Disorders of Ocular Movement and Pupillary Function: Introduction
Ocular movement and vision are virtually inseparable. A moving object evokes movement of the eyes and almost simultaneously arouses attention and initiates the perceptive process. To look searchingly, i.e., to peer, requires stable fixation of the visual image on the center of the two retinas. One might say that the ocular muscles are at the service of vision.
Abnormalities of ocular movement are of three basic types. One category can be traced to a lesion of the extraocular muscles themselves, the neuromuscular junction, or to the cranial nerves that supply them (nuclear or infranuclear palsy). The second type is a derangement in the highly specialized neural mechanisms that enable the eyes to move together (supranuclear and internuclear palsies). This distinction, in keeping with the general concept of upper and lower motor neuron paralysis, hardly portrays the complexity of the neural mechanisms governing ocular motility. Perhaps more common but not primarily neurologic is a third group of disorders, strabismus, in which there is a congenital imbalance of the yoked muscles of extraocular movement that may lead to a developmental reduction in monocular vision (amblyopia), as discussed at the end of the previous chapter. Knowledge of the anatomic basis of normal movement is essential to an understanding of abnormal movement.
Supranuclear Control of Eye Movement
In no aspect of human anatomy and physiology is the sensory guidance of muscle activity more instructively revealed than in the neural control of coordinated ocular movement. Moreover, the entirely predictable and “hardwired” nature of the central and peripheral oculomotor apparatus allows for a very precise localization of lesions within these pathways. To focus the eyes voluntarily, to stabilize objects for scrutiny when one is moving, to bring into sharp focus near and far objects—all require the perfect coordination of six sets of extraocular muscles and three sets of intrinsic muscles (ciliary muscles, sphincters, and dilators of the iris). The neural mechanisms that govern these functions reside mainly in the midbrain and pons but are greatly influenced by centers in the medulla, cerebellum, basal ganglia, and the frontal, parietal, and occipital lobes of the brain. Most of the nuclear structures and pathways concerned with fixation and stable ocular movements are now known and much has been learned of their physiology both from clinical-pathologic correlations in humans and from experiments in monkeys.
Accurate binocular vision is actually achieved by the associated action of all the ocular muscles. The symmetrical and synchronous movement of the eyes is termed conjugate movement or gaze (conjugate meaning yoked or joined together). The simultaneous movement of the eyes in different directions, as in convergence, is termed dysconjugate or disjunctive. These two forms of normal ocular movements are also referred to as versions (versional) and vergence, respectively. Vergence movements have two components—fusional and accommodative. The fusional movements are convergence and divergence, which maintain binocular single vision and depth perception (stereopsis); they are necessary at all times to ensure that visual images fall on corresponding parts of the retinas. Convergent movements are brought into action when one looks at a near object. The eyes turn inward and at the same time the pupils constrict and the ciliary muscles relax to thicken the lens and allow near vision (the accommodative-near reflex, or triad). Divergence, albeit slight, is required for distant vision.
Rapid voluntary conjugate movements of the eyes to the opposite side are initiated in area 8 of the frontal lobe (see Fig. 22-1) and relayed to the pons. These quick movements are termed saccadic (peak velocity may exceed 700 degrees per second). Their purpose is to rapidly change ocular fixation and bring images of new objects of interest onto the foveae. Saccades are so rapid that there is no subjective awareness of movement during the change in eye position, essentially, a momentary blindness. Saccadic movements can be elicited by instructing an individual to look to the right or left (commanded saccades), or to move the eyes to a target (refixation saccades). These two movements are sometimes differently affected in neurologic disease. Saccades may also be elicited reflexively, as when a sudden sound or the appearance of an object in the peripheral field of vision attracts attention and triggers an automatic movement of the eyes in the direction of the stimulus. Saccadic latency, the interval between the appearance of a target and the initiation of a saccade, is approximately 200 ms.
The neurophysiologic pattern of pontine neurons that produces a saccade has been characterized as “pulse-step” in type. This refers to the sudden increase in neuronal firing (the pulse) that is necessary to overcome the inertia and viscous drag of the eyes and move them into their new position; it is followed by a return to a new baseline firing level (the step), which maintains the eyes in their new position by tonic contraction of the extraocular muscles (gaze holding).
Saccades are distinguished from the slower and smoother pursuit or following movements, for which the major stimulus is a moving target. The function of pursuit movements is to stabilize the image of a moving object on the foveae, and thus to maintain a continuous clear image of the object as the object changes position (“smooth tracking”). Unlike saccades, pursuit movements to each side are generated in the ipsilateral parietooccipital cortex, with modulation by the ipsilateral cerebellum, especially the vestibulocerebellum (flocculus and nodulus). Another portion of the cerebellum, the posterior vermis, also influences saccadic movements (see further on).
When following a moving target, as the visual image slips off the foveae, the firing rate of the governing motor neurons increases in proportion to the speed of the target, so that eye velocity matches target velocity. If the eyes fall behind the target, supplementary catch-up saccades are required for refixation. The pursuit movement is then not smooth, but becomes jerky (“saccadic” pursuit). A lesion of one cerebral hemisphere may cause pursuit movements to that side to break up into saccades. Diseases of the basal ganglia are also a common cause of a disruption of normally smooth pursuit into a ratchet-like saccadic pursuit in all directions.
If a series of visual targets enters the visual field, as when one is watching trees from a moving car or the stripes on a rotating drum, involuntary repeated quick saccades refocus the eyes centrally; the resulting cycles of pursuit and refixation are termed optokinetic nystagmus. This phenomenon is used as a bedside test, the main value of which is in revealing a lesion of the ipsilateral posterior parietal lobe. It may also be found that a frontal lobe lesion eliminates the quick nystagmoid refixation phase away from the side of the lesion, thereby causing the eyes to continue to follow the target until it is out of view. This optokinetic phenomenon is described more fully further on, in the section on nystagmus.
Vestibular influences are of particular importance in stabilizing images on the retina during head and body movement. By means of the vestibuloocular reflex (VOR), a prompt short latency movement of the eyes is produced that is equal and opposite to movement of the head. During sustained rotation of the head, the VOR is supplemented by the optokinetic system, which enables one to maintain compensatory eye movements for a more prolonged period. If the VOR is lost, as occurs with disease of the vestibular apparatus or eighth nerve, the slightest movements of the head, especially those occurring during locomotion, cause a movement of images across the retina large enough to impair vision. A unilateral loss of the VOR strongly implicates a disease of the vestibular apparatus on the side toward the rotation of the head. When objects are tracked using both eye and head movements, the VOR must be suppressed, otherwise the eyes would remain fixed in space; to accomplish this, the smooth pursuit signals cancel the unwanted vestibular ones (Leigh and Zee). It follows that the inability to suppress the VOR, while viewing a target as the patient is rotated, is indicative of a defect of supranuclear pursuit.
