Chapter 20 Cerebellum
Cerebellum means “little brain,” and in a real sense, it is: it accounts for only about 10% of the mass of the brain, but the cerebellum contains as many neurons as all the rest of the central nervous system (CNS) combined. This semidetached mass of neural tissue covers most of the posterior surface of the brainstem, tethered there by three pairs of fiber bundles called cerebellar peduncles. Sensory inputs of virtually every description find their way to the uniquely structured cortex of the cerebellum, which in turn projects (via a set of deep cerebellar nuclei) to various sites in the brainstem and thalamus. Although the cerebellum is extensively concerned with the processing of sensory information, and although it has few ways to influence motor neurons directly, it is considered part of the motor system because cerebellar damage results in abnormalities of equilibrium, postural control, and coordination of voluntary movements.
The Cerebellum Can Be Divided into Both Transverse and Longitudinal Zones
The outside of the cerebellum has a banded appearance, as though its surface were folded like an accordion (Figs. 20-1 and 20-2). This folding is a successful device for increasing the cerebellar surface area; by some estimates, if the cortex could be unfolded into a flat sheet, it would be more than 1 meter long (Fig. 20-3). Deep fissures, most easily seen in sagittal sections (Fig. 20-2E), indent the cerebellar surface. Smaller creases indent the walls of these deep fissures, with the result that the entire cerebellar surface is made up of cortical ridges called folia,*most of which are transversely oriented; prominent fissures are the basis of common systems of dividing the cerebellum into lobes and lobules. Beneath the cortex is a mass of white matter, the medullary center of the cerebellum, which is composed of fibers going to or coming from the cerebellar cortex.
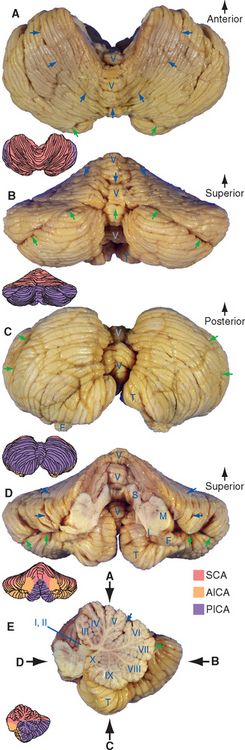
Figure 20-2 Gross anatomy and blood supply of the cerebellum, seen from above (A), behind (B), below (C), in front (D), and hemisected (E). The primary fissure, marking the division between the anterior and posterior lobes, is indicated by blue arrows. The horizontal fissure, which prominently subdivides the posterior lobe, is indicated by green arrows. In the hemisected view (E) the depth of many of the cerebellar fissures is apparent; these are used to divide the vermis into a number of lobules, commonly referred to by roman numerals. The same fissures continue laterally and divide up the cerebellar hemispheres (see also Fig. 20-4 and Table 20-1). Vermal lobules I and II are thin plates of cerebellar tissue (the lingula) that cover the roof of the rostral fourth ventricle; vermal lobule X is the nodulus. AICA, anterior inferior cerebellar artery; F, flocculus; I, M, and S, inferior, middle, and superior cerebellar peduncles; PICA, posterior inferior cerebellar artery; SCA, superior cerebellar artery; T, tonsil; V, vermis.
(Modified from Nolte J, Angevine JB Jr: The human brain in photographs and diagrams, ed 3, St. Louis, 2007, Mosby.)

Figure 20-3 What the human cerebellar cortex would look like if it could be peeled off the surface of the cerebellum and laid out as a flat sheet. The deep notches at the bottom indicate that some of the transverse bands of cerebellar cortex (e.g., the flocculus and nodulus; see Fig. 2-11D) lose their continuity during development. F, flocculus; L, lingula; N, nodulus; T, tonsil; U, uvula.
(Redrawn from Braitenberg V, Atwood RP: J Comp Neurol 109:1, 1958.)
