Introduction
Neuromonitoring in the intensive care unit (ICU) is undertaken for several acute neurologic conditions in children. Traumatic brain injury (TBI) constitutes the most common indication for neuromonitoring because injury is the leading cause of death in children over the age of 1 year, and those who sustain TBI have the highest chance of dying or being permanently disabled. Early treatment to prevent secondary insults may avoid as much as 30% of pediatric TBI deaths. Therefore, monitoring for these potential secondary insults is the foundation of management for children. Importantly, experimental studies demonstrate that neuromonitoring findings, including intracranial pressure (ICP), brain oxygen, and microdialysis correlate with neuropathy of animal models of pediatric TBI. The aim of this chapter is not to provide a comprehensive summary of monitoring options, but rather to highlight some differences between adults and children with respect to neuromonitoring. In addition, the reader is reminded that neuromonitoring may be valuable in children and adolescents with nontraumatic coma (e.g., meningitis, hepatic encephalopathy, stroke, near drowning, and hypoxic-ischemic injury).
Children and Adults Are Different
Potential secondary insults in children are similar to those in adults; however, children have different physical dimensions and physiologic responses. They are rapidly growing and developing, and normal ranges and thresholds for treatment not only are different from adults, but also vary between age groups. For these reasons, and because of the smaller research base, the best evidence-based recommendations for specific aspects of clinical management in pediatric TBI are still mostly set at the level of options.
Glasgow Coma Scale
The Glasgow Coma Scale (GCS) is the standard method of assessing and monitoring the level of consciousness in pediatric TBI. In children the utility of the GCS is limited in younger patients; therefore, modifications of the GCS such as the Pediatric Coma Scale and the Children’s Coma Scale are used. However, the GCS as a monitoring tool is limited by prehospital and ICU sedation and because children with higher GCS may deteriorate suddenly. Methods of assessing initial clinical severity, such as the GCS and acute illness scores, do not predict which patients are at risk for all types of secondary injury.
Prehospital Hypotension and Hypoxia
Polytrauma is common in children with severe TBI, and the addition of extracranial injury increases the risk of hypotension and hypoxia. Hypoxia and hypotension occur in 31% to 68% of children who sustain severe TBI and are associated with poor outcome, especially hypotension. Although the diagnosis of hypoxia in children is standard, and consistent with that in adults, the definition of hypotension varies. Systolic blood pressure (SBP) is commonly used to define hypotension, either as SBP less than 90 mm Hg or less than the fifth percentile for age, but these approaches have limitations. First, mean arterial pressure (MAP) is more appropriate for determining likely cerebral perfusion pressure (CPP). Second, height influences the normal range of blood pressure (BP) in children, but is rarely considered. Height is related to BP independently of age, so there are significant differences across height-for-age percentile blood pressure ranges.
Cerebral Blood Flow
There are important differences in cerebral blood flow (CBF) values between adults and children in health and disease. Specifically, the role of hyperemia as the predominant cause of diffuse swelling observed after TBI in children has been debated. When CBF values in children are compared with mean values for age, the incidence of true hyperemia appears to be much lower than previously believed. CBF values tend to increase from the lowest values at birth to a peak at age 3 to 5 years and then decrease to adult levels. Therefore, when interpreting high CBF values in children with TBI (high and low), age-specific ranges must be considered. Low CBF is common early after injury and is associated with worse outcome; however, data on practical CBF monitoring for children in critical care are limited. Ideally, absolute CBF values should be interpreted with metabolic demand and flow-metabolism coupling.
Intracranial Pressure: Indications, Methods, and Thresholds
Indications for intracranial pressure (ICP) monitoring in children vary among different institutions. Current recommendations support ICP monitoring for children with severe TBI, regardless of whether fontanels and sutures are open. Still, in the absence of randomized controlled trials, some question whether ICP monitoring is beneficial in pediatric TBI ; the reasons for this are complex. In the United Kingdom only about 60% of children presenting with severe TBI undergo ICP monitoring, with large among-center variation, and many patients receive ICP-targeted therapy without an ICP monitor in situ. But adherence to published guidelines appears to be increasing. Indications for ICP monitoring in children are based on less evidence than in adults because of the paucity of studies. Because computed tomography (CT) signs cannot reliably exclude intracranial hypertension, it is recommended to monitor all patients with severe TBI who are not likely to be extubated within 12 hours.