As already mentioned, the signals for volitional horizontal gaze saccades originate in the eye field of the opposite frontal lobe (area 8 of Brodmann, see Fig. 22-1) and are modulated by the adjacent supplementary motor eye field and by the posterior visual cortical areas. Leichnetz traced the cortical-to-pontine pathways for saccadic horizontal gaze in the monkey. These fibers traverse the internal capsule and separate at the level of the rostral diencephalon into two bundles, the first being a primary ventral “capsular–peduncular” bundle, which descends through the most medial part of the cerebral peduncle. This more ventral pathway undergoes a partial decussation in the low midbrain, at the level of the trochlear nucleus, and terminates mainly in the vaguely defined paramedian pontine reticular formation (PPRF) of the opposite side, neurons of which, in turn, project to the adjacent sixth nerve nucleus (Fig. 14-1). A second, more dorsal “transthalamic” bundle is predominantly uncrossed and courses through the internal medullary lamina and paralaminar parts of the thalamus to terminate diffusely in the pretectum, superior colliculus, and periaqueductal gray matter. An off-shoot of these fibers (the prefrontal oculomotor bundle) projects to the rostral part of the oculomotor nucleus and to the ipsilateral rostral interstitial nucleus of the medial longitudinal fasciculus (riMLF) and to the interstitial nucleus of Cajal (INC), which are involved in vertical eye movements, as discussed in the next section.
Figure 14-1.
The supranuclear pathways subserving saccadic horizontal gaze to the left. The pathway originates in the right frontal cortex, descends in the internal capsule, decussates at the level of the rostral pons, and descends to synapse in the left pontine paramedian reticular formation (PPRF). Further connections with the ipsilateral sixth nerve nucleus and contralateral medial longitudinal fasciculus are also indicated. Cranial nerve nuclei III and IV are labeled on left; nucleus of VI and vestibular nuclei (VN) are labeled on right. The right MLF (green line) is labelled between the 3rd and 6th nerves on that side. LR, lateral rectus; MLF, medial longitudinal fasciculus; MR, medial rectus.
The pathways for smooth pursuit movements are less well defined. One probably originates in the posterior parietal cortex and the adjacent temporal, and anterior occipital cortex (area MT of the monkey) and descends to the ipsilateral dorsolateral pontine nuclei. Also contributing to smooth pursuit movements are projections from the frontal eye fields to the ipsilateral dorsolateral pontine nuclei. The latter, in turn, project to the flocculus and dorsal vermis of the cerebellum, which provide stability for the pursuit movements. However, for the purposes of clinical work, lesions of the posterior parietal cortex are the ones known to impair pursuit toward the damaged side. Part of the frontal eye fields have been shown experimentally to participate in pursuit eye movements, but the influence of this area on pursuit is far less than that of the parietal lobes and is insignificant clinically.
Conventionally, the term ocular motor nuclei refers to the nuclei of the third, fourth, and sixth cranial nerves; the term oculomotor nucleus refers to the third nerve nucleus alone. Ultimately, all the pathways mediating saccadic and pursuit movements in the horizontal plane, as well as for vestibular and optokinetic movements, converge on the pontine tegmental center for horizontal gaze, the PPRF. Also required for horizontal versional movements are the nuclei prepositus hypoglossi and their commissure, the abducens and medial vestibular nuclei, and pathways in the pontine and tegmentum of the brainstem that interconnect the oculomotor nuclei (Fig. 14-1).
The PPRF and the prepositus and medial vestibular nuclei are conceptualized as a “neural integrator” and relay station for horizontal saccade pathways. It is further considered that the PPRF projects to the sixth nerve nucleus to command horizontal eye movement. However, it is understood from animal experiments that supranuclear neural signals that encode smooth pursuit, and vestibular and optokinetic movements bypass the PPRF and project independently to the abducens nuclei (Hanson et al).
The fiber bundle connecting the third and sixth nerve nuclei, and connecting both these nuclei with the vestibular nuclei lies in the medial tegmentum of the brainstem; this pathway is the medial longitudinal fasciculus (MLF). The fibers of the MLF emanating from the sixth nerve nucleus cross in the pons and ascend to the contralateral medial rectus subnucleus of the third nerve. In this way, the abduction of one eye is yoked to adduction of the opposite one to produce conjugate horizontal gaze as described just below.
The abducens nucleus contains two groups of neurons, each with distinctive morphologic and physiologic properties: (1) the intranuclear abducens motor neurons, which innervate the ipsilateral lateral rectus muscle, and (2) abducens internuclear neurons, which project via the contralateral MLF to the medial rectus neurons of the opposite oculomotor nucleus. Conjugate lateral gaze is accomplished by the simultaneous activation of the ipsilateral lateral rectus, and the contralateral medial rectus, again, the latter through fibers that run in the medial portion of the MLF. Interruption of the MLF results in a discrete impairment or loss of adduction of the eye ipsilateral to the lesion, a sign referred to as internuclear ophthalmoplegia, the details of which are discussed further on (Fig. 14-1).
Two other ascending pathways between the pontine centers and the mesencephalic reticular formation have been traced: one traverses the central tegmental tract and terminates in the pretectum, in the nucleus of the posterior commissure; the other is a bundle separate from the MLF that passes around the nuclei of Cajal and Darkschewitsch to the riMLF. These nuclei are involved more in vertical gaze and are described below. In addition, each vestibular nucleus projects onto the abducens nucleus, and the MLF of the opposite side. This pathway is considered to generate the slow phase of the VOR.
Although direct projections from the frontal eye fields that bypass the PPRF and innervate the oculomotor nuclei, as described above, undoubtedly exist, indirect projections are more important in the voluntary control of conjugate eye movements. According to Leigh and Zee, a more accurate view of these various voluntary influences is one of a hierarchy of cell stations and parallel pathways that do not project directly to oculomotor nuclei but to adjacent premotor or burst neurons, which discharge at high frequencies immediately preceding a saccade. The premotor or burst neurons for horizontal saccades lie within the PPRF and those for vertical saccades in the riMLF (see below). Yet a third class of neurons (omnipause cells), lying in the midline of the pons, is involved in the inhibition of unwanted saccadic discharges. Nonetheless, in clinical work, the circuit that comprises in sequence (1) frontal lobe eye fields, (2) contralateral pontine PPRF, (3) abducens nucleus, (4) MLF, and (5) opposite oculomotor nucleus makes understandable a number of highly characteristic defects of horizontal ocular motion, as detailed in the remainder of the chapter.