Transverse Fissures Divide the Cerebellum into Lobes
The first fissure to appear during development is the posterolateral fissure, which separates the flocculonodular lobe from the body of the cerebellum (corpus cerebelli). In humans, the body of the cerebellum is by far the larger of the two, and the posterolateral fissure is so deep that the flocculus of each side is almost pinched off from the rest of the cerebellum (Figs. 20-1 and 20-2D). The primary fissure, a prominent landmark in midsagittal sections of the cerebellum, subdivides the body of the cerebellum into anterior and posterior lobes.
Functional Connections Divide the Cerebellum into Longitudinal Zones
The cerebellum can also be divided into longitudinal zones, perpendicular to the fissures, which cut across the anterior, posterior, and flocculonodular lobes (see Fig. 20-4). The most medial zone, straddling the midline, is the vermis (Latin for “worm”). On either side of the vermis is a large cerebellar hemisphere. Each hemisphere is subdivided into a medial hemisphere, a longitudinal strip adjacent to the vermis (sometimes called the intermediate or paravermal zone), and a larger lateral hemisphere. The vermis is fairly clearly set off from the hemispheres on the inferior surface of the cerebellum* (Fig. 20-2C), but other longitudinal lines of separation are not as obvious from the outside (Fig. 20-2A). The demarcation into longitudinal zones is based primarily on patterns of connections and on the functional differences that result, as described shortly; cerebellar cortex has the same structure everywhere and is smoothly continuous from one hemisphere across the midline to the other.
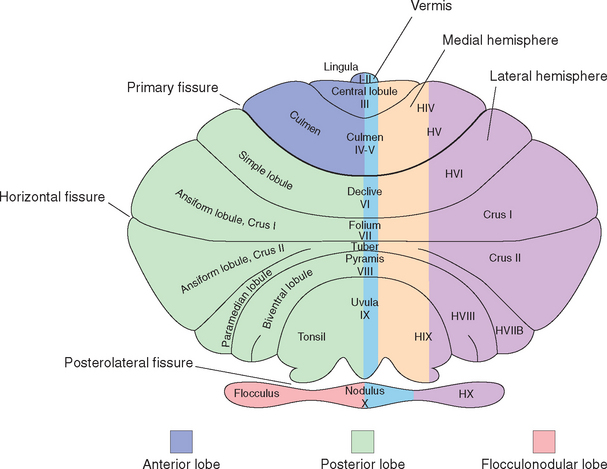
Figure 20-4 Cerebellar terminology on a schematic cerebellum, projected as though it were flattened out with its vermis in one plane (compare with Fig. 20-2). The general division into transversely oriented lobes is indicated on the left side of the diagram, and the division into longitudinal zones is indicated on the right. Also included is terminology for the various subdivisions of the vermis and lobules of the hemispheres. On the left side of the diagram are terms classically used for human cerebellar hemispheres. In the middle, both classic names and roman numerals are indicated for the vermis. On the right side is the roman numeral–based system more commonly used today for the hemispheres. This consists mostly of a series of lateral extensions of the vermal numbers, with an H added to signify hemisphere. The exceptions are crus I and crus II; these are parts of lobule HVII that are greatly expanded in the human cerebellum.
(Drawing modified from Larsell O: Anatomy of the nervous system, ed 2, New York, 1951, Appleton-Century-Crofts. Terminology based on Schmahmann JD et al: Neuro Image 10:233, 1999.)
The fissures that carve the cerebellum into lobules and folia are continuous across the midline during development, so each transverse wedge of cerebellum has a vermal portion and a more lateral portion. For example, the nodulus is the vermal portion of the flocculonodular lobe and continues laterally into the flocculus. The tonsils are the hemispheral portions just across the posterolateral fissure from the flocculi; appropriately enough, their vermal continuation is the uvula. An assortment of exotic names is applied to the lobules and the vermal areas of the corpus cerebelli (Table 20-1; Fig. 20-4), and a roman numeral system is commonly used as well; for the most part, however, these names and numbers are of limited utility in clinical settings. (One exception is the tonsil. Because this is the part of the cerebellum adjacent to the foramen magnum, expanding masses in the posterior fossa can cause tonsillar herniation and compression of the medulla [see Fig. 4-19D].)
