Table 27.2 Tumor Types Encountered in Medically Intractable, Chronic Epilepsy using Data Retrieved from the European Epilepsy Brain Bank
Table 27.3 Seizure Frequency in Various Brain Tumor Types
CLINICAL CHARACTERISTICS
Most tumor-related seizures first appear early in the course of the disease, usually as a presenting manifestation (9,10). In 10% to 30% of brain tumor patients, epilepsy develops later in the course of the disease (4,10). In brain tumor patients presenting with seizures, age and presence of associated neurologic deficits may correlate with tumor grade: Children and adolescents usually have no associated neurologic deficits and generally have a low-grade tumor, whereas middle-aged or elderly people often have other associated neurologic deficits on presentation and frequently present with a high-grade brain neoplasm (3,15,16).
Both focal and generalized seizures occur in the setting of brain tumors (10,11,17–19). Even isolated auras have been reported as the only epileptic manifestation of temporal lobe tumors (20). Therefore, in any given patient, seizure semiology is mainly determined by the location of the tumor and its connectivity. However, certain general remarks may be noted. First, seizures that start earlier in the course of a brain tumor are more likely to be generalized: Hildebrand et al. (10) found that while 50% of “early seizures” occurring at or soon after brain tumor diagnosis were generalized and 40% were focal, the converse was true when seizures occurred during the later follow-up phases, with about 75% being focal and only 20% being generalized. Second, in certain special situations, seizure semiology carries a specific tumor-related diagnostic correlation, for example, gelastic seizures and hypothalamic hamartomas.
The observations made above are likely due to distinctly different mechanisms of epileptogenicity that are detailed later. In brief though, younger patients are statistically more likely to have small, slow-growing tumors (developmental tumors, low-grade gliomas, etc.) that take their time to develop focal and remote cellular and pathway changes sufficient to develop epileptogenicity without causing major local tissue damage. As such, patients with low-grade temporal lobe tumors, for example, might not have deficits on a traditional neurologic examination, develop seizures later in the course of the tumor progression, and have more complex partial seizures related to dysfunction of the limbic network. On the other hand, older patients with glioblastoma multiforme (GBM) usually have a larger rapidly growing tumor, causing significant local tissue damage with associated neurologic deficits and seizures starting earlier in the tumor disease course as a result of abrupt tissue necrosis.
Regardless of the tumor type, patients who present with seizures as the initial symptom of a brain tumor are at higher risk of developing epilepsy later (recurrent seizures), even with prophylactic trials of antiepileptic drug (AED) treatment (21,22). Furthermore, up to 50% of those tumor-related epilepsies may become medically intractable, and this is a higher intractability risk than in other epilepsies (10,19,23).
PROPOSED MECHANISMS OF EPILEPTOGENESIS
The development of epilepsy in a brain tumor patient is probably a multifactorial phenomenon. Therefore, even though we will discuss multiple proposed mechanisms of epileptogenicity in brain tumors, it is important to remember that those mechanisms are not mutually exclusive and that in any given patient, epilepsy is likely due to an interplay of all of those variables.
Role of Tumor Type
High-grade tumors may lead to epilepsy by abruptly damaging local tissue, causing tissue necrosis and hemosiderin deposition, and increasing excitability of local and immediately surrounding cortex (4,16,24). Chronic intractable epilepsy is, however, most often caused by lower-grade tumors, specifically low-grade gliomas and developmental tumors—mainly gangliogliomas and dysembryoplastic neuroepithelial tumors (DNET) (12,13,17,25–28). These developmental tumors are surrounded by dysplastic cortex in 25% to 70% of cases (13,28–30) which should be classified as associated Focal Cortical Dysplasia ILAE Type IIIb, or may be associated with coexistent hippocampal sclerosis (14,30,31). In such a setting of “dual pathology,” seizures may be predominantly or even independently arising from the dysplasia or hippocampal sclerosis, and not necessarily the tumor. A practical and important implication of this “dual pathology” is the inability to control seizures surgically in these patients with chronic intractable epilepsy unless both “lesions” are resected (32).