In contrast to horizontal gaze, which is generated by unilateral aggregates of cerebral and pontine neurons, vertical eye movements, with few exceptions, are under bilateral control of the cerebral cortex and upper brainstem. The groups of nerve cells and fibers that govern upward and downward gaze, as well as torsional saccades, are situated in the pretectal areas of the midbrain and involve three integrated structures—the riMLF, the INC, and the nucleus and fibers of the posterior commissure (Fig. 14-2).
Figure 14-2.
Pathways for the control of vertical eye movements. The main structures are the interstitial nucleus of Cajal (INC), the rostral interstitial nucleus of the medial longitudinal fasciculus (riMLF), and the subnuclei of the third nerve, all located in the dorsal midbrain. Voluntary vertical movements are initiated by the simultaneous activity of both frontal cortical eye fields. The riMLF serves as the generator of vertical saccades and the INC acts tonically to hold eccentric vertical gaze. The INC and riMLF connect with their contralateral nuclei via the posterior commissure, where fibers are subject to damage. Projections for upgaze cross through the commissure before descending to innervate the third nerve nucleus, while those for downgaze may travel directly to the third nerve, thus accounting for the frequency of selective upgaze palsies (see text). The MLF carries signals from the vestibular nuclei, mainly ipsilaterally, to stabilize the eyes in the vertical plane (VOR) and maintain tonic vertical position.
The riMLF lies at the junction of the midbrain and thalamus, at the rostral end of the medial longitudinal fasciculus, just dorsomedial to the rostral pole of the red nucleus. It functions as the “premotor” nucleus with “burst cells” for the production of fast (saccadic) vertical versional and torsional movements. Input to the riMLF arises both from the PPRF and the vestibular nuclei. Each riMLF projects mainly ipsilaterally to the oculomotor and trochlear nuclei, but each riMLF is also connected to its counterpart by fibers that traverse the posterior commissure. Bilateral lesions of the riMLF or of their interconnections in the posterior commissure are more common than unilateral ones, and cause a loss either of downward saccades or of all vertical saccades.
The INC is a small collection of cells that lies just caudal to the riMLF on each side. Each nucleus projects to the motor neurons of the opposite elevator muscles (superior rectus and inferior oblique) by fibers that cross through the posterior commissure, and it projects ipsilaterally and directly to the depressor muscles (inferior rectus and superior oblique). The functional role of the INC appears to be in holding eccentric vertical gaze, especially after a saccade; it is also integral to the vestibuloocular reflex. Lesions of the INC produce a vertical gaze-evoked and torsional nystagmus, and an ocular tilt reaction and probably slow all conjugate eye movements, mainly vertical ones.
Lesions of the posterior commissure are common; they interrupt signals crossing to and from the INC and the riMLF. A lesion here characteristically produces a paralysis of upward gaze and of convergence, often associated with mild mydriasis, accommodative loss, convergence nystagmus, lid retraction (Collier “tucked lid” sign), and, less commonly, ptosis. This constellation is the Parinaud syndrome, also referred to as the pretectal, dorsal midbrain, or sylvian aqueduct syndrome (see “Vertical Gaze Palsy” further on). In some instances, only a restricted combination of these signs is seen. The same syndrome may be produced by unilateral lesions of the posterior commissure, presumably by interrupting bidirectional connections from the riMLF and INC. With acute lesions of the commissure, there is a tonic downward deviation of the eyes and lid retraction (“setting-sun sign”).
The MLF is the main conduit of signals that control vertical gaze from the vestibular nuclei in the medulla to the midbrain centers. For this reason, with internuclear ophthalmoplegia, along with the characteristic adductor paresis on the affected side, vertical pursuit and the VOR are impaired. This is most evident when there are bilateral internuclear ophthalmoplegias. Vertical deviation of the ipsilateral eye (skew) may be seen in cases of unilateral internuclear ophthalmoplegia, as discussed further on.
There are important vestibulocerebellar influences on both smooth pursuit and saccadic movements (see also Chap. 5). The flocculus and posterior vermis of the cerebellum receive abundant sensory projections from proprioceptors of the cervical musculature (responsive to head velocity), the retinas (sensitive to target velocity), proprioceptors of eye muscles (eye position and eye velocity), auditory and tactile receptors, and the superior colliculi and PPRF. Cerebellar efferents concerned with ocular movement project onto the vestibular nuclei, and the latter, in turn, influence gaze mechanisms through several projection systems: one, for horizontal movements, consists of direct projections from the vestibular nuclei to the contralateral sixth nerve nucleus; another, for vertical movements, projects via the contralateral MLF to third and fourth nerve nuclei (Figs. 14-1 and 14-2).
Lesions of the flocculus and posterior vermis are consistently associated with deficits in smooth pursuit movements and an inability to suppress the vestibuloocular reflex by fixation (Baloh et al). Floccular lesions are also an important cause of downbeat nystagmus. As indicated in Chap. 5, patients with cerebellar (floccular) lesions are unable to hold eccentric positions of gaze and must make repeated saccades to look at a target that is away from the neutral position (gaze-paretic nystagmus, a term also applied to nystagmus arising from a lesion in the PPRF). This phenomenon is explained by the fact that with acute, one-sided lesions of the vestibulocerebellum, the inhibitory discharges of the Purkinje cells onto the ipsilateral medial vestibular nucleus are removed, and the eyes deviate away from the lesion. When gaze to the side of the lesion is attempted, the eyes drift back to the midline, and fixation can be corrected only by a saccadic jerk. The head and neck may also turn away from the lesion (the occiput toward the lesion and the face away). In addition, the vestibuloocular reflexes, which coordinate eye movements with head movements, are improperly adjusted (Thach and Montgomery). The interested reader can find further details concerning cerebellar influences on ocular movements in the monograph by Leigh and Zee and the review by Lewis and Zee.
It is apparent from the foregoing remarks that there is considerable clinical information to be obtained from an analysis of ocular movements. To fully examine the eye movements, the patient should be asked to look quickly to each side as well as up and down (saccades) and to follow a moving target (pursuit of a light, the examiner’s finger, or an optokinetic drum). A patient with stupor and coma can be examined by passively turning the head or by irrigating the external auditory canals; these are vestibular stimuli to reflex eye movement as discussed in Chap. 17.