Standard methods for ICP monitoring in adults are also used in children; intraparenchymal devices are most commonly used and complications are rare. A ventriculostomy can be useful in some patients but is less commonly used, most likely because of difficulties in cannulating small ventricles in the typical child with diffuse swelling. For practical purposes the bolt system for intracranial catheters in pediatrics is usually suitable even in younger children, but the very young may require a formal burr hole and subgaleal tunneling if the skull is particularly thin.
In children the definition of elevated ICP is less clear because of changing physiologic norms with age, the different pressure-volume relationship of the brain in children, and uncertainty about whether the autoregulatory curve in children is similar to that of adults. Lower thresholds than those recommended for treatment in adults are often used for younger children, but there are no reliable data to support this. The treatment threshold of 20 mm Hg is recommended at the level of an option for children. If the anatomic characteristics of the brain that determine the point at which a pediatric brain undergoes herniation are similar to that of an adult brain, and the autoregulatory curve is similar, then targeting a similar threshold appears sensible, so long as an adequate CPP is maintained. This ICP threshold for treatment is supported by the findings in children of an inverse relationship between CBF and ICP greater than 20 mm Hg and significant changes in the pressure-volume index when ICP is greater than 20 mm Hg. However, the relationship between ICP and CBF or oxygenation of the brain is complex. Several pathophysiologic mechanisms may underlie an increase in ICP in individual children, including classic cerebral edema, seizures, impaired pressure autoregulation, hyperemia (uncoupled flow-metabolism coupling), increased CO 2 , and so on. Therefore, the precise relationship between ICP and perfusion of the brain depends not only on the effect of ICP on perfusion, but also on the reason for the increased ICP. In the author’s experience, ICP affects oxygenation of the brain at different levels, depending on the age of the patient, the absolute ICP value, and the underlying cause of the increased ICP. Although it is important to treat elevated ICP, additional data are required to guide sound clinical decisions. Therefore, a multimodal approach has particular value.
Cerebral Perfusion Pressure
Low CPP is known to be associated with poor outcome; however, this may be a surrogate marker for high ICP. However, some argue that absolute ICP values are of lesser importance if CPP is adequate. Therefore, as in adults, the merits of ICP-targeted treatment versus CPP-targeted treatment are also debated in children. Higher CPP targets in adult TBI have been recommended but may increase the risk of acute respiratory distress syndrome, and if autoregulation is impaired it may increase ICP and tissue edema. The Lund approach using brain volume regulation and microdialysis monitoring to accept lower CPP levels also has been described in children but is not without controversy and risks.
It has not been established that active modulation of CPP, beyond avoiding hypotension, benefits pediatric TBI patients, so there is little agreement on what constitutes an appropriate CPP target for age. Chambers et al. recommended CPP thresholds of 48, 54, and 58 for age-groups 2 through 6, 7 through 10, and 11 through 15 years, respectively, and 45 mm Hg overall. Prabhakaran et al. examined ICP- and CPP-targeted approaches but reached no definitive conclusion. Vavilala et al. found that all children who had an admission SBP less than 90 mm Hg had a poor outcome, but that the 75th percentile of SBP for age was a better predictor for outcome. Jones et al. and Hackbarth et al. recommended CPP greater than 50 to 60 mm Hg, but Downard et al. found that CPP greater than 50 mm Hg conferred no survival benefit and argued that benefits of CPP elevation may simply be a proxy for the avoidance of hypotension.
None of the these studies considered height for age in the evaluation of MAP or CPP. Furthermore, all recommendations for specific targets may not be appropriate given that impaired cerebral autoregulation is common in children with severe TBI. Nevertheless, current recommendations for pediatric TBI support the avoidance of CPP less than 40 mm Hg in all children and targeting CPP greater than 50 mm Hg as an option. These have no clear recommendations for an age-related approach. It is likely that a single CPP value is not appropriate for all patients given differences in age and metabolic needs, the tissue pressure effect of increased ICP (rather than just the CPP-lowering effect thereof), the status of pressure autoregulation, and the variable coupling between metabolism and CBF.