Role of Peritumoral Morphologic Changes
A brain tumor disrupts the tissue around it and causes a variety of morphologic changes that facilitate excitability and thus increase epileptogenicity. Those changes include aberrant neuronal migration, enhanced intercellular communication through increased expression of gap junctions, changes in synaptic vesicles, and increased local concentrations of glutamate and lactate (3,4,24,33). Synaptic transmission, including GABA receptor signaling, may be underexpressed in brain tumor tissues compared with control tissue, further increasing excitability (34,35).
In addition to the above microscopic and molecular changes, gross tumor-related effects include mass effect, local edema, and increased pressure. Also, local infiltrative tumor growth may cause local irritation and epileptogenicity, presumably through inducing tissue hypoxia (36).
Role of Changes to the Microenvironment
Tumors have increased metabolic requirements, and even with increased angiogenesis, eventually lead to intra- and peritumoral hypoxia. This alkalinizes the interstitial pH and causes glial cell swelling and damage, increasing neuronal excitability and facilitating epileptogenic activity (4,37). The risk of epilepsy further increases because of increased inward sodium currents at the level of the astrocytic cell membrane. This results from defective intracellular mechanisms for deoxyribonucleic acid repair and genetic instability also occurring due to tumor-related hypoxia (3). Cortical hemosiderin deposition is also a frequently considered cause for tumor-related epileptogenesis (38).
Role of Genetic Factors
Long-term epilepsy-associated tumors (LEATs) do not share those molecular oncogenes or tumor suppressor genes typically observed in diffusely infiltrating gliomas, such as IDH1 or 1p/19q deletions. In contrast, the oncofetal marker protein CD34 can be frequently identified, and developmental genes are likely to be involved (39). Mutations in B-RAF (40) or mTOR signaling (41) may also play an important role. Other studies have suggested a role for LGI-1 in tumor-related epilepsy (3,4). This is a tumor suppressor gene absent in GBM and other high-grade invasive tumors. It also happens to be responsible for the rare autosomal dominant lateral TLE. Therefore, some authors suggested that LGI-1 plays a role in both tumor progression and epileptogenesis (3,4).
Posttranscriptional and posttranslational alterations have also been implicated in the genesis of epilepsy in brain tumors. For example, LIM domain-binding 2 (LDB2) transcript, critical for brain development during embryogenesis, was one of the strongest reduced mRNAs in gangliogliomas in recent array analyses. Silencing of LDB2 resulted in substantially aberrant dendritic arborization in cultured developing primary hippocampal neurons. This characterizes yet another molecular mechanism operating in gangliogliomas, contributing to the development of dysplastic neurons and an aberrant neuronal network (42). Recent transcriptomic profiling of human peritumoral neocortex tissues revealed that several genes enriched in focal adhesion and cell adhesion molecular pathways may be involved in tumor-induced epilepsy (43). Lastly, the expression of aquaporin-4, a membrane protein implicated in peritumoral edema generation and in drug-resistant hippocampal sclerosis, was reduced in brain tissue from GBM patients without seizures as opposed to GBM patients with seizures. The aquaporin-4m-RNA levels were identical in these two groups of GBM patients suggesting a posttranslational mechanism (44).
Role of Disruption of Functional Network Topology and Secondary Epileptogenesis
Rather than traditional views conceptualizing the brain as a conglomerate of segregated functional areas, each specifically dedicated to one function, the modern theory of brain networks proposes the presence of cortical networks composed of multiple cortical regions connected via white matter pathways controlling various mainly higher cortical functions and requiring a delicate balance between excitability and inhibition of those multiple pathways to operate correctly (3,34). A disruption of those “normal networks”—as occurs anatomically with a tumor—will disturb this balance, leading to multiple consequences, including deafferentation and release of regulatory inhibition on potentially epileptogenic structures (such as the hippocampus), and the appearance of pathologic, less stable compensatory networks that may themselves be more excitable and thus potentially epileptogenic. This hypothesis is still being investigated, and further research is needed to clarify the full extent of its impact. It might, however, partly explain, among other things, how an epileptogenic focus arises distant from a tumor (14), and why a procedure such as a simple removal of the tumor via a lesionectomy, may not achieve optimal seizure freedom (45).