Most individuals make accurate saccades to a target. Alterations of saccadic movements, particularly overshooting of the eyes (hypermetria), are characteristic of a cerebellar lesion. Slowness of saccadic movements is mainly the result of disease of the basal ganglia such as Huntington and Wilson diseases, ataxia-telangiectasia, progressive supranuclear palsy, olivopontocerebellar degeneration, and certain lipid storage diseases. Lesions involving the PPRF may also be accompanied by slow saccadic movements to the affected side. Hypometric, slow saccades occurring only in the adducting eye indicate an incomplete internuclear ophthalmoparesis caused by a lesion of the ipsilateral MLF. When the earliest sign of a progressive eye movement disorder is slow saccades in the vertical plane, the likely diagnosis is progressive supranuclear palsy, but the same sign may occur in Parkinson disease and several less common processes that affect the basal ganglia, as discussed further on under “Vertical Gaze Palsy.” Slow up-and-down saccades are also found in Niemann-Pick disease type C.
Yet another saccadic disorder takes the form of an inability to initiate voluntary movements, either vertically or horizontally. This abnormality may be congenital in nature, as in the ocular “apraxia” of childhood (Cogan syndrome, see below) and in ataxia-telangiectasia; an acquired difficulty in the initiation of saccadic movements may be seen in patients with Huntington disease or with a lesion of the contralateral frontal lobe or ipsilateral pontine tegmentum. The most common explanation for the inability to move the eyes is hysteria, a circumstance that can be exposed by noting the patient will follow his own eyes in a mirror.
In addition to abnormalities of the saccades themselves, saccadic latency or reaction time (the interval between the impulse to move and movement) is prolonged in Huntington chorea and Parkinson disease. Saccadic latency is also increased in corticobasal ganglionic degeneration (see Chap. 39), in which case it seems to correspond to the degree of motor apraxia. The obligate need to initiate eye movements with a blink is often a subtle sign of disordered supranuclear control of conjugate movements that is evident in these same diseases and in other processes including frontal lobe lesions.
Fragmentation of smooth pursuit movement, a frequent neuroophthalmic finding, is a jerky irregularity of tracking that has been called “saccadic pursuit”. Two broad categories of disorders give rise to this phenomenon; a vestibulocerebellar and an extrapyramidal type. The former is commonly the result of sedative drug intoxication—with barbiturates, diazepam, and others as well as from a lesion of the vestibulocerebellar apparatus. In both, there is gaze directed nystagmus that seemingly interrupts pursuit movements as well. It is possible that sedatives cause this disturbance by affecting this same system.
A similar-appearing phenomenon, but one that does not manifest nystagmus, nicely called “cogwheel eye movements“, is seen in certain extrapyramidal diseases such as Parkinson disease, Huntington disease, and progressive supranuclear palsy. In these diseases there is a ratchet-like impairment of smooth pursuit movements in association with slow, hypometric saccades (“saccadic pursuit”). Indeed, according to Vidailhet and colleagues, smooth pursuit movements are impaired in all types of basal ganglionic degenerations.
Asymmetrical impairment of smooth pursuit movements is indicative of a parietal or a frontal lobe lesion. Pursuit is impaired toward the side of a parietal lesion and away from a frontal lesion, as described earlier. This is not a common clinical phenomenon unless elicited by optokinetic testing as explained further on.
This refers to involuntary conjugate eye movements that are contraversive to head turning and is manifest in unresponsive patients. Stimulation of the labyrinths by caloric or electrical activation elicits an identical phenomenon and can be performed in awake patients as well, as described in Chap. 17 on Coma.
The ability to suppress the VOR by visual fixation can provide considerable information and may be tested in various ways depending on the clinical need. One such test in the cooperative patient is performed by requesting that the patient fixate on a distant target and rapidly turning the head to one side by 5 to 10 degrees and (see “Tests of Labyrinthine Function” in Chap. 15). Slippage of fixation (impaired vestibuloocular reflex) is appreciated by observing a small corrective saccade in the direction opposite head turning. An alternative is to rotate the patient in a chair while he fixates on the thumb of his outstretched hand. There should be no loss of fixation at moderate rotational speeds; nystagmus during this maneuver is an abnormal sign. Zee has described yet another means of testing the VOR in which the examiner observes the optic nerve head while the patient rotates the head back and forth at a rate of one to two cycles per second. If the VOR is impaired, the optic nerve head appears to oscillate. Normally, movement of the head at this rate does not cause blurring of vision because of the rapidity with which the VOR accomplishes compensatory eye movements. As a result of inability to suppress the VOR, the patient experiences a feeling of instability.
Combined convergence and accommodative movements are tested by asking the patient to look at his thumbnail, the examiner’s finger, or object as it is brought toward the eyes. However, these fusional movements are frequently impaired in the elderly and in confused or inattentive patients and should not be interpreted as the result of disease in the ocular motor pathways. Otherwise, the absence or impairment of these movements should suggest a lesion in the rostral midbrain as a component of the Parinaud syndrome. Convergence spasms and retraction nystagmus may accompany paralysis of vertical gaze from a dorsal midbrain lesion. But when such convergent spasms occur alone, they are characteristic of hysteria, in which full horizontal movement can usually be obtained if each eye is tested separately. Also, cycloplegic eye drops will abolish accommodation and pupillary miosis.
Strictly speaking, gaze palsy refers to a complete loss of both saccadic and pursuit movements to one side. Gaze paresis would then refer to an incomplete loss of the same capacity. Operationally, a distinction is made between supranuclear disorders of gaze that can be overcome by intense stimuli, and certain nuclear lesions that cause an insurmountable loss of gaze to one side that cannot be overcome except by physically moving the globes (forced ductions).
As a rule, the horizontal gaze palsies of cerebral and pontine origin are readily distinguished by the side of an accompanying hemiparesis. When there is a tonic deviation of the eyes ipsilateral to a cerebral lesion, this relationship is expressed as “the eyes look toward the lesion and away from the hemiparesis.” The opposite pertains to brainstem gaze palsies, that is, gaze is impaired toward the side opposite the lesion, and if there is gaze deviation, the eyes are turned toward a hemiparesis. Palsies of pontine origin need not have an accompanying hemiparesis but are associated with other signs of pontine disease, particularly peripheral facial palsies and internuclear ophthalmoplegia on the same side as the paralysis of gaze. Pontine gaze palsies tend to be longer lasting than those of cerebral origin. Also, in the case of a cerebral lesion (but not a pontine lesion), the eyes turn to the paralyzed side if they are fixated on the target, and the head is rotated passively to the opposite side (i.e., by utilizing the VOR).