Brain Tissue Oxygen
Several studies have examined the relationship between brain tissue oxygen tension (P bt O 2 ) and outcome in adults, but few in children. Narotam et al. ( n = 16) found that no children with normal initial P bt O 2 died. Stiefel et al. ( n = 6) found P bt O 2 was significantly lower if ICP was greater than 20 mm Hg and CPP was less than 40 mm Hg. Figaji et al. ( n = 52) found that low P bt O 2 was an independent predictor of poor outcome. Poor outcome was linked to the depth and duration of brain hypoxia, especially when P bt O 2 was less than 10 mm Hg. In addition, episodes of critical brain hypoxia (P bt O 2 <10 mm Hg) occurred in almost one third of patients despite recommended treatment targets being met for ICP, CPP, arterial oxygenation, and hemoglobin concentration. These episodes appear to be not only outcome predictors but also a manifestation of ongoing or secondary injury. In children, low P bt O 2 is associated with poor outcome, is a manifestation of secondary injury, and responds to global changes in blood pressure, ICP ( Fig. 7.1 ), and systemic oxygenation.

Near-Infrared Spectroscopy
Theoretically, near-infrared spectroscopy (NIRS) may be more promising in young children and neonates in whom transillumination is easier. Although many report the usefulness of NIRS in adults and children when there is no primary brain injury, as in carotid endarterectomy and pediatric cardiac anesthesia, studies of its utility in the pediatric TBI population are limited. Although some suggest promising results, technical factors that interfere with signal reliability, especially in head trauma, may limit the utility of the method with current technology. Reduced correlation between NIRS values and other markers of oxygenation, such as jugular venous oxygen saturation (SjvO 2 ) and P bt O 2 , has been reported, and normal NIRS values have been found with complete ischemia. However, the development of newer technologies may reduce these technical limitations. NIRS may be used as a noninvasive tool for measuring physiologic phenomena, such as autoregulation and the effect of ICP.
Microdialysis
Very few cerebral microdialysis studies have been performed in children, but these have highlighted possible pathophysiologic differences between adult and pediatric TBI. In general, the principles are expected to be largely similar in children, as would be the limitations. The expense, infrastructure required, intermittent sampling technique, and lack of an “early warning system” have limited the more widespread use of microdialysis in pediatric ICUs.
Jugular Bulb Venous Oxygen Saturation
There are fewer reports of SjvO 2 monitoring in children compared with adults. The technique in children has been described. Perez et al. found that poor outcome in pediatric TBI was associated with episodes of SjvO 2 less than 55% and pathologic arteriovenous difference in lactate. The technical limitations of SjvO 2 have not been specifically described in children, but are probably similar to those in adults.
Transcranial Doppler
Transcranial Doppler (TCD) monitoring has been used as a noninvasive monitoring tool in pediatric TBI, hydrocephalus, and meningitis. Insonation of the basal vessels is usually easier in children because of the thinner temporal bone. The optimal depth of insonation of the middle cerebral artery (MCA), is shorter in children than in adults and depends on age and other physical factors such as scalp swelling after trauma. Normal values for TCD-derived flow velocity (FV) in the MCA (FV MCA ) depend on age. FV MCA is approximately 24 cm/second in newborns, increases with age to peak at 97 cm per second at 6 to 9 years old, and then decreases to adult values of about 50 cm per second. The reported age for peak mean FV MCA in children is slightly higher than the age reported for peak CBF. There also appear to be slight gender differences in anterior and posterior circulation FV in children.
The TCD-derived pulsatility index (PI) may be useful for the noninvasive estimation of ICP and CPP in adults. Although this is not as well defined in children, the PI may be associated with outcome after pediatric TBI and may be useful as a guide in treating hydrocephalus. The Lindegaard index may be helpful in monitoring vasospasm in adult TBI. Although vasospasm appears to be uncommon after pediatric severe TBI, monitoring for vasospasm may be useful for subarachnoid hemorrhage or meningitis.
Pressure Autoregulation
Pressure autoregulation (PAR) may be impaired in about 40% of children with severe TBI. Although this has implications for clinical management, PAR is not commonly measured in children with TBI. If PAR is impaired, maintenance of adequate CBF may require increased CPP, but higher CPP may also increase cerebral blood volume and ICP. Impaired PAR is associated with poor outcome in adults and children. Testing of PAR has been well described and bedside methods have been validated. TCD-based methods are the most common bedside techniques used in children. The strength of PAR in children may vary between the two hemispheres and may change over time. Continuous measurement of the strength of PAR over time has also been described in children. The status of PAR has profound consequences for the effect of BP changes on ICP and perfusion of the brain in pediatric TBI. Ideally, the status of PAR should be known when making decisions about what BP target is optimal in individual patients. Important issues that still need to be clarified in children include whether the autoregulation curve is similar to that of adults and whether information from testing PAR leads to therapeutic decisions that benefit the patient.
