Introduction
Maintenance of an adequate tissue oxygenation is a fundamental objective in the field of critical care medicine in general, and the assessment of tissue oxygenation is an essential part of the management of the critically ill patient. This provides information about oxygen supply and use in specific tissue beds. Hypoxia is defined as a reduction of tissue oxygenation to levels that are insufficient to maintain cellular function and metabolism. The pathophysiology of tissue hypoxia can be classified as follows:
-
Ischemic
-
Cytopathic
-
Anemic
-
Hypoxic
In the brain tissue, ischemic hypoxia may result from macrovascular ischemia (i.e., reduced or absent cerebral blood flow [CBF], e.g., cerebral thrombosis, vasospasm, low arterial carbon dioxide tension [PaCO 2 ], alkalosis) or from microvascular ischemia (e.g., blood-brain barrier disruption and brain capillary endothelial dysfunction). Cytopathic hypoxia in the brain includes a complex cascade of events after the initial brain insult (e.g., glutamate excitotoxicity, intracellular calcium influx, free radical and inflammatory cytokine release) that lead to cell energy and mitochondrial dysfunction, diffusion limited oxygen delivery to the cell, and reduced oxygen extraction. Anemia and impaired systemic oxygenation are additional causes of brain tissue hypoxia ( Table 35.1 ).
Etiology | Pathophysiology |
---|---|
Ischemic | |
Macrovascular | ↓ O 2 delivery, ↓ CBF (thrombosis, vasospasm, low PaCO 2 ) |
Microvascular | BBB disruption, vasogenic edema, endothelial dysfunction |
Cytopathic | ↓ O 2 extraction (cytotoxic edema, diffusion limited O 2 delivery, mitochondrial dysfunction) |
Anemic | ↓ Hemoglobin concentration |
Hypoxic | ↓ PaO 2 , SaO 2 |
Brain hypoxia can cause secondary brain damage. Thus monitoring of brain tissue oxygen partial pressure to discriminate between normal and critically impaired tissue oxygenation has been integrated into the management of patients with acute brain injury, and several lines of evidence suggest that it is a clinically useful modality in neurocritical care. In addition, several observational studies show that cerebral perfusion pressure (CPP) and intracranial pressure (ICP) are not surrogates for brain oxygen and that brain oxygenation varies independently of cerebral hemodynamics and ICP. Like other monitors, a brain oxygen monitor is not intended to be used alone; instead it should be used as a supplement or complement to other monitors, for example, an ICP monitor (see Chapter 34 ) or microdialysis (see Chapter 36 ).
Definition
Brain tissue oxygen is defined as the partial pressure of oxygen in the interstitial space of the brain and reflects the availability of oxygen for oxidative energy production. There has been some debate as to whether the monitor systems measure tissue oxygen pressure or tension, and, accordingly, several abbreviations have been used for brain tissue oxygen, including PbO 2 , PbrO 2 , P bt O 2 , PtiO 2 , and BTO 2 . A consensus conference at the 13th International Symposium on Intracranial Pressure and Brain Monitoring held July 2007 in San Francisco, California, proposed that P bt O 2 be used as the standard abbreviation. Accordingly, this chapter uses the P bt O 2 when referring to brain tissue oxygen pressure. Consistent with this abbreviation clinical studies suggest that P bt O 2 may be best defined by the equation:
where AVTO 2 is arterial oxygen tension (PaO 2 ) minus oxygen partial pressure in venous blood (PvO 2 )—that is, P bt O 2 represents the interaction between plasma oxygen tension and CBF.
Technology
There are four methods to measure brain oxygen: jugular venous bulb oximetry ( Chapter 32 ), direct brain tissue oxygen tension measurement, near infrared spectroscopy ( Chapter 33 ), and oxygen-15 positron emission tomography (PET) ( Chapter 29 ). This chapter discusses direct brain tissue oxygen tension measurement, which is now the most common technique used in the neurocritical care unit (NCCU) to assess cerebral oxygenation. The technique involves the insertion of a fine catheter (approximately 0.5 mm in diameter) into the brain parenchyma, specifically the white matter, to continuously measure P bt O 2 . The P bt O 2 catheters or probes can be inserted through a single- or multiple-lumen bolt secured in a single burr hole or they may be tunneled under the scalp. The procedure to insert the monitor may be performed in the operating room or at the bedside in the NCCU. P bt O 2 probes generally are placed adjacent to an ICP monitor and through the same triple-lumen bolt. Ideally the position and function of the monitor should be confirmed with a head computed tomography (CT) scan or oxygen challenge test particularly if the initial P bt O 2 reading after 30 to 60 minutes of stabilization is abnormal.