An acute lesion of one frontal lobe, such as an infarct, usually causes impersistence or paresis of contralateral gaze (more so than an actual palsy of gaze), and the eyes may for a limited time turn involuntarily toward the side of the cerebral lesion. In most cases of acute frontal lobe damage, the gaze palsy is incomplete and temporary, lasting for a week or less. Almost invariably, it is accompanied by hemiparesis. Forced closure of the eyelids may cause the eyes to move paradoxically to the side of the hemiparesis rather than upward (the latter being the Bell phenomenon), as would be expected. Similarly, during sleep, the eyes may also deviate conjugately away from the side of the lesion toward the side of the hemiplegia. As indicated above, pursuit movements away from the side of the lesion tend to be fragmented or lost. Posterior parietal lesions reduce pursuit movements but do not cause gaze palsy.
With bilateral frontal lesions, the patient may be unable to turn his eyes voluntarily in any direction but retains fixation and following movements. Occasionally, a deep cerebral lesion, particularly a thalamic hemorrhage extending into the midbrain, will cause the eyes to deviate conjugately to the side opposite the lesion (“wrong-way” gaze); the basis for this anomalous phenomenon is not established, but interference with descending oculomotor tracts in the midbrain has been postulated by Tijssen. It should be emphasized that cerebral gaze paralysis is not attended by strabismus or diplopia, i.e., the eyes always move conjugately. The usual causes of gaze paresis are vascular occlusion with infarction, hemorrhage, and abscess or tumor of the frontal lobe.
A seizure originating in the frontal lobe may also drive the eyes to the opposite side. When the eyes are driven contralaterally from the cerebral focus they may not return to the midline, giving the impression of gaze palsy. Also, in the postictal period, the eyes may reside in the opposite direction, ipsilaterally to the seizure focus.
The most common source of a nuclear-infranuclear gaze palsy is a lesion in the pontine horizontal gaze complex (PPRF; also involving the abducens nucleus), which causes ipsilateral gaze palsy and deviation of the eyes to the opposite side (Table 14-1). A unilateral lesion in the rostral midbrain tegmentum, by interrupting the cerebral pathways for horizontal conjugate gaze before their decussation, will also cause a paresis of gaze to the opposite side. Vestibulocerebellar lesions can cause yet another disorder of conjugate gaze that simulates a gaze palsy in which the eyes are forced, or driven to one side in a manner termed “pulsion” that prevents voluntary movement to the other side.
Midbrain infarction and hemorrhage |
Tumor in the region of the dorsal midbrain (e.g., pinealoma) |
Advanced hydrocephalus with enlargement of third ventricle |
Progressive supranuclear palsy |
Parkinson disease |
Lewy body disease |
Cortical basal ganglionic degeneration |
Whipple disease |
Metabolic diseases of childhood (Niemann-Pick type C, Gaucher, Tay-Sachs) |
Any cause of bilateral internuclear ophthalmoplegia (e.g., multiple sclerosis) |
Midbrain lesions affecting the pretectum and the region of the posterior commissure interfere with conjugate movements in the vertical plane. Paralysis of vertical gaze is a prominent feature of the Parinaud or dorsal midbrain syndrome described earlier. Upward gaze in general is affected far more frequently than downward gaze because, as already explained, some of the fibers subserving upgaze cross rostrally and posteriorly between the riMLF and INC and are subject to interruption before descending to the oculomotor nuclei, whereas the pathways for down-gaze apparently project directly downward to oculomotor nuclei from the two controlling centers.
The range of upward gaze is frequently restricted by extraneous factors, such as drowsiness, increased intracranial pressure, and particularly, aging. In a patient who cannot elevate the eyes voluntarily, the presence of reflex upward deviation of the eyes in response to flexion of the head (“doll’s-head maneuver”) or to voluntary forceful closure of the eyelids (Bell phenomenon) indicates that the nuclear and infranuclear mechanisms for upward gaze are intact and that the defect is supranuclear. However, useful this rule may be, in some instances of disease of the peripheral neuromuscular apparatus—such as Guillain-Barré syndrome and myasthenia gravis—in which voluntary upgaze may be limited, the strong stimulus of eye closure may cause upward deviation, whereas voluntary attempts at upgaze are unsuccessful, thereby spuriously suggesting a lesion of the upper brainstem. In addition, approximately 15 percent of normal adults do not show a Bell phenomenon; in others, deviation of the eyes is paradoxically downward.
In patients who during life had shown an isolated palsy of downward gaze, autopsy has disclosed bilateral lesions of the rostral midbrain tegmentum (just medial and dorsal to the red nuclei). An unusual case, described by Bogousslavsky and colleagues, suggests that a paralysis of vertical gaze may follow a strictly unilateral infarction that comprises the posterior commissure, riMLF, and INC. Hommel and Bogousslavsky have summarized the location of strokes that cause monocular and binocular vertical gaze palsies.
Several degenerative and related processes exhibit selective or prominent upgaze or vertical gaze palsies, as mentioned earlier (Table 14-1). In progressive supranuclear palsy, a highly characteristic feature is a selective paralysis of vertical gaze, with the more specific feature being downward paralysis beginning with impairment of saccades and later, restriction of all vertical movements. Parkinson and Lewy-body diseases (see Chap. 39), corticobasal ganglionic degeneration (see Chap. 39), and Whipple disease of the brain (see Chap. 40) may also produce vertical gaze palsies as these diseases progress.
The ocular tilt reaction, in which skew deviation (unilateral vertical separation of the eyes, discussed further on) is combined with ocular torsion and head tilt, is attributed to an imbalance of otolithic-ocular and otolithic-colic reflexes. In lesions involving the vestibular nuclei, as occurs in lateral medullary infarction, the eye is lower on the side of the lesion. With lesions of the MLF or INC, which can also cause skew and an ocular tilt reaction, the eye is higher on the side of the lesion.
Another unusual disturbance of gaze is the oculogyric crisis, or spasm, which consists of a tonic spasm of conjugate deviation of the eyes, usually upward and less frequently, laterally or downward. Recurrent attacks, sometimes associated with spasms of the neck, mouth, and tongue muscles and lasting from a few seconds to an hour or two, were pathognomonic of postencephalitic parkinsonism in the past. Now this phenomenon is observed as an acute reaction in patients being given phenothiazine and related neuroleptic drugs and in Niemann-Pick disease. The pathogenesis of these ocular spasms is not known. In the drug-induced form, upward deviation of the eyes is often associated with a report by the patient of peculiar obsessional thoughts; the entire syndrome can be terminated by the administration of an anticholinergic medication such as benztropine.
