Disturbances of Vision: Introduction
The importance of the visual system is attested by the magnitude of its representation in the central nervous system (CNS). A large part of the cerebrum is committed to vision, including the visual control of movement and the perception of printed words, and the form and color of objects. The optic nerve, which is a CNS structure, contains more than a million fibers (compared to 50,000 in the auditory nerve). The visual system also has special significance in that study of this system has greatly advanced our knowledge of both the organization of all sensory neuronal systems and the relation of perception to cognition. Indeed, we know more about vision than about any other sensory function. Furthermore, the eyes, because of their diverse composition of epithelial, vascular, neural, and pigmentary tissues, are virtually a medical microcosm, susceptible to many diseases, and its tissues are available for inspection through a transparent medium.
Impairment of visual function, expressed as defects in acuity and alterations of visual fields, obviously stands as the most important symptom of eye disease. A number of terms are commonly used to describe visual loss. Amaurosis is a general term that refers to partial or complete loss of sight. Amblyopia refers to any monocular deficit in vision that occurs in the presence of normal ocular structures. A major cause of amblyopia is the suppression by the brain of vision from one eye during early childhood caused by either strabismus, anisometropia (a significant difference in refractive error), or by media opacities. Nyctalopia is the term for poor twilight or night vision and is associated with extreme myopia, cataracts, vitamin A deficiency, retinitis pigmentosa, and, often, color blindness. There are also a number of positive visual symptoms (phosphenes, migrainous scintillations, visual illusions, and hallucinations), but they are generally less significant than symptoms of visual loss. Irritation, redness, photophobia, pain, diplopia and strabismus, changes in pupillary size, and drooping or closure of the eyelids are other major ocular symptoms and signs. Impairment of vision may be unilateral or bilateral, sudden or gradual, episodic or enduring.
The common causes of failing eyesight vary with age. In infancy, congenital defects, retinopathy of prematurity, severe myopia, hypoplasia of the optic nerve, optic pits, and coloboma are the main causes. In childhood and adolescence, nearsightedness or myopia, and amblyopia as a result of strabismus are the usual causes (see Chap. 14), although a pigmentary retinopathy or a retinal, optic nerve, or suprasellar tumor may also begin at this age. In middle age, usually beginning in the fifth decade, a progressive loss of accommodation (presbyopia) is almost invariable (at this age, half or more of the amplitude of accommodative power is lost and must be replaced by plus lenses). Still later in life, cataracts, glaucoma, retinal vascular occlusion and detachments, macular degeneration, and tumor, unilateral or bilateral, are the most frequent causes of visual impairment.
As a rule, episodic visual loss in early adult life, often hemianopic, is the result of migraine. The other important cause of transient (weeks) monocular visual loss in this age period is optic neuritis, often a harbinger of multiple sclerosis. Amaurosis in the child or young adult may also be caused by systemic lupus erythematosus and the related antiphospholipid syndrome, or by migraine, or there may be no discernible cause. Later in life, transient monocular blindness, or amaurosis fugax, lasting minutes to hours is more common; it is caused by vascular disease, particularly stenosis of the ipsilateral carotid artery. Table 13-1 lists the main causes of episodic monocular visual loss. Of course, at any age, diseases of the retina and of other components of the ocular apparatus are important causes of progressive visual loss, and the problem may at first be transient.
Adolescence and young adulthood |
Migraine |
Optic neuritis |
Papilledema |
Antiphospholipid antibody syndrome and systemic lupus erythematosus |
Early tumor compression of the optic nerve |
Takayasu aortic arteritis |
Viral neuroretinitis |
Idiopathic |
Adulthood |
Carotid stenosis or dissection |
Embolism to the retina |
Intrinsic central retinal artery atherosclerotic disease |
Temporal arteritis (generally over age 55) |
Glaucoma |
Papilledema |
Approach to the Problem of Visual Loss
In the investigation of a disturbance of vision, one inquires as to what the patient means when he claims that he cannot see properly, for the disturbance in question may vary from near- or farsightedness to diplopia, partial syncope, dizziness, or a hemianopia. Fortunately, the patient’s statement can be checked by the measurement of visual acuity, which is the single most important part of the ocular examination. Inspection of the refractive media and the optic fundi—especially the macular region—the testing of pupillary reflexes, color vision, and the plotting of visual fields complete this part of the examination. Examination of the eye movements is also essential, particularly if amblyopia predicated on an early life strabismus is suspected, as discussed in Chap. 14.
In the measurement of distance visual acuity the Snellen chart, which contains letters (or numbers or pictures) arranged in rows of decreasing size, is used (Fig. 13-1A). Each eye is tested separately and, if glasses are required, glasses for distance, not reading glasses, should be worn. The letter at the top of the chart subtends 5 min of an arc at a distance of 200 ft (or roughly 60 m). The patient follows rows of letters that can normally be read at lesser distances. Acuity is reported as a nonmathematical fraction that represents the patient’s ability compared to that of a person with normal distance vision. Thus, if the patient can read only the top letter, which would be normally be visible at 200 feet, the acuity is expressed as 20/200, or if the distance is measured in meters rather than feet, as 6/60. If the patient’s eyesight is normal, the visual acuity will equal 20/20, or 6/6, corresponding to the eighth line on most charts. Many persons, especially during youth, can read at 20 ft the line that can normally be read at 15 ft from the chart (20/15) and hence have better than “normal” vision.