Three Peduncles Convey the Inputs and Outputs of Each Half of the Cerebellum
The cerebellum is attached to the brainstem by three substantial peduncles on each side (Figs. 20-5 and 20-6). The inferior cerebellar peduncle, composed mainly of afferents to the cerebellum from the spinal cord and brainstem, has two parts. Most of it is the restiform (“ropelike”) body, which ascends through the rostral medulla, growing larger as it accumulates cerebellar afferents. At the pontomedullary junction it turns dorsally toward the cerebellum, and additional fibers travel over its medial surface as the juxtarestiform body (see Fig. 15-6) interconnecting the cerebellum and vestibular nuclei. The middle cerebellar peduncle (or brachium pontis—the “arm of the pons”), the largest of the three, emerges laterally from the basal pons. It is composed virtually exclusively of afferents to the cerebellum from the pontine nuclei of the contralateral side. The superior cerebellar peduncle (or brachium conjunctivum*—the “joined-together arm,” named for its decussation; see Figs. 11-11, 11-13, 11-14, 11-16, 11-17, and 20-22) contains the major efferent pathways from the cerebellum to the red nucleus and thalamus.
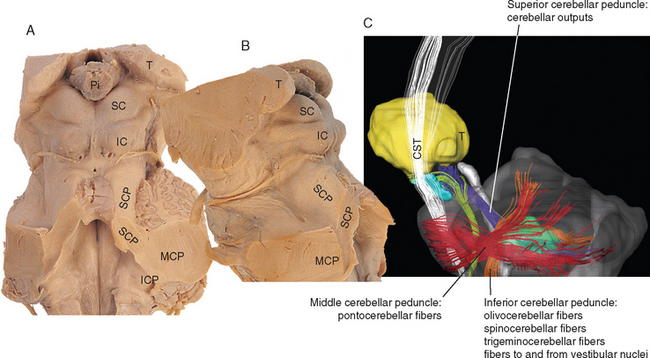
Figure 20-5 Dorsal (A) and lateral (B) views of the severed cerebellar peduncles. C, Diffusion tensor image of a lateral view of the brainstem, showing the courses and an overview of the contents of the cerebellar peduncles (for more details, see Table 20-2). CST, corticospinal tract; IC, inferior colliculus; ICP, inferior cerebellar peduncle; MCP, middle cerebellar peduncle; Pi, pineal gland; SC, superior colliculus; SCP, superior cerebellar peduncle; T, thalamus.
(C, from Mori S et al: MRI atlas of human white matter, Amsterdam, 2005, Elsevier.)
Deep Nuclei Are Embedded in the Cerebellar White Matter
A series of deep cerebellar nuclei is buried in the medullary center of each side of the cerebellum (Fig. 20-7). The most lateral is the dentate nucleus, a crumpled sheet of cells that looks strikingly like the inferior olivary nucleus. Most of the fibers in the superior cerebellar peduncle originate from the dentate nucleus and emerge from its medially facing mouth, or hilus (Fig. 20-6C). Medial to the dentate nucleus is the interposed nucleus, which in humans has two distinct subdivisions—an anterior and lateral emboliform nucleus, and a posterior and medial globose nucleus. (Because of their positions, the em-boliform and globose nuclei are sometimes referred to as the anterior and posterior interposed nuclei, respectively.) Finally, the most medial of the deep cerebellar nuclei is the fastigial nucleus.