Congenital oculomotor “apraxia” (Cogan syndrome) is a congential disorder characterized by unusual eye and head movements that are obligately tied together during attempts to change the position of the eyes. The patient is unable to make normal voluntary horizontal saccades when the head is stationary. If the head is free to move and the patient is asked to look at an object to either side, the head is thrust to one side and the eyes turn in the opposite direction; the head overshoots the target, and the eyes, as they return to the central position, fixate on the target. Both voluntary saccades and the quick phase of vestibular nystagmus are defective. The pathologic anatomy is not understood but the condition abates over time. This same phenomenon is also seen in ataxia-telangiectasia (Louis-Bar disease, Chap. 37) and with agenesis of the corpus callosum, in which both horizontal and vertical saccades may be affected.
Nuclear and Infranuclear Disorders of Eye Movement
The third (oculomotor), fourth (trochlear), and sixth (abducens) cranial nerves innervate the extrinsic muscles of the eye. Because their actions are closely integrated and many diseases involve all of them at once, they are suitably considered together.
The oculomotor (third-nerve) nuclei consist of several paired groups of motor nerve cells adjacent to the midline, and ventral to the aqueduct of Sylvius at the level of the superior colliculi. A centrally located group of cells that innervate the pupillary sphincters and ciliary bodies (muscles of accommodation) is situated dorsally in the Edinger-Westphal nucleus that subserves pupillary reactions to light and the near vision response; this is the parasympathetic portion of the oculomotor nucleus. Ventral to this nuclear group are cells that mediate the actions of the levator of the eyelid, superior and inferior recti, inferior oblique, and medial rectus, in this dorsal–ventral order. This functional arrangement has been determined in cats and monkeys by extirpating individual extrinsic ocular muscles and observing the retrograde cellular changes (Warwick). Subsequent studies using radioactive tracer techniques have shown that medial rectus neurons occupy three disparate locations within the oculomotor nucleus rather than being confined to its ventral tip (Büttner-Ennever and Akert). These experiments also indicated that the medial and inferior recti, and the inferior oblique are innervated strictly ipsilaterally from the oculomotor nuclei, whereas the superior rectus receives only crossed fibers, and the levator palpebrae superioris (lid elevators) has bilateral innervations. Whether this precise arrangement is reproduced in humans is not known. Vergence movements are under the control of medial rectus neurons and not, as was once supposed, by an unpaired medial group of cells (nucleus of Perlia).
The fibers of the third-nerve nucleus course ventrally in the midbrain, crossing the medial longitudinal fasciculus, red nucleus, substantia nigra, and medial part of the cerebral peduncle successively. Lesions involving these structures therefore interrupt oculomotor fibers in their intramedullary (fascicular) course and give rise to several crossed syndromes of hemiplegia and ocular palsy. With regard to the oculomotor subnuclei, schematic arrangements of their projections have been derived from various sources, mainly experimental but some clinical, and are shown in the figure from Ksiazek and colleagues (Fig. 14-3). The emerging fibers can be considered as situated in medial, lateral and rostro-caudal groups, with the pupillary fibers occupying the rostro-medial aspect. This location of axons destined for the pupil continues through the third nerve. This information becomes useful in recognizing that combined pupillary and inferior and medial rectus palsies on one side may be the result of a fascicular lesion of the oculomotor nerve.
Figure 14-3.
Topographic arrangement of oculomotor fascicular fibers in the mesencephalon. P, pupil; SR, superior rectus; IR, inferior rectus; MR, medial rectus; IO, inferior oblique; LP, levator palpebrae; CCN, central caudal nucleus. (From Ksiazek SM, Slamovits TL, Rosen CE, et al: Fascicular arrangement in partial oculomotor paresis. Am J Ophthalmol 118: 97, 1994.)
The sixth nerve (abducens) arises at the level of the lower pons from a paired group of cells in the floor of the fourth ventricle, adjacent to the midline. The intrapontine portion of the facial nerve loops around the sixth-nerve nucleus before it turns anterolaterally to make its exit; a lesion in this locality therefore causes a homolateral paralysis of the lateral rectus and facial muscles. It is important to note that the efferent fibers of the oculomotor and abducens nuclei have a considerable intramedullary extent, i.e., their fascicular portions (Fig. 14-4A and B).
Figure 14-4.
A. Midbrain in horizontal section, indicating the effects of lesions at different points along the intramedullary course of the third-nerve fibers. A lesion at the level of oculomotor nucleus results in homolateral third-nerve paralysis and homolateral anesthesia of the cornea. A lesion at the level of red nucleus results in homolateral third-nerve paralysis and contralateral ataxic tremor (Benedikt and Claude syndromes). A lesion near the point of exit of third-nerve fibers results in homolateral third-nerve paralysis and crossed corticospinal tract signs (Weber syndrome; see Table 47-2). B. Brainstem at the level of the sixth-nerve nuclei, indicating effects of lesions at different loci. A lesion at the level of the nucleus results in homolateral sixth- and seventh-nerve paralyses with varying degrees of nystagmus and weakness of conjugate gaze to the homolateral side. A lesion at the level of corticospinal tract results in homolateral sixth-nerve paralysis and crossed hemiplegia (Millard-Gubler syndrome).
The cells of origin of the trochlear nerves are just caudal to those of the oculomotor nerves in the lower midbrain. Unlike the third and sixth nerves, the fourth nerve emerges from the dorsal surface of the lower midbrain and then courses posteriorly (dorsally) and decussates a short distance from its origin, just caudal to the inferior colliculi.
The nerves proceed circumferentially and ventrally around the midbrain toward the entry of the nerve into the posterior cavernous sinus. Each nucleus therefore innervates the contralateral superior oblique muscle. The long extraaxial course and the position of the nerves adjacent to the brainstem is a putative explanation for the common complication of fourth-nerve palsy in head injury (see Chap. 35). The superior oblique muscle forms a tendon that passes through a pulley structure (the trochlea) and attaches to the upper aspect of the globe. When the eye is adducted, the muscle exerts an upward pull, but being attached to the globe behind the axis of rotation, it causes depression and intorsion of the eye; in abduction, it thereby pulls the ocular meridian toward the nose, thereby causing intorsion (i.e., clockwise in the right eye and counterclockwise in the left from the examiner’s perspective).
The oculomotor nerve, soon after it emerges from the brainstem, passes between the superior cerebellar and posterior cerebral arteries. The nerve (and sometimes the posterior cerebral artery) may be compressed at this point by herniation of the uncal gyrus of the temporal lobe through the tentorial opening (see Chap. 17). The sixth nerve, after leaving the brainstem, sweeps upward along the clivus and then runs alongside the third and fourth cranial nerves; together they course anteriorly, pierce the dura just lateral to the posterior clinoid process, and run in the lateral wall of the cavernous sinus, where they are closely applied to the internal carotid artery and first and second divisions of the fifth nerve (Fig. 14-5 and “Cavernous Sinus Thrombosis” in Chap. 34).