For bedside testing, a “near card” or newsprint held 14 in from the eyes can be used, and the results expressed in a distance equivalent as if a distance chart had been used (Fig. 13-1B). Here, the Jaeger system is used (J1 is “normal” vision, corresponding to the line 20/25 on a Snellen chart, J5 to 20/50, J10 to 20/100, J16 to 20/200, and so on). In young children, acuity can be estimated by having them mimic the examiner’s finger movements at varying distances or having them recognize and pick up objects of different sizes from varying distances. The Teller acuity cards test a child’s preference (and hence ability) to view cards with increasingly fine stripes. In most jurisdictions, a corrected acuity of 20/40 or better in one eye is required to obtain and retain a driver’s license.
If the visual acuity (with glasses) is less than 20/20, either the refractive error has not been properly corrected or there is some other reason for diminished acuity. The possibility of a nonrefractive error can usually be ruled out if the patient can read the 20/20 line (not the near card) through a pinhole in a cardboard held in front of the eye. The pinhole permits a narrow shaft of light to fall on the fovea (the area of greatest visual acuity) without distortion by the curvature of the lens; this eliminates the eye’s optical system, thereby testing the macula alone, and should give an acuity of 20/20 if the structures of the ocular media (cornea, lens, aqueous and vitreous humors) are clear.
Light entering the eye is focused by the biconvex lens onto the outer layer of the retina. Consequently, the cornea, fluid of the anterior chamber, lens, vitreous, and retina itself must be transparent. The clarity of these media can be determined ophthalmoscopically, and a complete examination requires that the pupil be dilated to at least 6 mm in diameter. This is accomplished by instilling two drops of 2.5 percent phenylephrine and/or 0.5 to 1.0 percent tropicamide in each eye after the visual acuity has been measured, the pupillary response recorded, and the intraocular pressure estimated. In elderly persons, lower concentrations of these mydriatics should be used. The mydriatic action of phenylephrine lasts for 3 to 6 h. Rarely, an attack of angle-closure glaucoma (manifesting itself by diminished vision, ocular pain, nausea, and vomiting) may be precipitated by pharmacologic pupillary dilatation; this requires the administration of pilocarpine to the eye and the immediate attention of an ophthalmologist. It is advisable to have access to pilocarpine if the pupils are to be dilated.
By looking through a high-plus lens of the direct ophthalmoscope from a distance of 6 to 12 in, the examiner can visualize opacities in the refractive media; by adjusting the lenses from a high-plus to a zero or minus setting, it is possible to “depth-focus” from the cornea to the retina. Depending on the refractive error of the examiner, lenticular opacities are best seen within the range of +20 to +12. The retina comes into focus with +1 to -1 lenses. The illuminated pupil appears as a red circular structure (red reflex), the color being provided by blood in the capillaries of the choroid layer. If all the refractile media are clear, reduced vision that is uncorrectable by glasses is caused by a defect in the macula, the optic nerve, or parts of the brain with which they are connected. The main limit of direct ophthalmoscopy is its inability to visualize lesions in the retina that lie anterior to the equator of the globe; these are seen only by the indirect method.
Nonneurologic Causes of Reduced Vision
It is hardly possible within the confines of this chapter to describe all the causes of opacification of the refractive media. Those with the most important medical or neurologic implications are briefly commented upon. Although changes in the refractive media do not involve neural tissue primarily, certain ones assume importance because they are associated with neurologic disease and provide clues to its presence.
In the cornea, the most common abnormality that reduces vision is scarring caused by trauma and infection. Ulceration and subsequent fibrosis may occur following recurrent herpes simplex, herpes zoster, and trachomatous infections of the cornea, or with certain mucocutaneous-ocular syndromes (Stevens-Johnson, Reiter). Hypercalcemia secondary to sarcoidosis, hyperparathyroidism, and vitamin D intoxication or milk-alkali syndrome may give rise to precipitates of calcium phosphate and carbonate beneath the corneal epithelium, primarily in a plane corresponding to the interpalpebral fissure—so-called band keratopathy. Other causes of corneal opacity include chronic uveitis, interstitial keratitis, corneal edema, lattice corneal dystrophy (amyloid deposition), and long-standing glaucoma. Polysaccharides are deposited in the corneas in some of the mucopolysaccharidoses (see Chap. 37), and copper is deposited in the Descemet membrane in hepatolenticular degeneration (Kayser-Fleischer ring). Crystal deposits may be observed in multiple myeloma and cryoglobulinemia. The corneas are also diffusely clouded in certain lysosomal storage diseases (see Chap. 37). Arcus senilis occurring at an early age (because of hyperlipidemia), sometimes combined with yellow lipid deposits in the eyelids and periorbital skin (xanthelasma), serves as a marker of atheromatous vascular disease.