It has been suggested that in almost one-third of patients with brain tumors and epilepsy, the epileptogenic focus does not correspond to the tumor location. This phenomenon is called secondary epileptogenesis, implying that an actively discharging epileptogenic region induces similar paroxysmal activity in regions distant from the original site. This process is mostly seen with low-grade brain tumors located in the temporal lobe, which frequently present with hippocampal sclerosis (20). In those cases, the “secondary focus” becomes a completely independent epileptic generator that also needs to be removed to achieve seizure freedom in intractable patients. Since young age and long disease duration have been proposed as being the main risk factors for this secondary epileptogenesis (46), early resection of the primary focus—the tumor—has been promoted to avoid the development of an irreversible secondary focus and was actually shown to correlate with better rates of seizure freedom following resective epilepsy surgery (11,17,46).
TREATMENT OF SEIZURES IN THE SETTING OF BRAIN TUMORS
With seizures occurring so frequently in patients with brain tumors, it is important to be aware of the various treatment options available. Very often, adequate treatment of seizures in such a setting requires a multidisciplinary approach, including the patient’s neuro-oncologist, neurosurgeon, and epileptologist. Goals of treatment need to be clarified early on in the treatment course, as well as a clear determination of the risk–benefit ratio of various medical and surgical therapeutic options. For example, aiming at complete seizure freedom in a patient with an inoperable, rapidly growing, GBM while concomitantly using five different AEDs with their associated side effects may actually be counterproductive and worsen the patient’s quality of life. On the other hand, merely aiming for reduction in seizure frequency in a patient with a developmental tumor would likely be an unacceptable treatment goal as resective epilepsy surgery has a high chance of rendering this patient seizure free, with a relatively low risk of complications.
The following section reviews current information on medical and surgical aspects of the treatment of seizures in the setting of brain tumors.
Medical Treatment
Anticonvulsant medications are the mainstay of epilepsy treatment in any patient with seizures, including patients with brain tumors. However, little is known about the specific efficacy of different AEDs in the setting of a brain neoplasm. Many patients have recurrent seizures (60% to 70%) despite the use of AEDs, and results of specific AEDs trials are outlined in Table 27.4. First-line AEDs fail in about 60% of patients and, of the remainder, a similar proportion of second-line treatments with monotherapy or polytherapy fails (10,34). A few retrospective studies have favored the use of valproic acid when compared to phenytoin or carbamazepine. Valproic acid was preferred due to the promptness of achieving a therapeutic level, its enzyme-inhibiting properties that may increase the effectiveness of concomitant chemotherapy, and some potential inherent antitumor effects (3,36,54,55). However, it may cause significant bone marrow suppression, especially in combination with chemotherapy. On the other hand, several prospective studies have recently suggested that gabapentin (51), levetiracetam (47,48,52), topiramate (50), or lacosamide (56) may be effective options for add-on therapy. In one prospective series of 26 patients with primary brain tumors who received add-on levetiracetam, usually in combination with valproic acid, a seizure reduction of more than 50% was observed in 65% of patients (47). Similar success rates were seen with levetiracetam used both as add-on or monotherapy (52,57,58). In a small prospective series of 14 patients with intractable seizures and brain neoplasms, gabapentin was added to phenytoin, carbamazepine, or clobazam. Reduction in seizure frequency was seen in all patients, and more than 50% became seizure free (51). In another prospective observational study of 47 glioma patients, initial or add-on therapy with topiramate achieved complete seizure freedom in 56% of patients with a seizure reduction in an additional 20% after a mean follow-up of 16.5 months (59). Most recently, a prospective pilot study following 14 patients with brain tumors and epilepsy showed a 78.6% responder rate with lacosamide (56). All these prospective studies report a low incidence of side effects, although those were slightly higher with topiramate when compared to others.