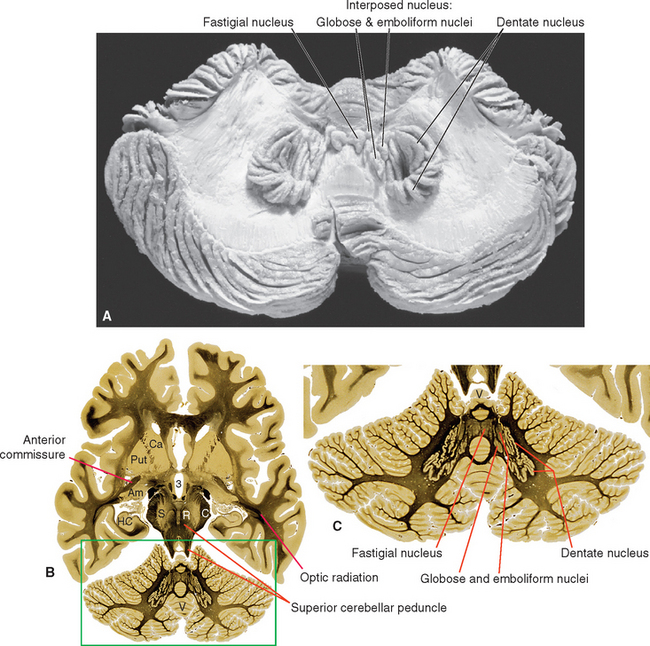
Figure 20-7 Deep cerebellar nuclei. A, A beautiful dissection demonstrating the deep nuclei of a human cerebellum. B, The deep nuclei as seen in an axial section slightly superior to that shown in Figure 20-6C (enlarged in C). 3, third ventricle; Am, amygdala; C, cerebral peduncle; Ca, caudate nucleus; HC, hippocampus; Put, putamen; R, red nucleus; S, substantia nigra; V, vermis.
(A, from Gluhbegovic N: J Anat 137:396, 1983.)
All Parts of the Cerebellum Share Common Organizational Principles
The cerebellum as a whole has a straightforward organization (Fig. 20-8): inputs arrive at the cerebellar cortex, the cortex works its magic and projects to the deep nuclei, and the deep nuclei provide the cerebellar output. Cerebellar cortex has a systematic, strikingly regular structure (see Figs. 20-9 and 20-10). The uniformity of this organization suggests that all regions of the cerebellum perform the same fundamental operation and that distinctive inputs and outputs of different regions allow the cerebellum to be involved in multiple neural functions.
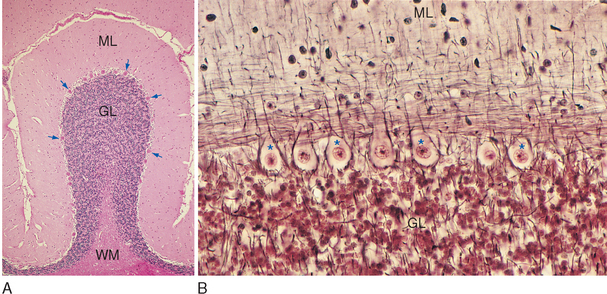
Figure 20-9 Light micrographs of cerebellar cortex. A, Cross section of a single folium from a human cerebellum (stained with hematoxylin and eosin). The molecular layer (ML) adjacent to the pial surface of the cerebellum contains relatively few neurons and is the main site of synaptic interactions between cerebellar interneurons and Purkinje cells. The granular layer (GL), adjacent to the central white matter (WM) of the cerebellum, contains the tightly packed cell bodies of tiny granule cells (see Fig. 20-11). Between the molecular and granular layers is a single layer of large Purkinje cells (arrows). B, Higher-magnification view of the Purkinje cell layer of monkey cerebellar cortex (Bodian silver stain), showing the large cell bodies of these neurons (some indicated by *) surrounded by silver-stained processes of cerebellar interneurons. Purkinje cell dendrites project into the molecular layer, where they are contacted by parallel fibers whose parent axons arise in the granular layer.
(Courtesy Dr. Nathaniel T. McMullen, University of Arizona College of Medicine.)
