Sleep and Its Abnormalities: Introduction
Sleep, that familiar yet inexplicable condition of repose in which consciousness is in abeyance, is obviously not abnormal, yet it is appropriately considered in connection with abnormal phenomena because there are a number of interesting and common irregularities of sleep, some of which approach serious extremes. Furthermore, a number of neurological conditions have special types of sleep disruption as common features. The psychologic and physiologic benefits of sleep are of paramount importance, and it is increasingly recognized that disruption of sleep increases the risks for a number of medical diseases, including stroke, hypertension, and coronary disease. Everyone, of course, has had a great deal of personal experience with sleep, or lack of it, and has observed people in sleep, so it requires no special knowledge to understand something about this condition or to appreciate its importance to health and well-being.
Physicians are frequently consulted by patients who suffer from some derangement of sleep. Most often, the problem is one of sleeplessness, but sometimes it concerns excessive sleepiness or some peculiar phenomenon occurring in connection with sleep. Certain points concerning normal sleep and the sleep–wake mechanisms are worth reviewing, as familiarity with them is necessary for an understanding of disorders of sleep. A great deal of information about sleep and sleep abnormalities is now available as a result of the development of the subspecialty of sleep medicine, and the creation of centers for the diagnosis and treatment of sleep disorders.
Physiology of Sleep and Sleep–Wake Mechanisms
Sleep represents one of the basic 24-h (circadian) rhythms, traceable through all mammalian, avian, and reptilian species. The neural control of circadian rhythms is thought to reside in the ventral-anterior region of the hypothalamus, more specifically, in the suprachiasmatic nuclei. Lesions in these nuclei result in a disorganization of the sleep–wake cycles as well as of the rest–activity, temperature, and feeding rhythms. Chapter 27 describes the ancillary role of melatonin and the pineal body in modulating this cyclic activity. There is also an important dimension of a homeostatic drive to sleep as the day wears on. This modulates the circadian rhythm independent of light entrainment of the circadian rhythm and makes the workday possible.
Observations of the human sleep–wake cycle show it to be closely age linked. The newborn baby sleeps from 16 to 20 h a day, and the child, 10 to 12 h. Total sleep time drops to 9 to 10 h by mid-adolescence and to about 7 to 7.5 h during young adulthood. A gradual decline to about 6.5 h develops in late adult life. However, there are wide individual differences in the length and depth of sleep, apparently as a result of genetic factors, early life conditioning, the amount of physical activity, and psychologic states.
The pattern of sleeping, which is adjusted to the 24-h day, also varies in the different epochs of life. The circadian rhythm, with predominance of daytime wakefulness and nighttime sleep, begins to appear only after the first few weeks of postnatal life of the full-term infant; as the child matures, the morning nap is omitted, then the afternoon nap; by the fourth or fifth year, sleep becomes consolidated into a single long nocturnal period. (Actually, a large part of the world’s population continues to have an afternoon nap, or siesta, as a lifelong sleep–wake pattern.) Fragmentation of the sleep pattern begins in late adult life. Over ensuing years, night awakenings tend to increase in frequency, and the daytime waking period may be interrupted by episodic sleep lasting seconds to minutes (microsleep), as well as by longer naps. From about 35 years of age onward, women tend to sleep slightly more than men.
Seminal contributions to our understanding of the physiology of sleep were made by Loomis and associates and by Aserinsky, Dement, and Kleitman through electroencephalographic analysis and clinical observation. As a result of their studies, five stages of sleep, representative of two alternating physiologic mechanisms, have been defined. In each stage, the electrical activity of the brain occurs in organized and recurring cycles, referred to as the architecture of sleep. As the electrophysiologic stages of sleep progress, sleep becomes deeper, meaning that arousal requires a more intense stimulus. These findings put to rest the antiquated ideas that sleep is a purely passive state and reflects fatigue and reduction in environmental stimuli.