Two primary technologies underlie P bt O 2 measurement: one is based on the Clark principle, the other on an optical technique. The Clark principle uses the electrochemical properties of noble metals to measure the oxygen content of tissue. The Clark electrode consists of a membrane that covers a layer of electrolyte and two metallic electrodes. Oxygen diffuses through the membrane and is electrochemically reduced at the cathode. The greater the oxygen partial pressure, the more oxygen diffuses through the membrane. The change in voltage between the reference electrode and the measuring electrode is proportional to the amount of oxygen molecules being reduced on the cathode. The Licox P bt O 2 probe (Integra Lifesciences, Plainsboro, NJ) uses such a closed polarographic Clark-type cell with reversible electrochemical electrodes ( Fig. 35.1 ). This oxygen-consuming process is temperature dependent, and so requires constant calibration of the system to patient temperature. The Licox system includes a brain temperature probe (thermocouple) that is inserted through a triple-lumen bolt with the P bt O 2 and ICP monitor (e.g., Camino, Integra Lifesciences). This temperature probe measures brain temperature and allows for automatic calibration. The new Licox PMO probe can measure P bt O 2 and brain temperature using a single probe. The Neurovent-P Temp (Raumedic AG, Munchberg, Germany), which uses the same polarographic technique as the Licox, also can measure P bt O 2 and brain temperature in one catheter. There are important differences in values obtained by the Neurovent and Licox devices and so the values obtained by these devices cannot be used interchangeably. Purpose-designed triple-lumen cranial access devices allow simultaneous P bt O 2 , ICP, and cerebral microdialysis monitoring. However, if brain temperature is not measured (e.g., a microdialysis catheter is inserted through the lumen instead of the temperature probe), the monitor should be calibrated manually on a regular basis, for example, every 30 minutes by using core body temperature. The Licox system averages oxygen over a probe area of 14 to 18 mm 2 and has excellent long-term stability, even after 7 days. The Neurovent-P Temp has a greater surface area of 24 mm 2 .


The second technique used to measure P bt O 2 is based on a fluorescence quenching technique in which a marker changes color according to the ambient amount of gas. Optode sensors are used to measure concentrations of substances by photochemical reactions that create changes in optical properties of indicator compounds. OxyLabPO 2 (Oxford Optronix Ltd., Oxford, UK) allows P bt O 2 to be measured using optical fluorescence technology. Unlike the Licox, this process does not consume oxygen and does not affect the measured oxygen level. However, the probe measures a smaller area. The Neurotrend (Codman, Johnson & Johnson, Raynham, MA) uses this technique, but it is no longer commercially available for clinical use. The accuracy and clinical stability of the Neurotrend sensor also appears to be less than the Licox system. There are several other important differences between techniques based on the Clark principle (e.g., the Licox) and on an optical technique (e.g., Neurotrend). First, Licox catheters are precalibrated and can be inserted without any pre-use calibration. However, postinsertion stabilization (about 1 hour) is required before readings are reliable. By contrast, the Neurotrend monitor needs bedside calibration to a defined oxygen concentration. Second, the catheters are of different lengths; the Neurotrend is inserted at a greater depth than the Licox catheter. Third, the critical P bt O 2 threshold for hypoxia is different, so it is difficult to compare studies that use the different techniques. In addition, the Neurotrend changed design in 1998, making it difficult to compare between old and more recent studies that describe this technology.
Catheter Placement
P bt O 2 catheters are placed in the right frontal lobe white matter in diffuse brain injury or on the side of maximal pathology when there is focal injury. In subarachnoid hemorrhage (SAH) patients, the authors place the P bt O 2 monitor where maximal vasospasm is likely to develop based on the distribution of SAH and aneurysm location. Recommendations for the preferred brain area to be monitored with a P bt O 2 probe are summarized in Table 35.2 .
