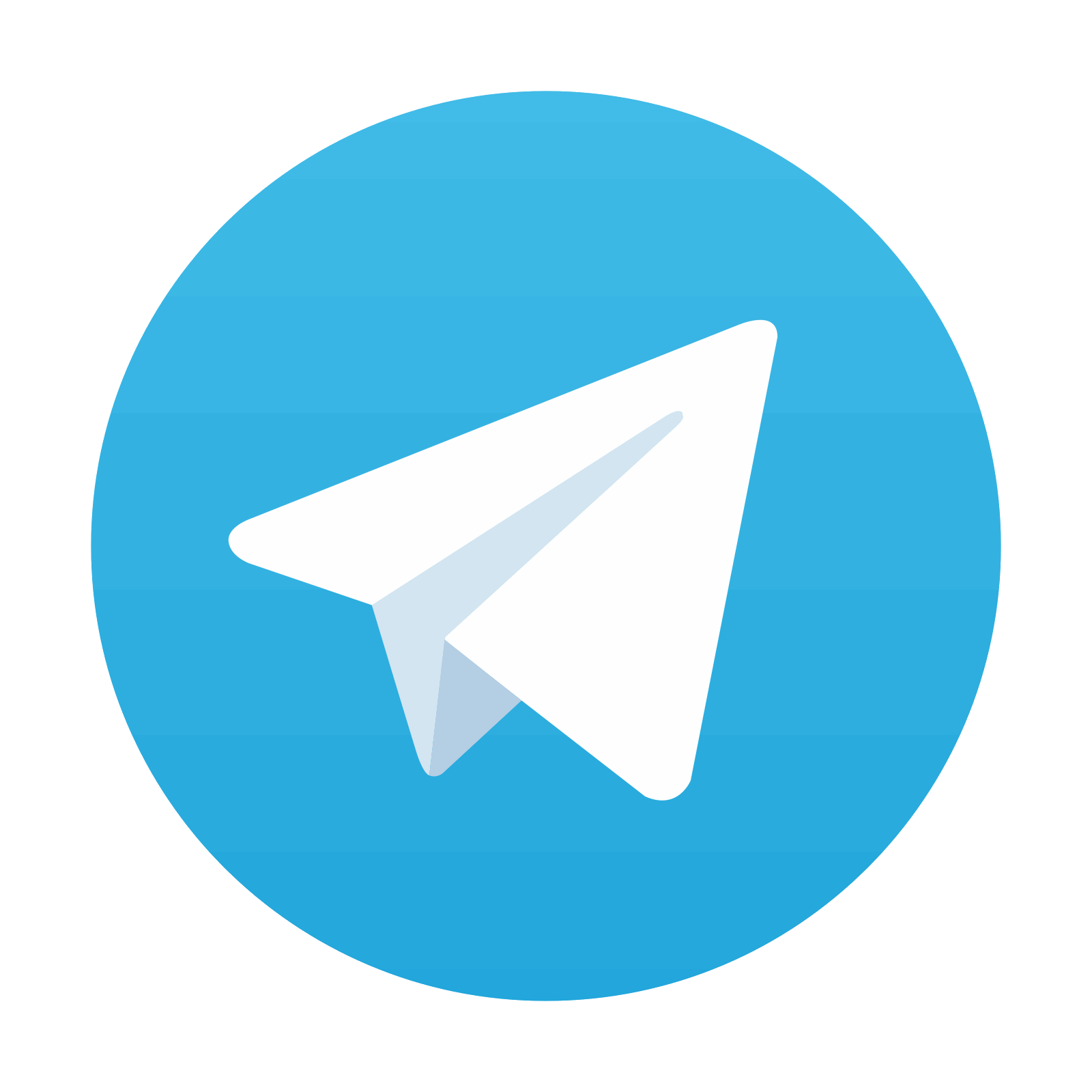
Stay updated, free articles. Join our Telegram channel

Full access? Get Clinical Tree
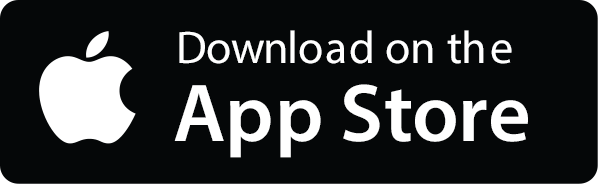
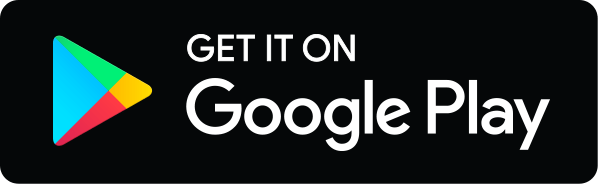