Table 27.4 Summary of Studies Evaluating Effectiveness of Various AEDs in the Setting of Seizures in Brain Tumors
Few head-to-head studies compare the effectiveness of various AEDs in treating brain tumor–related epilepsy. Levetiracetam was compared to phenytoin in two retrospective series (60,61) and one prospective study (62). All showed similar efficacy in both groups, although the prospective study suggested better tolerability and response rates with levetiracetam (62). Similarly, a retrospective observational study suggested that oxcarbazepine had similar effectiveness and better tolerability when compared to traditional AEDs (63). Interestingly, one observational study comparing the use of valproic acid and levetiracetam used either alone or in combination to other AEDs found the highest percentage of responders (81.5%) in those treated with the levetiracetam plus valproic acid combination (64).
Special Issues Pertaining to Medical Treatment of Epilepsy in Brain Tumors
Medical Intractability of Epilepsy in Brain Tumors
While 20% to 25% of epilepsy patients in general continue to have frequent seizures despite the use of AEDs at adequate serum concentrations, this medical intractability occurs in up to 50% to 60% of patients with seizures and brain tumors (3,11,15,19,24,34,37). This has been attributed to a variety of possible mechanisms. Overexpression of proteins belonging to the multidrug resistance pathway is a frequently discussed mechanism of refractoriness. These proteins are members of the adenosine triphosphate–binding cassette transporter family, normally present in the apical membranes of endothelial cells. The multidrug-resistance gene (MDR)-1 (ABCB1, P-glycoprotein) and multidrug-resistance–related protein (MRP, ABCC1) contribute to the blood–brain and blood–cerebrospinal fluid barriers by controlling the transport of various lipophilic substances in and out of the brain. Many AEDs, including phenytoin, carbamazepine, lamotrigine, felbamate, and phenobarbital, are substrates for MDR-1 products, and are therefore actively eliminated from the intracellular milieu and brain parenchyma when the MDR proteins are overexpressed. Such overexpression has been found for MDR-1 in cells of patients with gliomas and gangliogliomas (19,24,65) and for another MDR protein, the breast cancer resistance protein, in endothelial cells of brain tumor specimens from astrocytomas, anaplastic astrocytomas, and GBM (37). Gabapentin may be transported via a nonspecific transporter out of the brain. However, levetiracetam does not seem to be a substrate for either MDR-1 or other MDR proteins, while the histone deacetylase–inhibiting effects of valproic acid might reduce the expression of P-glycoprotein and MRP-1, raising interest in the potential usefulness of those AEDs in intractable patients (37). This needs to be confirmed by further studies.
Other proposed mechanisms of AED resistance in brain tumors are reduced drug receptor sensitivity, including ion channels, neurotransmitter receptors, and metabolic enzymes involved in the activity of neurotransmitters, as well as reduction in the concentration of several enzyme-inducing AEDs caused by concomitant use of chemotherapeutic agents. Figure 27.1 illustrates the interaction among various proposed mechanisms of resistance to medical therapy.
Figure 27.1. Decreased receptor sensitivity and increased expression of multidrug resistance proteins play the most important roles in medical refractoriness seen with brain tumors and epilepsy.
Issues Related to Interactions Between AEDs and Antineoplastic Agents
There is a significant risk for drug–drug interactions during concomitant use of AEDs and chemotherapeutic agents, as both are substrates for the same hepatic metabolic pathways, mainly the cytochrome P450 system. Carbamazepine, phenytoin, phenobarbital, and primidone, and to a lesser extent lamotrigine and topiramate, have prominent cytochrome P450 enzyme–inducing effects, while valproic acid has an inhibitory effect. Induction or inhibition of these enzymes by AEDs can cause a decrease or increase in anticancer drug concentrations and thus possibly their effectiveness. Similarly, enzyme inhibition or induction by anticancer drugs can lead to toxicity or loss of seizure control (37,66). Newer AEDs such as gabapentin, levetiracetam, and pregabalin do not influence the cytochrome P450 or other metabolic pathways and theoretically only have minimal risk for drug–drug interactions with chemotherapeutic agents (4,24). Focal treatment with AEDs has been investigated in animal models to circumvent efficacy and toxicity challenges, including intraparenchymal injections, self-contained implanted devices, or convection-enhanced delivery (34). Further data are needed before definite statements can be made about the clinical applicability of these investigations.