Intracranial Pathology | Catheter Location |
---|---|
Traumatic brain injury | |
Diffuse injury | Right frontal lobe |
Focal injury (subdural hematoma, contusion) | Pericontusional tissue |
Subarachnoid hemorrhage | Expected distribution area of the parent artery of the aneurysm, at highest risk for developing symptomatic vasospasm and delayed ischemia |
Cerebral infarction | Area of lesion, at distance from the infarcted tissue |
In general, monitors are placed when the Glasgow Coma Scale (GCS) score is equal to or less than 8 (i.e., consistent with the indications for an ICP monitor) (see Chapter 34 ). There are no specific guidelines on how long P bt O 2 should be monitored. In traumatic brain injury (TBI) patients the authors leave the monitor in place until ICP is normal for 24 hours without any specific treatment other than sedation for ventilation. On average, in patients with severe TBI this is 4 to 5 days. In SAH patients the monitor is kept in place during the risk period for vasospasm. In addition, continuous P bt O 2 can be monitored twice as long as jugular venous oxygen saturation (SjvO 2 ) and without needing recalibration. In studies that compare P bt O 2 and SjvO 2, good quality data are acquired 95% of the time with P bt O 2 , but only 43% of the time for SjvO 2 . Postinsertion noncontrast head CT confirmation of probe position in the brain parenchyma ( Fig. 35.2 ) is important to interpret readings. In some patients a CT-perfusion study also may be useful. For example, if P bt O 2 readings are consistently low or poorly responsive to therapy, it is useful to know the monitor’s proximity to a focal abnormality or whether the region it is in is hypoperfused. In these cases the P bt O 2 threshold for treatment may be lower than normally used. A “run-in” or equilibration time of up to 1 hour is required before readings are stable. Adjustment of insertion depth (when used through an access device) is not possible with the Licox system, and if the monitor is removed, the insertion bolt then should be replaced if a new monitor is inserted to avoid potential contamination.

Transiently increasing the inspiratory oxygen fraction (FiO 2 ) and observing the corresponding P bt O 2 increase can help confirm function or exclude the presence of surrounding microhemorrhages or sensor damage at insertion. An oxygen challenge is therefore performed when monitoring starts and, in some institutions, daily thereafter as part of regular clinical care to evaluate the function and responsiveness of the P bt O 2 probe or to determine “oxygen reactivity.” The oxygen challenge involves increasing the FiO 2 from baseline to 1.0 for approximately 5 minutes. This typically leads to a several-fold increase in the amount of oxygen dissolved in the plasma, translating into a mean threefold increase in P bt O 2 . However, the response to hyperoxia usually is less robust when the monitor is in an underperfused (CBF is <20 mL/100 g/min) region.
Regional Versus Global Measurement
P bt O 2 probes sample approximately 15 mm 2 of tissue around the tip, and the P bt O 2 value depends on O 2 diffusion from the vasculature to a small amount of tissue. Therefore it is a regional monitor with values that depend on probe location. This has led to debate about probe location and whether the value can be used to make decisions about global oxygenation. In most patients P bt O 2 is measured in “normal appearing” frontal subcortical white matter; there is evidence that this local measurement can be regarded as an indicator of global oxygenation. Consistent with this, two clinical studies have shown good correlation between P bt O 2 and SjvO 2 , used to assess global brain oxygenation, in areas without focal pathology after TBI. In areas with focal pathology, this correlation between P bt O 2 and SjvO 2 was absent, and the brain tissue oximetry values reflected regional brain oxygenation better than jugular bulb oximetry. Other studies also show that when the probe is in tissue immediately adjacent to a contusion or other pathology (e.g., a subdural hematoma), values are often lower, even if CPP is higher. In addition, when the monitor is placed adjacent to abnormal brain, regional hypoxia lasts longer than in normal-appearing tissue and the relationship with outcome appears to be more robust.