Computed Tomography Scanning
Head CT is used for diagnosis, to estimate prognosis, to monitor progress, and as a guide to treatment. Unlike adult TBI, diffuse injury is more common than focal injury in children with severe TBI and mass lesions requiring surgical removal are less common. Diffuse brain swelling has been reported to be the most common CT finding in children with severe TBI and increases the likelihood of poor outcome. Subarachnoid hemorrhage on head CT has also been associated with poor outcome in pediatric TBI. In the presence of brain swelling, often the basal cisterns on head CT are effaced or obliterated; however, in children increased ICP can occur in the presence of completely normal-appearing cisterns. There is increasing concern about the effects of head CT on the developing brain. Careful thought should be given to children who require multiple CT scans, including those of other body parts and studies such as CT-perfusion or angiography, and alternative examinations sought, if feasible, to reduce the total radiation dose and the potential for cumulative deleterious effects. For example, susceptibility-weighted imaging (SWI), a relatively novel MRI sequence may be useful in select patients to detect hemorrhagic lesions. However, its clinical usefulness in children is still to be elucidated.
Serum Biomarkers
Various serum biomarkers have been examined in adult and pediatric TBI for prognosticating and predicting the need for imaging. Berger et al. found that biomarkers (neuron-specific enolase, myelin-basic protein and S100-B) were predictive of outcome. Specifically, the prediction of good outcome (97%) was better than the prediction of poor outcome (75%), and peak concentrations correlated better with outcome than initial concentrations. However, the relative strengths of association between biomarkers and outcome for different childhood age groups still need further clarification, and it is unclear whether biomarker levels will influence acute management or be used primarily to predict outcome.
Glucose, Hemoglobin, and Temperature Monitoring
Global trends have favored more conservative thresholds for blood transfusion in critically ill children ; however, acute anemia may have particular risks in TBI patients. Studies examining the effect of blood transfusion on brain oxygenation in adults and children demonstrate increased oxygenation with transfusion; however, this tends to be temporary. Transfusion does not universally increase oxygenation, and the prediction of the effect based on pretransfusion characteristics is incomplete. Therefore, optimal hemoglobin concentrations, or transfusion triggers, in critically ill children with neurologic pathology remain debated. It may be that the optimal level depends on the underlying severity of brain injury and risk of ischemia.
Glucose control is similarly controversial. Hyperglycemia is associated with worse outcome in children with TBI, and strict control of glucose in pediatric trauma patients has been recommended. However, whether hyperglycemia is a modifiable factor that causes secondary injury or is merely a marker of injury severity remains uncertain. Furthermore, strict control of glucose may significantly reduce cerebral glucose, increase markers of tissue injury, and worsen outcome. No studies have examined the impact on outcome of strict glycemic control in children with severe TBI.
Core temperature is routinely monitored in pediatric critical care, and hyperthermia should be avoided. Induced hypothermia remains controversial for pediatric TBI, but is better established as a therapy for other conditions, such as perinatal asphyxia.
Other
Although not strictly a monitoring modality, imaging of the brain has evolved substantially and has become a powerful tool for research and outcome prediction in TBI. Tools such as diffusion-weighted imaging, susceptibility-weighted imaging, magnetic resonance spectroscopy, and diffusion tensor imaging have contributed to the appreciation of edema, microhemorrhages, ischemia, and white matter injury in adult and pediatric TBI. Continuous electroencephalographic monitoring can be used to diagnose convulsive and nonconvulsive epileptiform activity and monitor the depth of barbiturate sedation. Seizures may be seen in as many as one third of children in the critical care environment, with the great majority of these being nonconvulsive. Cortical spreading depolarization events happen frequently in adult TBI, and are associated with metabolic disturbances and poor outcome. The role of these events in pediatric neurocritical care has not yet been determined.
Conclusion
There are fewer reports that examine neuromonitoring tools in critically ill children than in adults, so the evidence base for making clinical decisions is small. This is compounded by the increased complexity of changing physiology with age in children, and so the choice of monitoring tools and appropriate targets is arguably more challenging than in adults. It is important to remember that the rules governing monitoring issues in adults may not apply to children, that the nature of the pathology may be different, and that age-related physical and physiologic issues must be taken into account when treating children. Given the increased complexity of brain injury pathophsyiology in children it is arguable that a multimodal approach has even greater value than in adults.