Dexamethasone is frequently used in the treatment of tumor-associated edema and also interacts significantly with certain AEDs. It competes with phenytoin for protein binding, raising its blood concentration. Conversely, it may cause the opposite effect of lowering phenytoin’s serum concentration by affecting its hepatic metabolism (24).
In summary, significant risks for reduced effectiveness and toxicity exist for both chemotherapeutic agents and enzyme-inducing and enzyme-inhibiting AEDs when used concomitantly. The patient should be cautiously observed for side effects, and serum AED blood levels need to be monitored closely.
AED Prophylaxis in Brain Tumors
The American Academy of Neurology published a consensus statement in 2000 recommending that AED prophylaxis should not be used, and current AEDs should be discontinued, in brain tumor patients who do not have a history of seizures (21). A more recent meta-analysis of controlled clinical trials evaluating the effectiveness of seizure prophylaxis in people with brain tumors, performed between 1966 and 2007, found no difference between the treatment interventions and the control groups in preventing a first seizure in participants with brain tumors concluding that the evidence is neutral, neither for nor against seizure prophylaxis, in people with brain tumors (22). The authors recognized that these conclusions apply only for the older AEDs: phenytoin, phenobarbital, and divalproex sodium (22). Therefore, based on current evidence, the decision to start prophylactic AED use in brain tumor patients should ultimately be guided by assessment of individual risk factors and careful discussion with patients, keeping in mind that there is no strong evidence that any of the currently available AEDs reliably prevent a first seizure in a brain tumor patient.
Surgical Treatment
In general, the following questions are considered when evaluating the surgical management of a patient with tumor- associated epilepsy: does this patient really need surgery now to treat his or her seizures, or is medical therapy sufficient? Should any kind of neurophysiologic testing be performed prior to surgery? What type of surgery should be offered? Will a lesionectomy be appropriate, or is a more aggressive resection required? How can this patient be counseled about his or her seizure outcome following surgery? Despite extensive literature on these topics, such decisions remain very much patient dependent, and a careful consideration of all treatment options as well as a clear and educated patient informed consent process represent the cornerstones of a successful “outcome.” Although the WHO has published a classification system for brain tumors, only few prognostic and predictive tumor-specific features are available for those tumors typically associated with early-onset epilepsy (67). Indeed, neuropathologic agreement for microscopic diagnosis is only moderate and is discussed further below. The following segments briefly address some of the questions raised above.
Timing of Surgery: Now or Later?
A critical piece of information that determines the answer to this question is the type and grade of the tumor in question. Alternatively asked, this question is equivalent to deciding whether the patient needs “tumor surgery” or “epilepsy surgery.” In patients with a high-grade brain tumor, or one with a high risk of malignant transformation such as gangliogliomas, surgical removal is an essential part of the tumor treatment and should be performed regardless of whether it is believed to help with seizures or not in order to improve the patient’s quality of life and chances of survival (16,17,20,24,25,68). On the other hand, most developmental tumors and many low-grade tumors may be observed for years from a tumor treatment perspective or be treated with chemotherapy or radiotherapy. “Tumor surgery” is therefore not required immediately, and the decision to operate would mainly depend on whether a patient’s seizures are controlled with AEDs or not. Many epileptologists usually wait until seizures are medically intractable before pursuing surgical tumor removal. However, many studies suggest that a shorter epilepsy duration at the time of tumor resection is an important predictor of postoperative seizure freedom (69,70). In a retrospective review of 332 patients following resection of low-grade gliomas, postoperative seizure control was significantly poorer in patients with longer seizure history (P < 0.001) (11). In another study evaluating the seizure outcome of 26 children following resection of their DNETs, all patients with epilepsy duration of <2 years were seizure free at 12 months after surgery as opposed to 7/11 of those with longer seizure history (23). Such observations, together with the high risk of intractability in low-grade tumors (1,3,4), support early removal of low-grade brain tumors associated with epilepsy, especially when the tumor is easily surgically accessible (3). At any rate, a careful preoperative attempt at determining the nature of the tumor should be one of the initial steps in evaluating whether a patient needs immediate surgery or not.