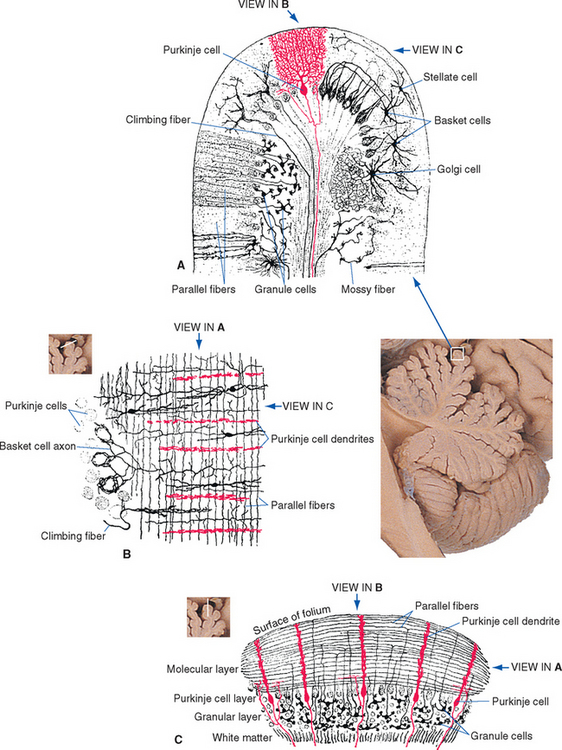
Figure 20-10 Composite drawings of Golgi-stained cerebellar neurons, from sections cut in three nearly orthogonal planes. A, Transverse section cut perpendicular to the long axis of a folium, showing mossy fibers, climbing fibers, and the major neuronal cell types of the cerebellar cortex. The elements of the principal circuit through the cerebellar cortex (see Fig. 20-14A) can be seen clearly. Other cell types in the cerebellar cortex: basket cells, whose dendrites spread out in the molecular layer and whose axons branch to enclose the cell bodies of a series of Purkinje cells; stellate cells, whose axons and dendrites all ramify in the molecular layer; and Golgi cells, whose dendrites spread out in the molecular layer and whose axons end on granule cell dendrites. B, A section parallel to the long axis of a folium, cut at an oblique angle so that it passes through the molecular layer on the right and the Purkinje cell layer on the left. This view demonstrates how the parallel fibers and the flattened dendritic trees of Purkinje cells are oriented perpendicular to each other. C, Another section parallel to the long axis of a folium, this time perpendicular to its surface, to demonstrate the layers of the cerebellar cortex.
(Modified from Ramón y Cajal S: Histologie du système nerveux de l’homme et des vertébrés, Paris, 1909, 1911, Maloine.)
Inputs Reach the Cerebellar Cortex as Mossy and Climbing Fibers
The cortex of the cerebellum has a uniform and fairly simple three-layered structure (Fig. 20-9). The most superficial layer is the molecular layer, consisting mainly of the axons and dendrites of various cerebellar neurons. Deep to the molecular layer is a single layer of large neurons called Purkinje cells. Finally, adjacent to the medullary center is the granular layer, composed mainly of small granule cells arranged in a stratum many cells thick. The molecular and granular layers also contain characteristic types of interneurons (seeFigs. 20-10 and 20-14B), but the fundamental circuitry of the cerebellar cortex can be described in terms of Purkinje cells, granule cells, and the afferents to the cortex (see Fig. 20-14A).
Purkinje cells are the only neurons whose axons leave the cerebellar cortex. They are, in addition, among the most anatomically distinctive neurons found in the nervous system. Each Purkinje cell has an intricate, extensive dendritic tree (see Fig. 1-4A) that is flattened out in a plane perpendicular to the long axis of the folium in which it resides (Fig. 20-10). Each granule cell (Fig. 20-11) sends its axon into the molecular layer, where it bifurcates to form a fine, unmyelinated parallel fiber (Fig. 20-12) that extends for about 5 mm along the long axis of the folium. In its course, each parallel fiber passes through and synapses on the dendritic trees of a succession of Purkinje cells (as many as 500 of them). Each of us is estimated to have an incredible 1011 granule cells—half the neurons in the CNS—and each of our 25 million Purkinje cells receives synapses from perhaps 105 of them.