In the anterior chamber of the eye, a common problem is impediment to the outflow of aqueous fluid, associated with excavation of the optic disc and visual loss, i.e., glaucoma. In more than 90 percent of cases (of the open-angle type), the cause of this syndrome is unknown and a genetic factor is suspected. The drainage channels in this type appear normal. In approximately 5 percent of cases, the angle between iris and the peripheral cornea is narrow and blocked when the pupil is dilated (angle-closure glaucoma). In the remaining cases, the condition is a result of some disease process that blocks outflow channels—inflammatory debris of uveitis, red blood cells from hemorrhage in the anterior chamber (hyphema), new formation of vessels and connective tissue on the surface of the iris (rubeosis iridis), a relatively infrequent complication of ocular ischemia secondary to diabetes mellitus, retinal vein occlusion, or carotid artery occlusion. The visual loss is gradual in open-angle glaucoma and the eye looks normal, unlike the red, painful eye of angle-closure glaucoma that was described above in reference to pharmacologic dilation of the pupil to facilitate fundoscopy. However, some cases of open-angle glaucoma may progress to rapid loss of vision.
Intraocular pressures that are persistently above 20 mm Hg may damage the optic nerve over time. This may be manifest first as an arcuate defect in the upper or lower nasal field or as a paracentral field defect, which, if untreated, may proceed to blindness. The classic finding in glaucoma is the Bjerrum field defect, consisting of an arcuate scotoma extending from the blind spot and sweeping around the macula to end in a horizontal line at the nasal equator. Other characteristic glaucomatous field patterns are winged extensions from the blind spot (Seidel scotoma) and a narrowing of the superior nasal quadrant that may progress to a horizontal edge, corresponding to the horizontal raphe of the retina (nasal step). The damage is at the optic nerve head, the optic disc appearing excavated and any pallor that is present extends only to the rim of the disc and not beyond, thus distinguishing it from other optic neuropathies. Elongation of the optic cup in the vertical axis is typical. It is now appreciated that elevated intraocular pressure is only a concurrent finding and a risk factor for glaucoma and that optic damage may be seen in patients with near normal pressure. This represents a major revision of the previous view that pressure was the elemental cause of damage in glaucoma.
In the lens, cataract formation is the most common and mundane abnormality. The cause of the common type in the elderly is unknown. The “sugar cataract” of diabetes mellitus is the result of sustained high levels of blood glucose, which is changed in the lens to sorbitol, the accumulation of which leads to a high osmotic gradient with swelling and disruption of the lens fibers. Galactosemia is a much rarer cause, but the mechanism of cataract formation is similar, i.e., the accumulation of dulcitol in the lens. In hypoparathyroidism, lowering of the concentration of calcium in the aqueous humor is in some way responsible for the opacification of newly forming superficial lens fibers. Prolonged high doses of corticosteroids, as well as radiation therapy, induce lenticular opacities in some patients. Down syndrome and oculocerebrorenal syndrome (see Chap. 38), spinocerebellar ataxia with oligophrenia (see Chap. 39), and certain dermatologic syndromes (atopic dermatitis, congenital ichthyosis, incontinentia pigmenti) are also accompanied by lenticular opacities. Myotonic dystrophy (see Chap. 48) and, rarely, Wilson disease (see Chap. 37) are associated with special types of cataract. Subluxation of the lens, the result of weakening of its zonular ligaments, occurs in syphilis, Marfan syndrome (upward displacement), and homocystinuria (downward displacement).
In the vitreous humor, hemorrhage may occur from rupture of a ciliary or retinal vessel. On ophthalmoscopic examination, the hemorrhage appears as a diffuse haziness of part or all of the vitreous or, if the blood is between the retina and the vitreous and displaces the latter rather than mixing with it, takes the form of a sharply defined clot. The common cause is rupture of newly formed vessels of proliferative retinopathy in patients with diabetes mellitus, but there are many others including orbital or cranial trauma, rupture of an intracranial aneurysm or arteriovenous malformation with high intracranial pressure, retinal vein occlusion, sickle cell disease, age-related macular degeneration (ARMD), and retinal tears, in which the hemorrhage breaks through the internal limiting membrane of the retina. The most common vitreous opacities are benign “floaters” caused by the condensation of vitreous collagen fibers, which appear as darting gray flecks or threads with changes in the position of the eyes; they may be annoying or even alarming until the person stops looking for them.
A sudden burst of flashing lights associated with an increase in floaters may mark the onset of retinal detachment. Patients complaining of bright flashes and spots in vision should be examined with the indirect ophthalmoscope to rule out tears, holes, or detachments of the vitreous or retina. Another common occurrence with advancing age is shrinkage of the vitreous humor and retraction from the retina, causing persistent streaks of light, usually in the periphery of the visual field. These phosphenes, also known as Moore lightning streaks, had been thought to be quite benign, but they may at times, indicate incipient retinal or vitreous tears or detachment, and their first appearance requires prompt evaluation by an ophthalmologist. They are most prominent on movement of the globe, on closure of the eyelids, at the moment of accommodation, with saccadic eye movements, and with sudden exposure to dark. The vitreous may also be infiltrated by lymphoma originating in the brain; biopsy by planar vitrectomy may be used to establish the diagnosis in those rare instances where the lymphoma is restricted to the eye; its presence can be inferred when there is vitreous cellular infiltration and also a brain lymphoma.