Figure 14-5.
(See also Fig. 34-29.) The cavernous sinus and its relation to the cranial nerves. A. Base of the skull; the cavernous sinus has been removed on the right. B. The cavernous sinus and its contents viewed in the coronal plane.
When infraclinoid retrocavernous compressive lesions, such as aneurysms and tumors, affect the oculomotor nerve, they tend to also involve all three divisions of the trigeminal nerve. In the posterior portion of the cavernous sinus, the first and second trigeminal divisions are involved along with the oculomotor nerves; in the anterior portion, only the ophthalmic division of the trigeminal nerve is affected.
Just posterior and superior to the cavernous sinus, the oculomotor nerve crosses the terminal portion of the internal carotid artery at its junction with the posterior communicating artery. An aneurysm at this site frequently damages the third nerve; this serves to localize the site of compression or bleeding.
Together with the first division of the fifth nerve, the third, fourth, and sixth nerves enter the orbit through the superior orbital fissure. The oculomotor nerve, as it enters the orbit, divides into superior and inferior branches, although a functional separation of nerve bundles occurs well before this anatomic bifurcation. The superior branch supplies the superior rectus and the voluntary (striated) part of the levator palpebrae (the involuntary part is under the control of sympathetic fibers of Müller); the inferior branch supplies the pupillary and ciliary muscles and all the other extrinsic ocular muscles except, of course, two—the superior oblique and the lateral rectus which are innervated by the trochlear and abducens nerves, respectively. Superior branch lesions of the oculomotor nerve caused by an aneurysm or more commonly by diabetes, result in ptosis and uniocular upgaze paresis.
Under normal conditions, all the extraocular muscles participate in every movement of the eyes; for proper movement, the contraction of any muscle requires relaxation of its antagonist. Clinically, however, an eye movement can be thought of in terms of the one muscle that is predominantly responsible for an agonist movement in that direction, e.g., outward movement of the eye requires the action of the lateral rectus; inward movement, action of the medial rectus. The action of the superior and inferior recti and the oblique muscles varies according to the position of the eye. When the eye is turned outward, the elevator is the superior rectus and the depressor is the inferior rectus. When the eye is turned inward, the elevator and depressor are the inferior and superior oblique muscles, respectively. The actions of the ocular muscles in different positions of gaze are illustrated in Fig. 14-6 and Table 14-2.
MUSCLE | PRIMARY ACTION | SECONDARY ACTION | OCULOMOTOR NERVE |
---|---|---|---|
Medial rectus | Adduction | — | III |
Lateral rectus | Abduction | — | VI |
Superior rectus | Elevation | Intorsion | III |
Inferior rectus | Depression | Extorsion | III |
Superior oblique | Intorsion | Depression | IV |
Inferior oblique | Extorsion | Elevation | III |
The term binocular diplopia refers to the symptom of double vision caused by a misalignment of the visual axes of the two eyes. With very few exceptions, in order to experience diplopia there must be some vision in both eyes. Put another way, covering one eye usually obliterates double vision. If the visual axes are separated by a significant amount, the individual may suppress the image from one eye and not experience diplopia. There is a form of monocular diplopia that is due to lenticular or retinal disease and is also a manifestation of hysteria.
Strabismus, strictly speaking, refers to a muscle imbalance that results in misalignment of the visual axes, but the term is used most often by neurologists to describe a congenital variety of misalignment. Strabismus may be caused by weakness of an individual eye muscle (paralytic strabismus) or by an imbalance of muscular tone, presumably because of a faulty “central” mechanism that normally maintains a proper angle between the two visual axes (nonparalytic or pediatric strabismus, see below). Almost everyone has a slight tendency to strabismus, i.e., to misalign the visual axes when a target is viewed preferentially with one eye. This tendency is referred to as a phoria and is normally overcome by the fusion mechanisms. A misalignment that is manifest during binocular viewing of a target and cannot be overcome, even when the patient is forced to fixate with the deviant eye, is called a tropia. The ocular misalignment is then apparent by viewing the position of the patient’s eyes while they fixate on a distant target. When tested monocularly, the range of movement in the affected eye are normal, or nearly so. The prefixes eso- and exo– indicate that the phoria or tropia is directed inward or outward, respectively, and the prefixes hyper– and hypo-, that the deviation is upward or downward. Paralytic strabismus is primarily a neurologic problem; nonparalytic strabismus (referred to as comitant strabismus if the angle between the visual axes is the same in all fields of gaze) is an ocular muscle problem that is managed by ophthalmologists, although it is associated with a number of congenital cerebral diseases and forms of developmental delay.
It is in this sense that the unqualified term strabismus is often used. The normal slight exotropia of neonates corrects by about 3 months of age. Large malalignments (greater than 15 degrees) are considered abnormal, even at birth. Most children with developmental esotropic strabismus present between ages 2 and 3 years, whereas those with exotropia show the condition in a broader range of preschool years. Esodeviations are initially intermittent and then become persistent; exodeviations are commonly intermittent. In both cases, eye movements are full and the child initially alternates fixation.
Esotropia is typically an acquired problem as a result of congenital farsightedness and the overengagement of the near response in order to see clearly, thereby driving the eyes to cross. Treatment with glasses within 6 months of the onset of the strabismus restores vision and usually leads to realignment of the axes. Large degrees of esotropia that are not the result of hypermetropia (farsightedness) are best treated by surgical realignment.
In contrast, persistent exotropic strabismus in a child is usually associated with a developmental delay, often as a component of a recognizable mental retardation syndrome, as detailed in Chap. 38, or with ocular pathology. It does, however, occur in neurologically normal children. If mild, intermittent exotropia is initially treated by one of a number of nonsurgical means such as patching and visual exercises to stimulate convergence; surgical correction is reserved for unresponsive cases. Donohue has written an informative review of the subject.
Once binocular fusion is established, usually by 6 months of age, any type of ocular muscle imbalance will cause diplopia, as images then fall on disparate or noncorresponding parts of the two functionally active retinas. After a time, however, the child eliminates the diplopia by suppressing the image from one eye. After another variable period, the suppression becomes permanent, and the individual retains diminished visual acuity in that eye, the result of prolonged disuse (amblyopia ex anopsia), as described in the last portion of Chap. 13.
Nonparalytic strabismus may create misleading ocular findings in the neurologic examination. Sometimes a slight phoric misalignment of the eyes is first noticed after a head injury or a febrile infection, or it may be exposed by any other neurologic disorder or drug intoxication that impairs fusional mechanisms (vergence). In a cooperative patient, nonparalytic strabismus may be demonstrated by showing that each eye moves fully when the other eye is covered. Tropias and phorias can also readily be detected by means of the simple “cover” and “cover–uncover” tests. When fusion is disrupted by covering one eye, the occluded eye will deviate; uncovering that eye results in a quick corrective movement designed to reestablish the fusion mechanism.