Relaxed wakefulness with the eyes closed is accompanied in the electroencephalogram (EEG) by posterior alpha waves of 9 to 11 Hz (cycles per second) and intermixed low-voltage fast activity of mixed frequency. Except for the facial muscles, the electromyogram (EMG) is silent when the patient is sitting or lying quietly. With drowsiness, as the first stage of sleep sets in, the eyelids begin to droop, the eyes may rove slowly from side to side, and the pupils become smaller. As the early stage of sleep evolves, the muscles relax and the EEG pattern changes to one of progressively lower voltage and mixed frequency with a loss of alpha waves; this is associated with slow, rolling eye movements and is called stage 1 sleep. As this changes into stage 2 sleep, 0.5- to 2-s bursts of biparietal 12- to 14-Hz waves (sleep spindles) and intermittent high-amplitude, central-parietal sharp slow-wave complexes appear (vertex waves) (Fig. 19-1). The American Academy of Sleep Medicine (AASM) recommends the following staging: stage W (wakefulness), stage N1 (non-REM sleep, or NREM 1, formerly stage 1), stage N2 (NREM 2, formerly stage 2), stage N3 (NREM 3, combining former stages 3 and 4—or slow-wave sleep), and stage R (rapid eye movement [REM] sleep). The essential difference between this new nomenclature and the one formerly used by neurologists is that stage N3 now represents slow-wave sleep, replacing stage 3 and stage 4 sleep, composed of an increasing proportion of high-amplitude delta waves (0.75-μV, 0.5- to 2-Hz) in the EEG (Table 19-1). If the eyelids are raised gently, the globes are usually seen to be exotropic and the pupils are even smaller than before, but with retained responses to light. An additional stage of the sleep cycle, which follows the others intermittently throughout the night, is associated with further reduction in muscle tone except in the extraocular muscles and with bursts of rapid eye movement; thus the term REM sleep designates this stage. The EEG becomes desynchronized, i.e., it has a low-voltage, high-frequency discharge pattern. The first three stages of sleep are called nonrapid eye movement (NREM) sleep or synchronized sleep; the last stage, in addition to REM sleep, is variously designated as fast-wave, nonsynchronized, or desynchronized sleep. Figure 19-2 illustrates these features.
AMERICAN ACADEMY OF SLEEP MEDICINE | FORMER NOMENCLATURE | EEG CHARACTERISTICS | BEHAVIORAL STATE |
---|---|---|---|
W | — | Posterior reactive alpha rhythm | Awake |
N1 | Stage 1 | Diffuse theta and loss of alpha | Drowsy |
N2 | Stage 2 | Sleep spindles and K complexes | Light sleep |
N3 | Stages 3 and 4 | High-voltage theta and delta activity | Deep sleep |
R | REM | Diffuse theta | Predominance of dreaming (see text) |
Figure 19-2.
Representative polysomnographic recordings from adults in the awake state and various stages of sleep. Recordings are made at conventional sleep laboratory speed of 10 mm/s (i.e., at the paper speed of one-third standard clinical EEG recordings). A. Upper tracings: Awake state (with eyes closed). Alpha rhythms are prominent in EEG. Normally active chin EMG. B. Middle tracings: Stage 1 (N1) sleep. Onset of sleep is defined by the diminished amplitude of alpha waves in the occipital EEG channel (“flat” appearance). C. Lower tracings: Stage 2 (N2) sleep, characterized by appearance of high-amplitude single-complex (K) waves and bursts of 13- to 16-Hz waves (sleep spindles) on a background of low frequency. D. Upper tracings: Stage 3 (N3) sleep. Appearance of high-voltage slow (delta) waves. E. Middle tracings: Deepest stage of N3 sleep, with predominant delta-wave activity occupying 50 percent of a 30-s tracing. F. Lower tracings: Rapid eye movement (REM) sleep, characterized by episodes of REM and occasional muscle twitches in an otherwise flat chin EMG. Technical Note: Four sites from the same montage are illustrated in each recording: C3/A2, left central to right mastoid; O2/A1, right occipital to left mastoid; ROC/A1, right outer canthus to left mastoid; LOC/A2, left outer canthus to right mastoid. A chin EMG tracing is added to each recording. (Adapted with permission from Butkov N. Atlas of Clinical Polysomnography. Vol 1. Synapse Media, Medford, OR, 1996.)
In the first portion of a typical night’s sleep, the normal young and middle-aged adult passes successively through stages N1, N2, N3, and R (REM) sleep. After about 70 to 100 min, a large proportion of which consists of stage N3 sleep, the first REM period occurs, usually heralded briefly by a transient increase in body movements and a shift in the EEG pattern from that of stage N3 to stage N2. This NREM–REM cycle is repeated at about the same interval four to six times during the night, depending on the total duration of sleep. The first REM period may be brief; the later cycles have less stage N3 sleep or none at all. In the latter portion of a night’s sleep, the cycles consist essentially of two alternating stages—REM sleep and stage N2 (spindle–K-complex) sleep. The relation of dreaming to these sleep stages is described further on.
Newborn full-term infants spend approximately 50 percent of their sleep in the REM stage (although their EEG and eye movements differ from those of adults). The newborn sleep cycle lasts about 60 min (50 percent REM, 50 percent NREM, generally alternating through a 3- to 4-h interfeeding period); with age, the sleep cycle lengthens to 90 to 100 min. Approximately 20 to 25 percent of total sleep time in young adults is spent in REM sleep, 3 to 5 percent in stage N1, 50 to 60 percent in stage N2, and 10 to 20 percent in stage N3 combined. The amount of sleep in N3 decreases with age, and persons older than 70 years of age have virtually no very deep slow-wave sleep (Fig. 19-3). The 90- to 100-min cycle is fairly stable in any one person and is believed to continue to operate to a less-perceptible degree during wakefulness in relation to a number of other cyclic phenomena, such as core body temperature, gastric motility, hunger, urinary output, alertness, and capacity for cognitive activity.