The term uveitis refers to an infective or noninfective inflammatory disease that affects any of the uveal structures (iris, ciliary body, and choroid). According to Bienfang and colleagues, uveitis accounts for 10 percent of all cases of legal blindness in the United States. Infective causes of posterior uveitis (choroidal) are toxoplasmal and cytomegalic inclusion disease, occurring mainly in patients with AIDS and other forms of reduced immune function. Noninfective autoimmune types are also common in the adult. The inflammation may be in the anterior part of the eye or in the posterior part, behind the iris and extending to the retina and choroid. Anterior uveitis is sometimes linked to ankylosing spondylitis and the human leukocyte antigen (HLA) B-27 marker, sarcoidosis, and recurrent meningitis (Vogt-Koyanagi-Harada disease); the posterior forms are associated with sarcoidosis, Behçet disease, and lymphoma.
Retinal diseases, particularly ARMD and diabetic retinopathy, are a more common cause of blindness than are neurologic diseases, as discussed further on, under “Other Diseases of the Retina.”
Neurologic Causes of Reduced Vision
Certain anatomic and physiologic facts are required for an interpretation of the neurologic lesions that affect vision. Visual stimuli entering the eye traverse the inner layers of the retina to reach its outer (posterior) layer, which contains two classes of photoreceptor cells: the flask-shaped cones and the slender rods. The photoreceptors rest on a single layer of pigmented epithelial cells, which form the outermost surface of the retina. The rods and cones and pigmentary epithelium receive their blood supply from the capillaries of the choroid and, to a smaller extent, from the retinal arterioles. The rod cells contain rhodopsin, a conjugated protein in which the chromophore group is a carotenoid akin to vitamin A. The rods function in the perception of visual stimuli in subdued light (twilight or scotopic vision), and the cones are responsible for color discrimination and the perception of stimuli in bright light (photopic vision). Most of the cones are concentrated in the macular region, particularly in its central part, the fovea, and are responsible for the highest level of visual acuity. Traquair described the rapid fall-off of acuity as the distance from the fovea increases as “an island of vision in a sea of blindness.” Specialized pigments in the rods and cones absorb light energy and transform it into electrical signals, which are transmitted to the bipolar cells of the retina and then, in turn, to the superficially (anteriorly) placed neurons, or ganglion cells (Fig. 13-2). There are no ganglion cells in the fovea.
Figure 13-2.
Diagram of the cellular elements of the retina. Light entering the eye anteriorly passes through the full thickness of the retina to reach the rods and cones (first system of retinal neurons). Impulses arising in these cells are transmitted by the bipolar cells (second system of retinal neurons) to the ganglion cell layer. The third system of visual neurons consists of the ganglion cells and their axons, which run uninterruptedly through the optic nerve, chiasm, and optic tracts, synapsing with cells in the lateral geniculate body. (Courtesy of Dr. E.M. Chester.)
The axons of the retinal ganglion cells, as they stream across the inner surface of the retina, pursue an arcuate course. Being unmyelinated, they are not visible, although fluorescein retinography shows a trace of their outlines; an experienced examiner, using a bright light and deep green filter, can visualize them through direct ophthalmoscopy. The axons of ganglion cells are collected in the optic discs and then pass uninterruptedly through the optic nerves, optic chiasm, and optic tracts to synapse in the lateral geniculate nuclei, the superior colliculi, the midbrain pretectum and the suprachiasmatic nucleus of the hypothalamus (Figs. 13-2 and 13-3). The fibers derived from macular cells form a discrete bundle that first occupies the temporal side of the disc and optic nerve and then assumes a more central position within the nerve (papillomacular bundle). These fibers are of smaller caliber than the peripheral optic nerve fibers and appear to be especially sensitive to toxic and metabolic injury. Damage to the papillomacular bundle produces the “cecocentral” scotoma (extending from fixation to the blind spot). It is important to keep in mind that the retinal ganglion cells and their axon extensions are, properly speaking, an exteriorized part of the brain and that their pathologic reactions are the same as in other parts of the CNS.
Figure 13-3.
Diagram showing the effects on the fields of vision produced by lesions at various points along the optic pathway. A. Complete blindness in left eye from an optic nerve lesion. B. The usual effect is a left-junction scotoma in association with a right upper quadrantanopia. A left nasal hemianopia could occur from a lesion at this point but is rare. C. Chiasmatic lesion causing bitemporal hemianopia. D. Right homonymous hemianopia from optic tract lesion. E and F. Right superior and inferior quadrant hemianopia from interruption of visual radiations. G. Right homonymous hemianopia caused by lesion of occipital striate cortex. H. Hemianopia with macular sparing.
In the optic chiasm, the fibers derived from the nasal half of each retina decussate and continue in the optic tract with the uncrossed temporal fibers of the other eye (Figs. 13-3 and 13-4). Thus, interruption of the left optic tract causes a right hemianopic defect in each eye, i.e., a homonymous (left nasal and right temporal) field defect (Fig. 13-3D). In partial tract lesions, the visual defects in the two eyes may not be exactly congruent, as the tract fibers are not evenly admixed. Lesions at the junction of the optic nerve and chiasm, generally compressive in nature, may cause a small contralateral superotemporal quadrantic defect in addition to the expected central scotoma in the ipsilateral eye (“junctional scotoma,” Fig. 13-3B). The finding is explained by the compression of the tract and the nerve on the same side. It had been thought for many decades to result from compression of Wilbrand’s knee, a bundle of fibers that turn back into the contralateral optic nerve before crossing in the chiasm, but it has since been related by Horton that the very existence of the bundle is merely an artifact of fixation in experimental material. We are uncertain if the explanation of this particular configuration of visual field abnormality has a better explanation than the existence of Wilbrand’s knee and are reluctant to discard it.