Values in Normal and Pathologic Conditions
Normal Values
The Licox monitor provides a measure of P bt O 2 in units of tension (mm Hg). Usually oxygen content is expressed in units of concentration (mL O 2 /100 mL) and oxygen delivery to the brain and cerebral metabolic rate of oxygen (CMRO 2 ) are expressed in mL O 2 /100 g brain/minute. The conversion factor: 1 mm Hg equals 0.003 mL O 2 /100 g brain can be used to compare P bt O 2 with other oxygen concentration measurements. Normal P bt O 2 measurements have been acquired experimentally, but human measurements have been restricted to “normal” values obtained during neurosurgical procedures and in normal-appearing brain after TBI. In animals, normal P bt O 2 varies from 30 to 40 mm Hg. In humans without severe brain injury, P bt O 2 ranges from 37 to 48 mm Hg. Mean values of 23 mm Hg have been measured in awake subjects undergoing functional neurosurgery. P bt O 2 values in patients with normal ICP are between 25 and 30 mm Hg in TBI.
Hypoxic Thresholds
The identification of hypoxic brain tissue permits intervention potentially before irreversible injury occurs; that is, the therapeutic window can be widened. In addition, threshold values provide therapeutic endpoints. However, in managing a patient, trends over time and how the P bt O 2 value relates to other variables such as ICP and CPP, also should be considered. In addition, threshold is not the only factor that is important in terms of outcome; the duration spent below threshold and the depth or severity of brain tissue hypoxia also are important. Indeed the duration of P bt O 2 less than 10 mm Hg is an independent factor associated with poor outcome after severe TBI, and this association also is independent of ICP. Threshold values can vary with probe type and probe site, and so values obtained with different makes of monitors (e.g., Licox vs. Neurovent vs. Neurotrend) are not interchangeable. Various P bt O 2 thresholds have been proposed. A P bt O 2 less than 20 mm Hg indicates compromised brain tissue oxygen and often is taken as a threshold at which to consider or initiate therapy to correct brain oxygen. This value is the threshold at which therapy is initiated in an National Institutes of Health (NIH)–sponsored phase II trial that is expected to finish patient recruitment in late 2013. The 2007 guidelines for severe TBI recommend that P bt O 2 values less than 15 mm Hg be considered as the critical threshold for “ischemia.” Microdialysis studies show that at this level other markers of ischemia are elevated. Normal mitochondria require an oxygen level of about 1.5 mm Hg to function; this corresponds with a P bt O 2 level between 15 and 20 mm Hg in normal white matter. A P bt O 2 less than 10 mm Hg is a marker of severe brain tissue hypoxia and is an independent factor associated with both mortality and unfavorable outcome. Values of 0 mm Hg that persist longer than 30 minutes and show no response to an oxygen challenge are consistent with brain death. Table 35.3 summarizes normal and hypoxic thresholds of P bt O 2 .
Condition | P bt O 2 Values |
---|---|
Normal | 25-50 mm Hg |
Hypoxic thresholds | |
Moderate brain hypoxia | 15-25 mm Hg |
Critical brain hypoxia | <15 mm Hg * |
Severe brain hypoxia | <10 mm Hg |
Elevated | >50 mm Hg |
* P bt O 2 thresholds according to Brain Trauma Foundation guidelines. In a National Institutes of Health–sponsored phase II trial that is expected to finish patient recrurtment in 2013 to examine P bt O 2 , treatment is initiated when P bt O 2 is <20 mm Hg.