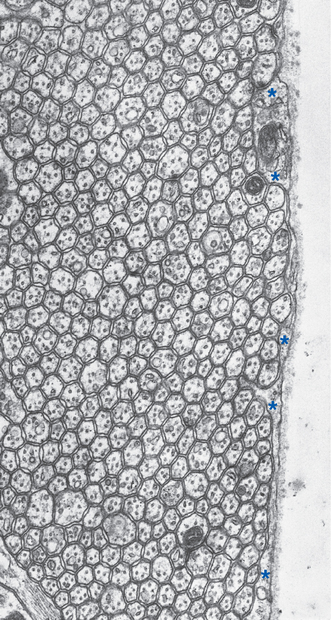
Figure 20-12 Regular array of parallel fibers in the molecular layer of rat cerebellar cortex (same plane as in Fig. 20-10A). Only a thin glial covering (*) separates some parallel fibers from the surface of the cerebellum. Despite the tiny size of these unmyelinated axons (about 0.2 μm in diameter), each contains the usual microtubules and neurofilaments.
(From Pannese E: Neurocytology: fine structure of neurons, nerve processes, and neuroglial cells, New York, 1994, Thieme Medical Publishers.)
The cerebellum receives its share of diffuse modulatory inputs from places such as the locus ceruleus and the raphe nuclei (see Figs. 11-24 and 11-27). There are also two distinctive sets of afferent fibers to the cerebellar cortex: climbing fibers and mossy fibers. A single climbing fiber ends directly on each Purkinje cell,winding around its dendrites like ivy climbing a trellis (Fig. 20-13); in the process, it makes tens of thousands of excitatory synapses, and collectively, these form the most powerful excitatory input in the nervous system. All these climbing fibers arise in the contralateral inferior olivary nucleus. All the rest of the afferents to the cerebellar cortex are mossy fibers. Mossy fibers end on the dendrites of granule cells, so this is a less direct route to the Purkinje cells (mossy fiber→granule cell→parallel fiber→aPurkinje cell).
Purkinje Cells of the Cerebellar Cortex Project to the Deep Nuclei
Although Purkinje cell axons are the only route out of the cerebellar cortex, few of them leave the cerebellum itself. Rather, they project to the deep nuclei, which in turn give rise to the cerebellar output (Fig. 20-8). However, the deep nuclei are not just simple relay stations (Fig. 20-14C). For example, climbing fibers and many mossy fibers send collateral branches to the deep nuclei. It has been suggested that these inputs provide a tonic excitatory drive to neurons of the deep nuclei, and that inhibitory projections from Purkinje cells then modulate the firing rates of these neurons. In addition to giving rise to axons that leave the cerebellum, the deep nuclei project back to the same areas of cerebellar cortex from which they receive Purkinje axons. The functional implications of these additional connections are not fully understood, but they make it less surprising that the consequences of cerebellar damage are much more severe and long lasting when the deep nuclei are included in the lesion.
One Side of the Cerebellum Affects the Ipsilateral Side of the Body
There are many crossings of the midline in the various circuits interconnecting the cerebellum and other parts of the CNS, ultimately forming the basis for the fact that one cerebral hemisphere controls skeletal muscle of the contralateral limbs, but one half of the cerebellum influences movements of the ipsilateral limbs (see Figs. 3-33 and 20-23). For example, pontine nuclei receive inputs from the ipsilateral cerebral cortex and project to the contralateral half of the cerebellum (see Fig. 20-16), and one half of the cerebellum projects to the contralateral thalamus (see Fig. 20-21).