The optic chiasm lies just above the pituitary gland and also forms part of the anterior wall of the third ventricle; hence the crossing fibers may be compressed from below by a pituitary tumor, a meningioma of the tuberculum sellae, or an aneurysm, and from above by a dilated third ventricle or craniopharyngioma. The resulting field defect is bitemporal (“bitemporal hemianopia”; Fig. 13-3C); if the lesion has an anterior extension to the junction with one optic nerve there is a loss of full-field vision in that eye and a partial loss in the other (“functional scotoma”). Optic tract lesions, in comparison with chiasmatic and optic nerve lesions, are relatively rare and cause a full contralateral hemianopia. In albinism, there is an abnormality of chiasmatic decussation, in which a majority of the fibers cross to the other side. How this relates to the global albinic defect in pigmented epithelium is not known.
Approximately 80 percent of the fibers of the optic tract terminate in the lateral geniculate body, a thalamic nucleus, and synapse with the six laminae of its neurons. Three of these laminae (1, 4, 6), which constitute the large dorsal nucleus, receive crossed (nasal) fibers from the contralateral eye, and three (2, 3, 5) receive uncrossed (temporal) fibers from the ipsilateral eye. Selective occlusion of either component of the dual blood supply to the lateral geniculate, consisting of the anterior and posterior choroidal arteries, is infrequent but when it does occur, produces a characteristic “multiple sectoral field defect”; a quadruple sectoranopia, meaning homonymous sectoral defects in the upper and lower quadrants of both eyes due to occlusion of the anterior choroidal artery, and two horizontal sectoranopias with occlusion of the posterior (lateral) choroidal artery. The geniculate cells project to the visual (striate) cortex of the occipital lobe, also called area 17 (Brodmann classification) or V1 (Figs. 13-4 and 13-5).
Figure 13-5.
Diagrammatic depiction of the retinal projections, showing the disproportionately large representation of the macula in the lateral geniculate nucleus and visual (striate) cortex. (Redrawn by permission from Barr ML, Kiernan J: The Human Nervous System, 4th ed. Philadelphia, Lippincott, 1983.)
Other optic tract fibers terminate in the pretectum and innervate both Edinger-Westphal nuclei, which subserve pupillary constriction and accommodation (see Fig. 14-8). A small group of fibers terminate in the suprachiasmatic nuclei in animals and presumably also in humans. These anatomic details explain several useful clinical signs. If there is a lesion in one optic nerve, a light stimulus to the affected eye will have no effect on the pupil of either eye, although the ipsilateral pupil will still constrict consensually, i.e., in response to a light stimulus from the normal eye. This phenomenon is termed an afferent pupillary defect.
In their course through the temporal lobes, the fibers from the lower and upper quadrants of each retina diverge. The lower ones arch around the anterior pole of the temporal horn of the lateral ventricle before turning posteriorly; the upper ones follow a more direct path through the white matter of the uppermost part of the temporal lobe (Fig. 13-4), and probably of the adjacent interior parietal lobe. Both groups of fibers merge posteriorly at the internal sagittal stratum. For these reasons, incomplete lesions of the geniculocalcarine pathways (optic radiations) cause visual field defects that are partial and often not fully congruent (Fig. 13-3E and F).
It is in Brodmann area 17, embedded in the medial lip of the occipital pole, that cortical processing of the retinogeniculate projections occurs. The receptive neurons are arranged in columns, some of which are activated by edges and forms and others by moving stimuli or by color. The neurons for each eye are grouped together and have concentric, center-surround receptive fields. The deep neurons of area 17 project to the secondary and tertiary visual areas of the occipitotemporal cortex of the same and opposite cerebral hemispheres and also to other multisensory parietal and temporal cortices. Several of these extrastriate connections are just now being identified. Separate visual systems are utilized in the perception of motion, color, stereopsis, contour, and depth perception. Conceptually, the flow of secondary visual processing can be divided into a ventral stream, which carries predominantly spatial information to the parietal lobe (“the where”) and a dorsal stream, which carries shape and color information to the temporal lobe (“the what”) as articulated by Levine and colleagues. The classic studies of Hubel and Wiesel have elucidated much of this visual cortical anatomy and physiology and their papers, for which they were awarded the Nobel Prize, should be consulted for a fuller appreciation of the organization of the visual cortex.
The normal development of the connections described above requires that the visual system be activated at each of several critical periods of development. The early deprivation of vision in one eye causes a failure of development of the geniculate and cortical receptive fields of that eye. Moreover, in this circumstance the cortical receptive fields of the seeing eye become abnormally large and usurps the monocular dominance columns of the blind eye (Hubel and Wiesel). In children with a congenital cataract, the eye will remain amblyopic if the opacity is removed after a critical period of development. A severe strabismus in early life, especially an esotropia, will have the same effect (amblyopia ex anopsia).