Presurgical Neurophysiologic Evaluation
In most tumor-related epilepsy surgery patients, preoperative video-EEG evaluations confirm that seizures arise from the lobe involved with the tumor. In one study of children with DNET, all but one case had ictal onset discharges unilaterally concordant with the tumor location (23). In another series of adult tumor-related epilepsy patients, the epileptogenic focus as determined by interictal and ictal recordings agreed with the involved lobe in 72% of the cases (28). So, why do we need to perform video-EEG evaluations prior to treating the epilepsy of a brain tumor patient by simply removing the tumor? Several hypothetical and evidence-based outcome data support this practice. First, it is important to acknowledge that even though the above-mentioned studies showed seizures arising from the “same lobe” as the tumor, electrocorticographic recordings usually show that the tumors themselves are electrically inert and that epilepsy often arises from the tissue surrounding the tumor (33). Some have even suggested that seizures arise distant from the tumor in up to a third of patients with brain tumors and epilepsy (10,14). So, a preoperative video-EEG evaluation may provide evidence for the extent of epileptogenicity in the surrounding brain tissues. Figure 27.2 illustrates a case where a subdural EEG evaluation confirmed ictal onset distal from the tumor and where seizure freedom was achieved by resecting both the lesion and surrounding cortex. Second, not all brain tumors are epileptogenic and not all “spells” are epileptic, so a video-EEG evaluation may be helpful in confirming the epileptic nature of a patient’s spells and characterizing the relationship of the epileptogenic focus to the tumor itself (29). Third, some studies have reported better seizure outcomes with the use of intraoperative electrocorticography in tumor surgery and the resection of intraoperatively identified zones of interictal spiking and ictal onsets (3,25,71). In a study of 35 patients with intractable TLE related to benign mass lesions, the number of 3-year postoperative seizure-free incidences for the group that underwent lesionectomy plus additional spike-positive site resection equated to 90.9%. In contrast, in the group that underwent a lesionectomy only, 76.9% were seizure free for 3 years postoperatively (71). However, such results need to be reproduced in larger-scale randomized studies. Lastly, when tumors occur in proximity to eloquent cortex, intra- or extraoperative functional mapping is often essential in determining the extent of the surgical resection (72). For all the above reasons, it is recommended to perform a neurophysiologic evaluation, preferably at least a video-EEG evaluation with ictal recordings, prior to proceeding to surgery in a brain tumor patient with seizures.
Figure 27.2. This figure illustrates the case of a 10-year-old boy with a left parietal low-grade glioma where subdural EEG recordings showed interictal activity diffusely, both proximal and distal to the lesion (light gray), and two different seizure patterns: one arising from the lesion itself (speckled gray) and one arising somewhat distally from the lesion (dark gray). Central sulcus (CS) location is also illustrated. An “extensive lesionectomy” was performed to include areas of identified epileptogenicity rendering the patient seizure free (follow-up of 2 years). A and C show the preoperative and postoperative MRI, respectively.