A complete third nerve lesion causes ptosis, or drooping of the upper eyelid (as the levator palpebrae is supplied mainly by this nerve), and an inability to rotate the eye upward, downward, or inward. This corresponds to the weaknesses of the medial, superior, and inferior recti and the inferior oblique muscles. The remaining actions of the fourth and sixth nerves give rise to a position of the eye described by the mnemonic “down and out.” The patient experiences diplopia in which the image from the affected eye is projected upward and medially. In addition, one finds a dilated, light-nonreactive pupil (iridoplegia), and paralysis of accommodation (cycloplegia) because of interruption of the parasympathetic fibers in the third nerve. However, the extrinsic and intrinsic (papillary) eye muscles may be affected separately in certain diseases. For example, infarction of the central portion of the oculomotor nerve, as occurs in diabetic ophthalmoplegia, typically spares the pupil, as the parasympathetic preganglionic pupilloconstrictor fibers lie near the surface. Conversely, compressive lesions of the nerve usually dilate the pupil as an early manifestation. After injury, regeneration of the third-nerve fibers may be aberrant, in which case some of the fibers that originally moved the eye in a particular direction now reach another muscle or the iris; in the latter instance the pupil, which is unreactive to light, may constrict when the eye is turned up and in.
A lesion of the fourth nerve, which innervates the superior oblique muscle, is the most common cause of isolated symptomatic vertical diplopia. Although oculomotor palsy was a more common cause of vertical diplopia in Keane‘s 1975 series, as stated earlier, in instances where this is the sole complaint, trochlear palsy (and brainstem lesions) have predominated in our material. Paralysis of the superior oblique muscle results in weakness of downward movement of the affected eye, most marked when the eye is turned inward (Fig. 14-7E
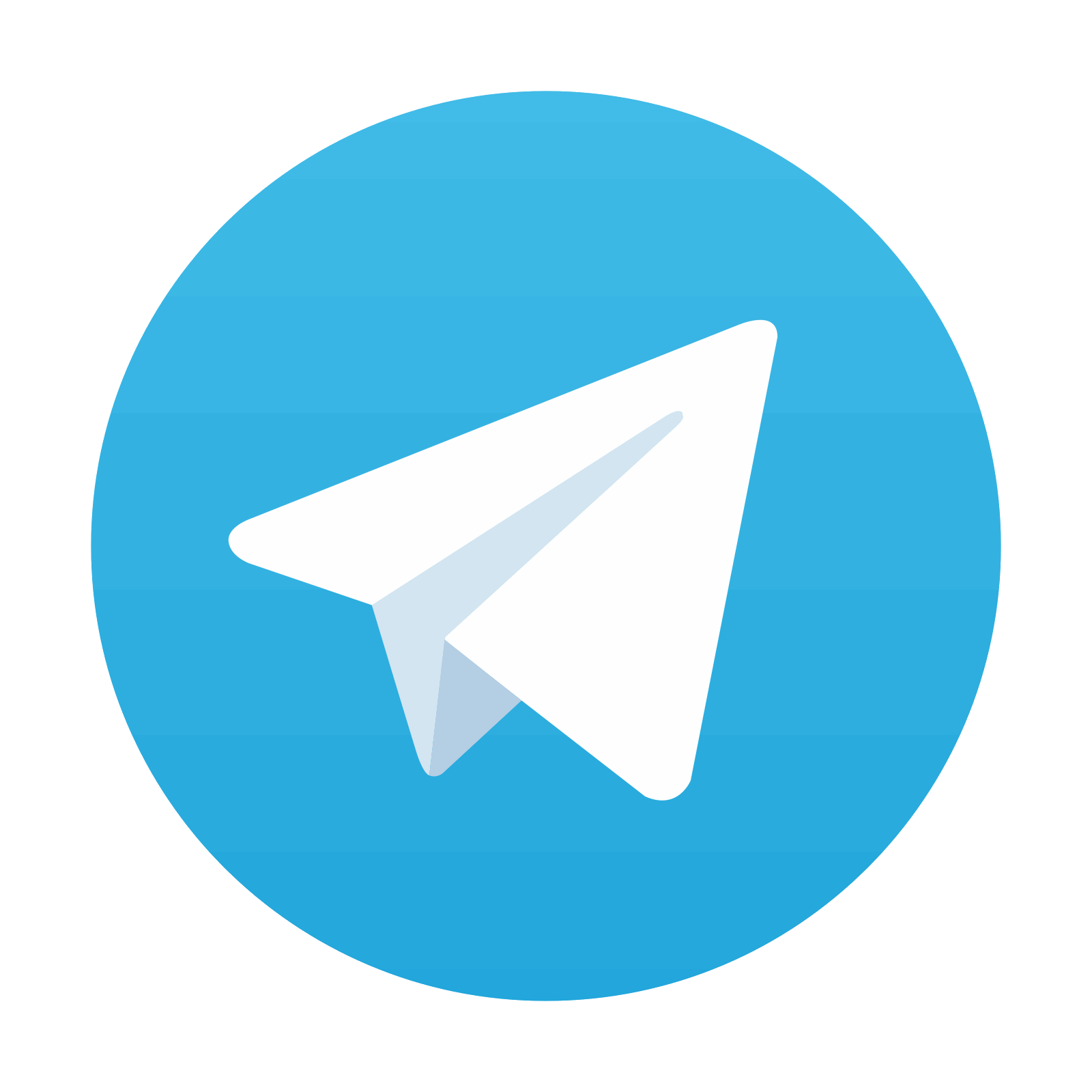
Stay updated, free articles. Join our Telegram channel

Full access? Get Clinical Tree
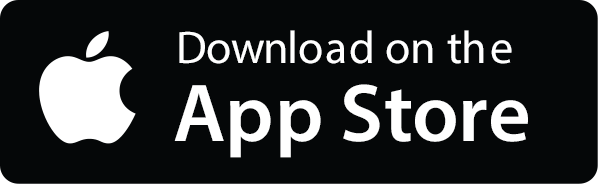
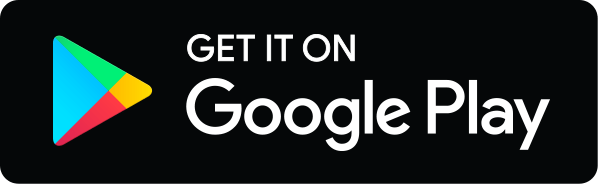