The vascular supply of the eye is through the ophthalmic branch of the internal carotid artery that supplies the retina, posterior (uveal) coats of the eye, and optic nerve head. This artery gives origin to the posterior ciliary arteries; the latter form a rich circumferential plexus of vessels (arterial circle of Zinn-Haller) located deep to the lamina cribrosa. The lamina cribrosa is a sieve-like scleral (dural) structure through which the axons of the central and nasal part of the disc run. This arterial circle supplies the optic disc and adjacent part of the distal optic nerve, the choroid, and the ciliary body; it anastomoses with the pial arterial plexus that surrounds the optic nerve. The other major branch of the ophthalmic artery is the central retinal artery. It supplies the inner retinal layers and issues from the optic disc, where it divides into four branches, each of which supplies a quadrant of the retina; it is these vessels and their branches that are visible by ophthalmoscopy. A short distance from the disc, these vessels lose their internal elastic lamina and the media (muscularis) becomes thin; they are properly classed as arterioles. The inner layers of the retina, including the ganglion and bipolar cells, receive their blood supply from these arterioles and their capillaries, whereas the deeper photoreceptor elements and the fovea are nourished by the underlying choroidal vascular bed, by diffusion through the retinal pigmented cells and the semipermeable Bruch membrane upon which they rest. In up to a third of the population, a small cilioretinal artery may arise from either the choroidal circulation or from the circle of Zinn-Haller and supply the macula. In the case of a central retinal artery occlusion, the presence of this cilioretinal artery leads to the preservation of central acuity.
As indicated above, the thin (100- to 350-mm) retinal sheet and the optic nerve head, into which all visual information flows, are exteriorized parts of the CNS and the only part of the nervous system that can be inspected directly. A common limitation in the funduscopic examination in cases of visual loss is failure to carefully inspect the macular zone (which is located 3 to 4 mm lateral to the optic disc and provides for 95 percent of visual acuity). There are variations in the appearance of the normal macula and optic disc, and these may prove difficult to distinguish from disease. A normal macula may be called abnormal because of a slight aberration of the retinal pigment epithelium, a few drusen, or a deep optic cup (see further on). With experience, the examiner can visualize the unmyelinated nerve-fiber layer of the retina by using bright-green (red-free) illumination. This is most often helpful in detecting demyelinative lesions of the optic nerve, which produce a loss of discrete bundles of the radially arranged and arching bundles of retinal fibers as they converge to the disc.
The absence of receptive elements in the optic disc accounts for the normal blind spot. The normal optic disc varies in color, being paler in infants and in blond individuals. The ganglion cell axons normally acquire their myelin sheaths after penetration of the lamina cribrosa, but they sometimes do so in their intraretinal course, as they approach the disc. These myelinated fibers adjacent to the disc appear as white patches with fine-feathered edges and are a normal variant, not to be confused with exudates.
In evaluating the retinal vessels, one must remember that these are arterioles and not arteries. Since the walls of retinal arterioles are transparent, what is observed with the ophthalmoscope is a column of blood within them. The central light streak of normal arterioles is thought to represent the reflection of light as it strikes the interface of the column of blood and the concave vascular wall. In arteriolosclerosis (usually coexistent with hypertension), the lumina of the vessels are segmentally narrowed because of fibrous tissue replacement of the media and thickening of the basement membrane. Straightening of the arterioles and venous compression by arterioles are other signs of hypertension and arteriolosclerosis. In this circumstance the vein is compressed by the thickened arteriole within the adventitial envelope shared by both vessels at the site of crossing. Progressive arteriolar disease, to the point of occlusion of the lumen, results in a narrow, white (“silver-wire”) vessel with no visible blood column. This change is associated most often with severe hypertension but may follow other types of occlusion of the central retinal artery or its branches (see descriptions and retinal illustrations further on). Sheathing of the venules, probably representing focal leakage of cells from the vessels, is reportedly observed in up to 25 percent of patients with the optic neuritis of multiple sclerosis, but we have only rarely been able to detect it. Arterial and venule sheathing are also seen in leukemia, sarcoid, Behçet disease, and other forms of vasculitis.
In malignant, or accelerated, hypertension there are, in addition to swelling of the optic nerve head and the retinal arteriolar changes noted above, a number of extravascular lesions: the so-called soft exudates or cotton-wool patches, sharply marginated and glistening “hard” exudates, and retinal hemorrhages. In many patients who show these retinal changes, analogous lesions are found in the brain (necrotizing arteriolitis and microinfarcts) and underlie hypertensive encephalopathy.
Microaneurysms of retinal vessels appear as small, discrete red dots and are located in largest number in the paracentral region. They are most often a sign of diabetes mellitus, sometimes appearing before the usual clinical manifestations of that disease. The use of the red-free (green) light on the ophthalmoscope helps to pick out microaneurysms from the background. Microscopically, the aneurysms take the form of small (20- to 90-mm) saccular outpouchings from the walls of capillaries, venules, or arterioles. The vessels of origin of the aneurysms are invariably abnormal, being either acellular branches of occluded vessels or themselves occluded by fat or fibrin.