< div class='tao-gold-member'>
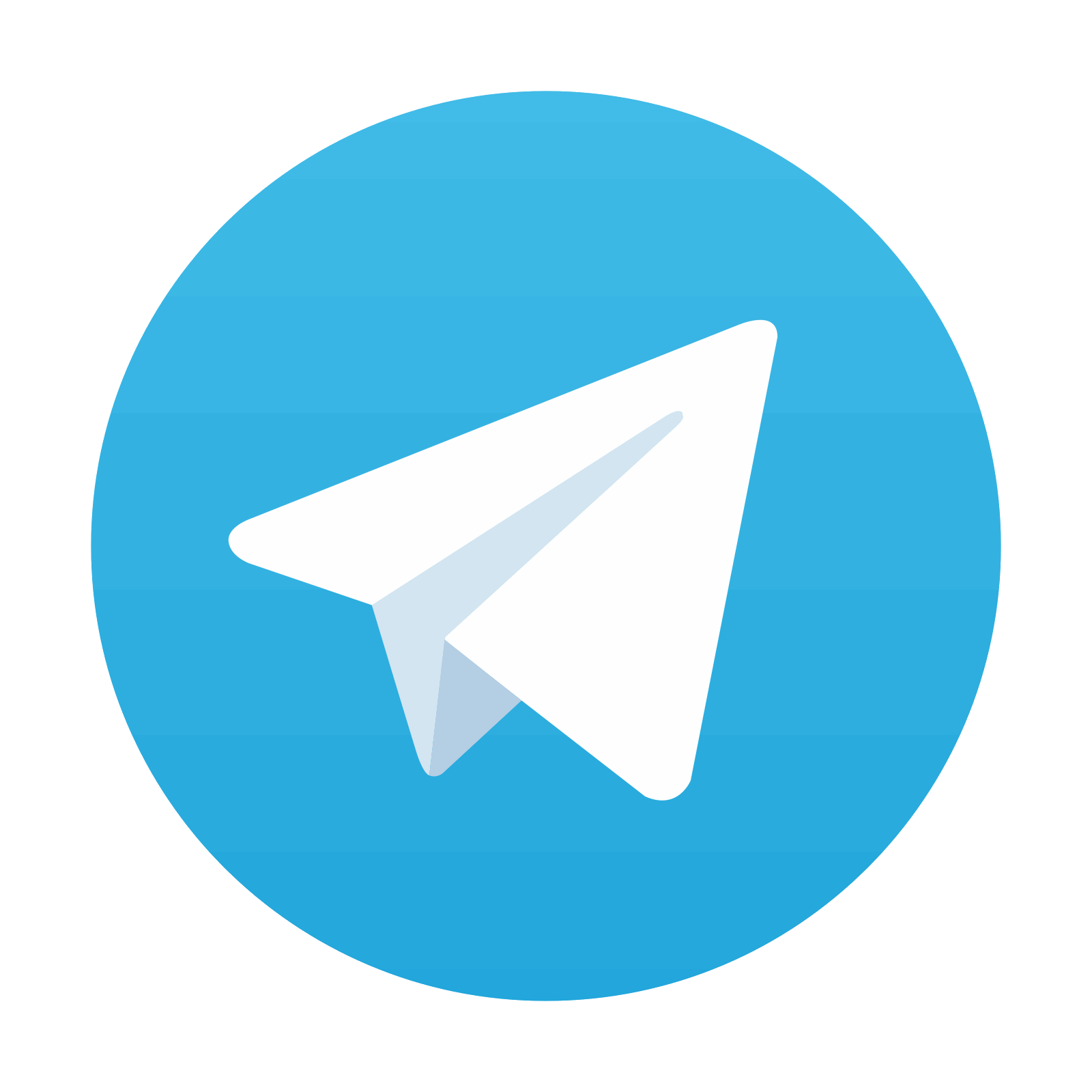
Stay updated, free articles. Join our Telegram channel

Full access? Get Clinical Tree
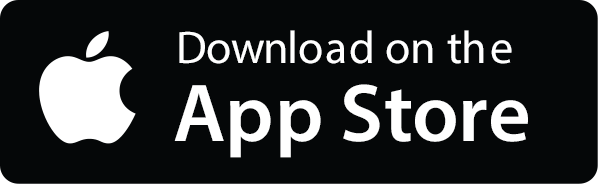
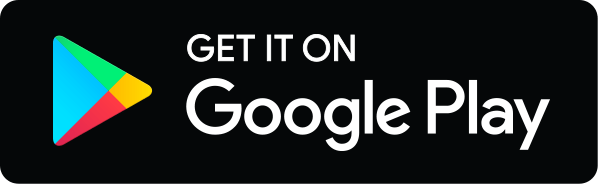