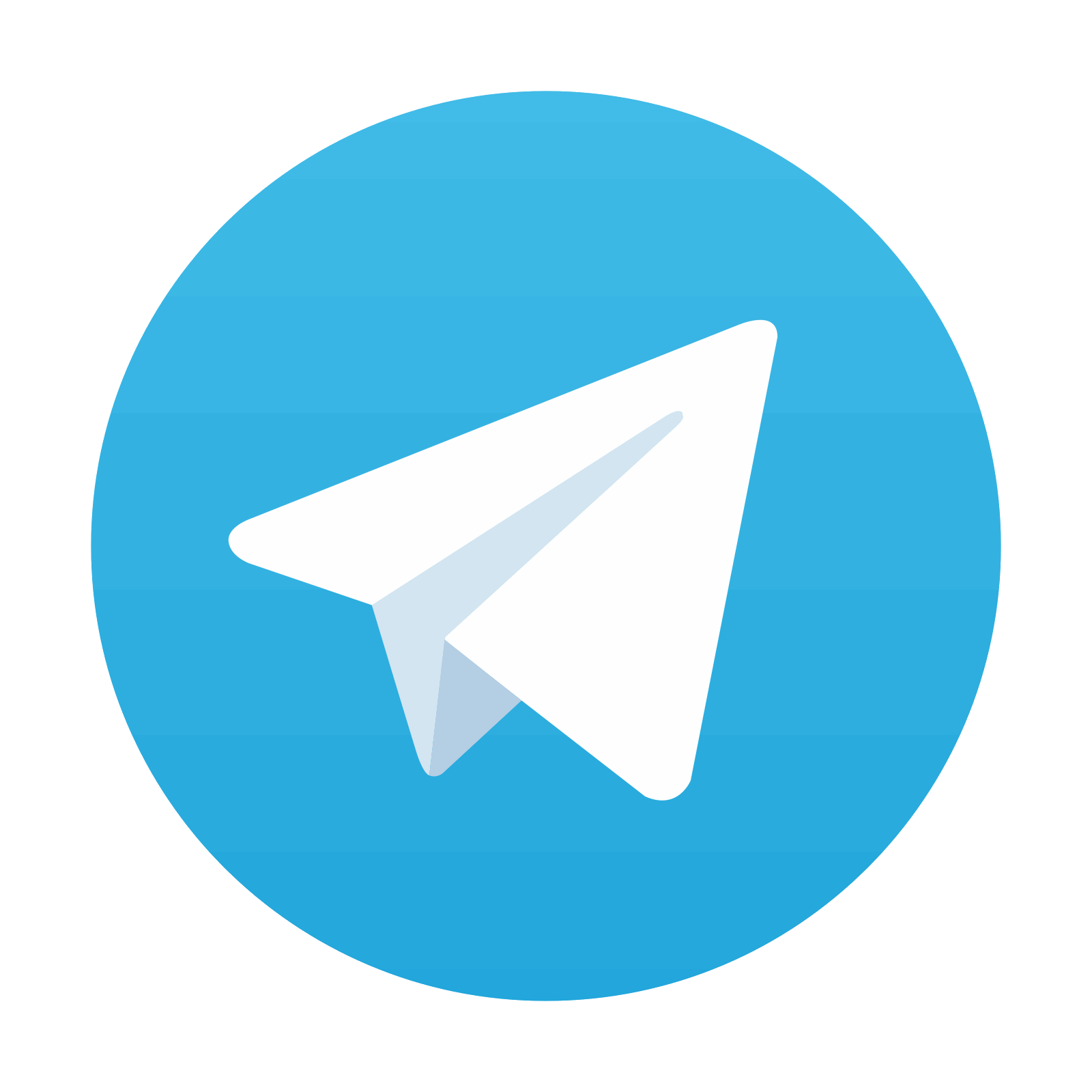
Stay updated, free articles. Join our Telegram channel

Full access? Get Clinical Tree
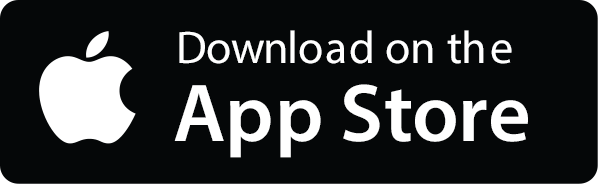
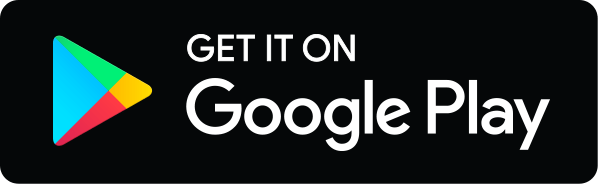