The ophthalmoscopic appearance of retinal hemorrhage is determined by the structure of the particular tissue in which it occurs. In the superficial layer of the retina, they are linear or flame-shaped (“splinter” hemorrhages) because of their confinement by the horizontally coursing nerve fibers in that layer. These hemorrhages usually overlie and obscure the retinal vessels. Round or oval (“dot-and-blot”) hemorrhages lie behind the vessels, in the outer plexiform layer of the retina (synaptic layer between bipolar cells and nuclei of rods and cones—Fig. 13-2); in this layer, blood accumulates in the form of a cylinder between vertically oriented nerve fibers and appears round or oval when viewed end-on with the ophthalmoscope. Rupture of arterioles on the inner surface of the retina—as occurs with ruptured intracranial saccular aneurysms, arteriovenous malformations, and other conditions causing sudden severe elevation of intracranial pressure—permits the accumulation of a sharply outlined lake of blood between the internal limiting membrane of the retina and the vitreous or hyaloid membrane (the condensed gel at the periphery of the vitreous body); this is the subhyaloid or preretinal hemorrhage, termed Terson syndrome. Either the small superficial or deep retinal hemorrhage may show a central or eccentric pale (Roth) spot, which is caused by an accumulation of white blood cells, fibrin, histiocytes, or amorphous material between the vessel and the hemorrhage. This lesion is said to be characteristic of bacterial endocarditis, but it is also seen in leukemia and occasionally in embolic retinopathy caused by carotid disease.
Cotton-wool patches, or soft exudates, like splinter hemorrhages, overlie and tend to obscure the retinal blood vessels. These patches, even large ones, rarely cause serious disturbances of vision unless they involve the macula. Soft exudates are in reality infarcts of the nerve-fiber layer, caused by occlusion of precapillary arterioles; they are composed of clusters of ovoid structures called cytoid bodies, representing the terminal swellings of interrupted axons. Hard exudates appear as punctate white or yellow bodies; they lie in the outer plexiform layer, behind the retinal vessels, like the punctate hemorrhages. If present in the macular region, they are arranged in lines radiating toward the fovea (macular star). Hard exudates consist of lipid and other serum precipitants as a result of abnormal vascular permeability of a type that is not completely understood. They are observed most often in cases of diabetes mellitus and chronic hypertension.
Drusen in the retina (colloid bodies) appear ophthalmoscopically as pale yellow spots and are difficult to distinguish from hard exudates except when they occur alone; as a rule, hard exudates are accompanied by other funduscopic abnormalities. Although retinal drusen may be a benign finding, in many cases they reflect an ARMD and their accumulation in the macula eventually leads to significant visual loss. The source of retinal drusen is uncertain, but they may result from chronic inflammation generated by degeneration of the retinal pigment epithelium. Hyaline bodies located on or near the optic disc, are also referred to as drusen but must be distinguished from those occurring peripherally. In contrast to peripheral retinal drusen, drusen of the optic discs are probably mineralized residues of dead axons and can be seen on CT in some cases. Their main significance for neurologists is that drusen that are buried under the disc (“buried drusen”) are often associated with anomalous elevation of the disc that can be mistaken for papilledema (see further on) but they are for the most part, benign.
The periphery of the retina may harbor a hemangioblastoma, which may appear during adolescence, before the more characteristic cerebellar lesion. A large retinal artery may be seen leading to it and there may be a large draining vein. Occasionally, retinal examination discloses the presence of a vascular malformation that may be coextensive with a much larger malformation of the optic nerve and basilar portions of the brain.
Transient ischemic attacks of visual loss involving all or part of the field of vision of one eye are referred to as amaurosis fugax or transient monocular blindness (TMB). They are common manifestations of atherosclerotic carotid stenosis but have other causes. An altitudinal horizontal border, or “shade”, is often, but not invariably, an aspect of the visual loss. The shade may rise or fall at the onset or cessation of the spell and occasionally remains throughout the episode. Fortuitous inspection of the retina during an attack may show segments of arteries that are filled with white material that migrate distally over many minutes. There can be stagnation of arterial and venous blood flow, which returns within seconds or minutes as vision is restored (Fisher). One interpretation of these observations is that an embolus had occurred to the central retinal artery and had broken up and moved distally. Fisher went on to discredit the theory of the time that transient monocular blindness was due to vasospasm of the retinal arteries.
One or dozens of attacks may precede infarction of a cerebral hemisphere, or as often, they may abate without adverse consequence. In one series of 80 patients followed by Marshall and Meadows for 4 years, in an era prior to modern treatment of atherosclerosis, 16 percent developed permanent unilateral blindness, a completed hemispheral stroke, or both. Chapter 34 discusses this subject further.
Occlusion of the internal carotid artery usually causes no disturbance of vision whatsoever, provided that there are adequate anastomotic branches from the external carotid artery or other sources to the ophthalmic artery. Occasionally, occlusion of the proximal internal carotid artery is marked by an episode of transient monocular blindness on the same side, just as a hemispheral transient ischemic attack may indicate recent acute carotid occlusion. Chronic carotid occlusion with inadequate collateralization is associated with an ischemic oculopathy, which may predominantly affect the anterior or posterior segment or both. In this case, insufficient circulation to the anterior segment of the globe is manifest by scleral vascular congestion, cloudiness of the cornea, anterior chamber flare, and low intraocular pressure, or sometimes high intraocular pressure if neovascularization of the iris (rubeosis iridis) occurs and compromises the outflow of aqueous humor. Ischemia of the posterior segment of the eye is manifest by circulatory changes in the optic nerve or by venous stasis. Other signs of carotid disease may be present, for example, a local bruit over the carotid bifurcation.