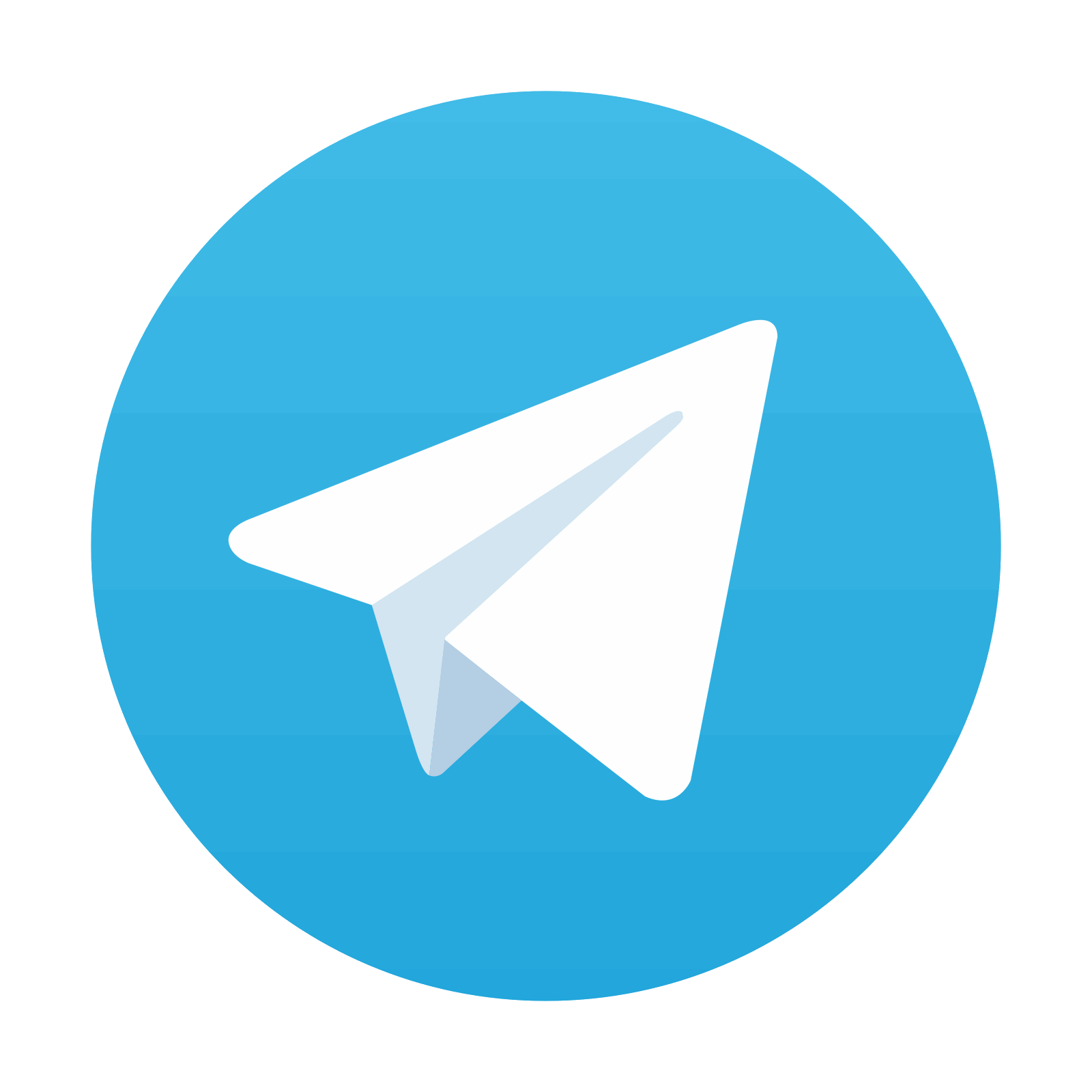
Stay updated, free articles. Join our Telegram channel

Full access? Get Clinical Tree
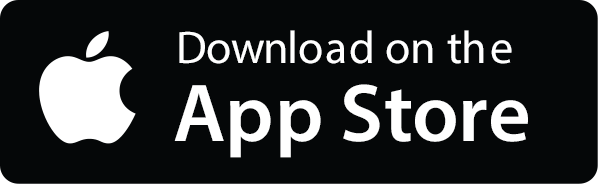
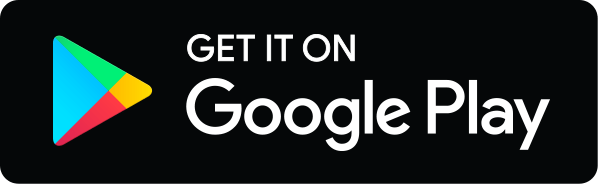