Observational Data
A large body of observational clinical data on P bt O 2 , primarily in patients with severe TBI and less frequently SAH, has accrued since the 1980s. These findings may be summarized as follows. First, P bt O 2 may be abnormal, despite a normal ICP and CPP both in adults and children and when SjvO 2 is normal. This suggests that P bt O 2 may be a novel target for resuscitation following brain injury and can supplement the information provided by an ICP monitor or retrograde jugular catheter. Second, compromised P bt O 2 is common after acute brain injury even after adequate resuscitation, defined as normal ICP and CPP, and an episode of reduced P bt O 2 may complicate the ICU course of up to 70% of patients, again often independently of ICP and CPP. Third, imaging studies obtained at admission after TBI do not reliably predict whether brain hypoxia will develop. Fourth, reduced P bt O 2 is associated with other markers of cellular distress such as an elevated lactate-to-pyruvate ratio on microdialysis that can be corrected with a variety of therapies. Common therapies include head position, ventilator manipulation, CPP augmentation, and sedation that overall are successful in correcting the abnormality in about 70% of episodes of reduced P bt O 2 . Fifth, P bt O 2 values may help guide therapeutic interventions (e.g., blood transfusion), osmotherapy, and decompressive craniectomy (DC), or identify deleterious effects of other treatments such as vasoconstriction with hyperventilation or shivering with induced hypothermia. In addition, P bt O 2 monitors may identify patients at risk for cerebral compromise associated with hospital transport. Sixth, P bt O 2 -pressure reactivity may be used to assess cerebral autoregulation and help target CPP in individual patients. Seventh, P bt O 2 has been used for early detection of delayed cerebral ischemia (DCI) in SAH patients and to evaluate the effects of various therapies for DCI, temporary artery occlusion during surgery, angiography, or pharmacologic angioplasty. In addition, studies in SAH have shown that in some patients nimodipine or intra-arterial papaverine can have unexpected adverse effects on P bt O 2 . Finally, several observational studies demonstrate an independent association between low P bt O 2 and poor outcome in adults as well as children with TBI and in SAH. Together these various observations suggest that care based on information provided by a P bt O 2 monitor may be a reasonable strategy in severe acute brain injury. Consistent with this several observational studies or based on historical controls, but not all, suggest that the combination of P bt O 2 – and ICP-based care is associated with better outcome than just ICP-based care alone. In part this potential outcome benefit may be associated with better-targeted care in each patient, determination of an “optimal” CPP, and avoidance of inappropriate ICP treatment and potential side effects if ICP is slightly elevated (≈25 mm Hg) but P bt O 2 is normal, a concept known as permissive intracranial hypertension . At the time this book was published a multicenter phase II trial was underway to further evaluate the role of P bt O 2 -based care in severe TBI.