Most often, ischemia of the retina can be traced to occlusion of the central retinal artery or its branches by thrombi or emboli—central retinal artery occlusion
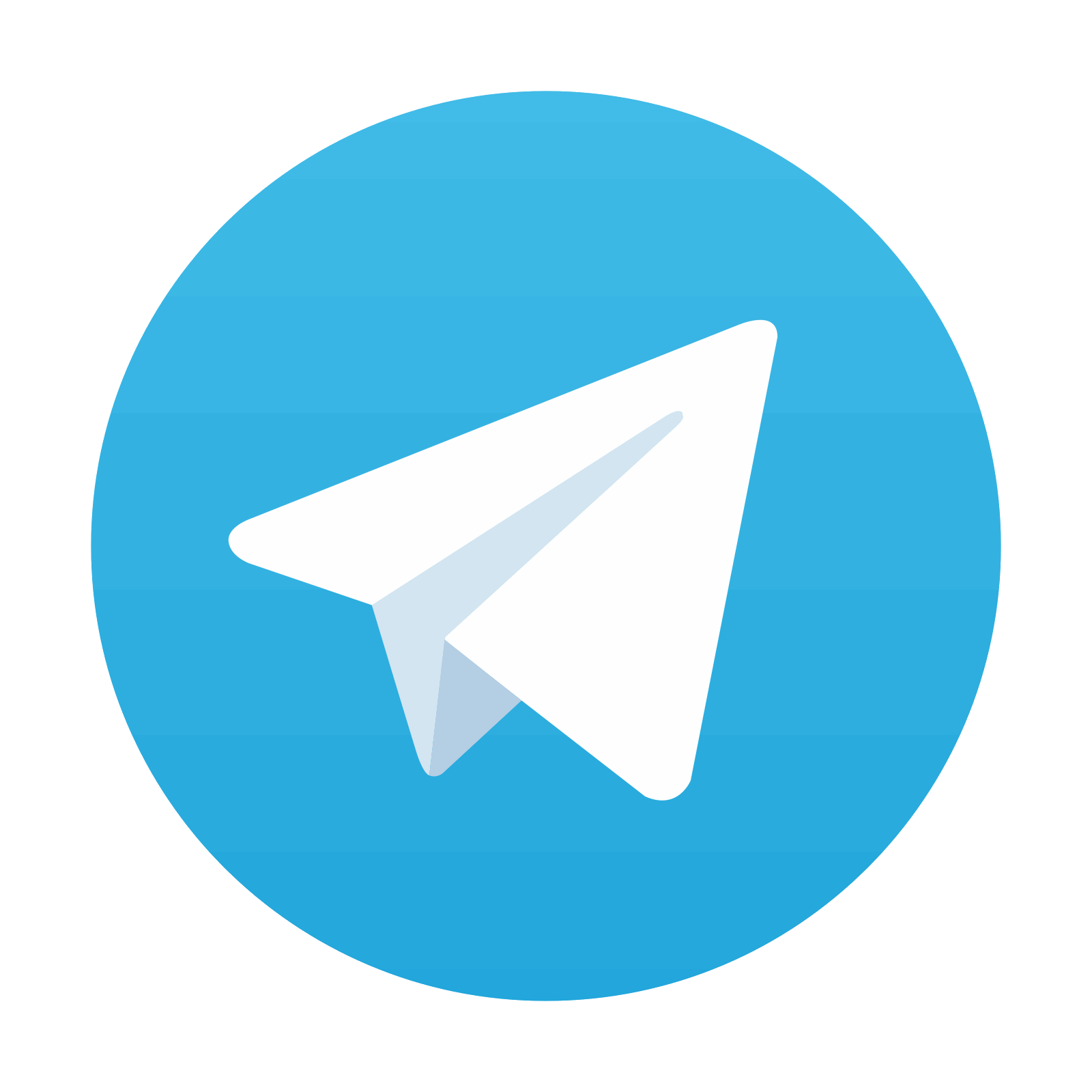
Stay updated, free articles. Join our Telegram channel

Full access? Get Clinical Tree
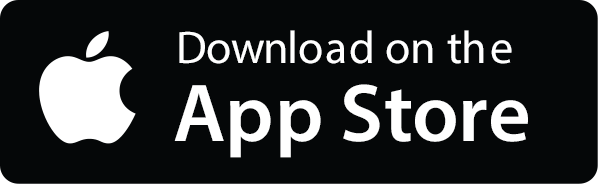
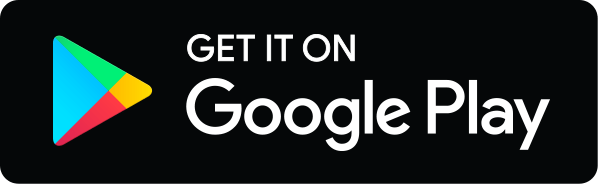