Interpretation of Measured Values
Information from a P bt O 2 monitor, like other monitors, should be interpreted with other data such as clinical examination, CT scan findings, ICP, CPP, pulmonary status, and hemoglobin among other variables. In addition, the P bt O 2 value needs to be temperature corrected. This is performed automatically when a brain temperature probe is placed with a Licox; if a temperature probe is not inserted, systemic temperature can be manually entered into the device. It also is essential to know exactly where the monitor is placed (see earlier text). In the brain the sensor reads an integration of all small vessels in the area of the probe, and so the P bt O 2 value depends on the relative predominance of veins or arteries, and the number, diameter, and spatial distribution of the local microvasculature and so can be influenced by changes in cerebral capillary perfusion. Because the venous fraction within cortical microvasculature is 70%, it has been suggested that P bt O 2 predominantly reflects venous PO 2 . However, exactly what P bt O 2 measures is still to be fully elucidated but it likely represents (1) the balance between regional oxygen delivery and cellular oxygen consumption, (2) oxygen diffusion rather than total oxygen delivery or cerebral oxygen metabolism, or (3) oxygen that accumulates in brain tissue because PET studies suggest it may correlate inversely with oxygen extraction fraction (OEF).
Many factors can affect P bt O 2 . Among these the effect of CPP and CBF has been the most studied. P bt O 2 is associated with regional CBF. This relationship follows the autoregulation curve that regulates CBF across a wide range of mean arterial pressures (MAPs). CPP and MAP augmentation can increase CBF and therefore P bt O 2 , whereas the P bt O 2 response to an oxygen challenge is reduced in areas with low CBF. Together this suggests that P bt O 2 can provide information about CBF and impending cerebral ischemia in certain conditions. However, P bt O 2 is more than a marker of ischemia and PET, and microdialysis studies suggest that hypoxia in the brain can be independent of CPP and more related to diffusion rather than perfusion abnormalities. The total amount of oxygen that diffuses across the blood-brain barrier per unit time is defined as follows:
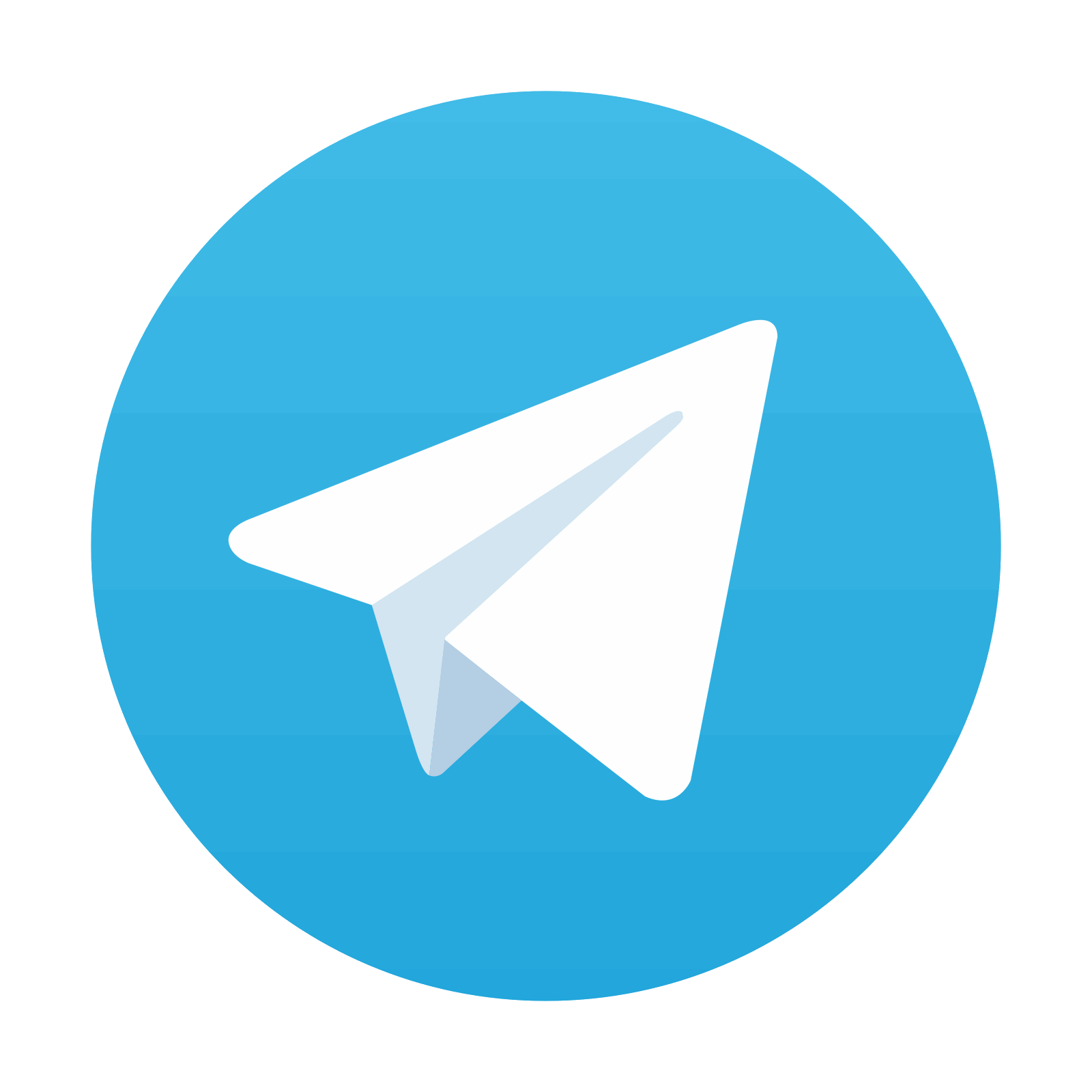
Stay updated, free articles. Join our Telegram channel

Full access? Get Clinical Tree
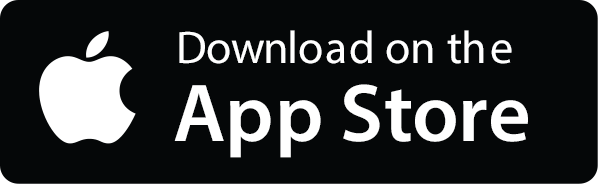
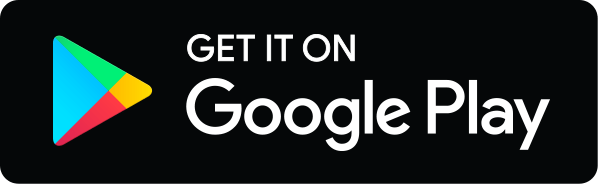