Figure 19-3.
Sleep architecture, or sleep cycles. REM sleep (darkened areas) occurs cyclically throughout the night at intervals of approximately 90 min in all age groups. REM sleep shows little variation in the different age groups, whereas stage 4 sleep decreases with age. Stages 3 and 4 are now considered N3. (Redrawn by permission from Kales, Kales, and Soldatos.)
A comparison of the physiologic changes in NREM and REM sleep is of interest. The change in the EEG pattern has already been indicated. Cortical neurons tend to discharge in synchronized bursts during NREM sleep and in nonsynchronized bursts during wakefulness. In REM sleep, the EEG pattern is generally asynchronous as well. Much of the night’s complex visual dreaming has been found to occur in the REM period, with the qualifications noted below, and dreams are recalled most consistently if the subject is awakened during this time. It is important to point out, however, that dreaming activity is reported by subjects awakened from NREM sleep, although less consistently. Because the time spent in NREM is so much greater than that in REM, approximately 20 percent of dreaming occurs outside of REM periods but REM sleep nonetheless maintains a special relationship to dreaming.
Subjects are easily aroused from REM sleep, but arousing a person during stage N3 is more difficult; full arousal may take minutes or more, during which time the subject may be slightly disoriented and confused (for which reason, physicians called at night should, if possible, avoid making complex medical decisions during this brief period).
As mentioned above, tonic muscle activity is minimal during REM sleep, although small twitches in facial and digital muscles (hand and foot) can still be detected. Eye movements of REM sleep are conjugate and occur in all directions (horizontal more than vertical). They can be appreciated through the closed eyelids. Gross body movements occur every 15 min or so in all stages of sleep but are maximal in the transition between REM and NREM sleep, at which time the sleeping person changes position, usually from side to side (most people sleep on their sides).
On closer study, REM sleep has been found to have phasic and tonic components. In addition to the rapid eye movements, phasic phenomena include activation of the sympathetic nervous system with attendant alternate dilatation and constriction of the pupils and fluctuation of the blood pressure, heart rate, and respiration. The phasic activities are related to bursts of neuronal activity in the pontine, vestibular, and median raphe nuclei and are conducted through the corticobulbar and corticospinal tracts. In the nonphasic periods of REM sleep, alpha and gamma spinal neurons are inhibited, the H responses diminish (see Chap. 45), and the tendon and postural and flexor reflexes diminish or are abolished. This flaccidity and atonia, which are prominent in the abdominal, upper airway, and intercostal muscles, may compromise breathing during REM sleep and pose a threat to infants with respiratory difficulty and to adults who are obese or have respiratory difficulty as a result of kyphoscoliosis, muscular dystrophy, hypoplastic or otherwise compromised airways, and neuromuscular paralyses (see Guilleminault and Dement).
It has long been known that body temperature falls slightly during sleep; however, if sleep does not occur, there is still a drop in body temperature as part of the circadian (24-h) temperature pattern. This reduction in temperature is also independent of the 24-h recumbency–ambulatory cycle. During sleep, the decline in temperature occurs mainly during the NREM period, and the same is true of the heartbeat and respiration, both of which become slow and more regular in this period. Cerebral blood flow and oxygen consumption in muscle diminish during NREM sleep and increase during REM sleep. Also, cerebral blood flow and metabolism are markedly reduced across the entire brain during deep NREM sleep; however, during REM sleep, metabolism and blood flow are restored to the levels of the waking state (Madsen and Vorstrup). Presumably, as a result of increased blood flow, intracranial pressure rises during REM sleep.
Urine excretion decreases during sleep and the absolute quantity of sodium and potassium that is eliminated also decreases; however, urine specific gravity and osmolality increase, presumably because of increased antidiuretic hormone excretion, and reabsorption of water. Parasympathetic outflow is activated periodically in REM sleep; sympathetic activity is suppressed. It has also been recognized that there is a drop in blood pressure and heart rate during slow-wave sleep and the loss of this dip, for example as a result of sleep apnea, has been associated with daytime hypertension and increased risk for cardiovascular events. As mentioned, in phasic REM sleep, there is an increase in sympathetic tone. Breathing is more irregular, and heart rate and blood pressure fluctuate. Penile erections appear periodically, usually during REM periods.
A number of endocrine changes also have a regular relationship to the sleep–wake cycle. During the first 2 h of sleep, there is a surge of growth hormone secretion, mainly during slow-wave sleep. In men, there tends to be a single peak, whereas women have a multiple episodes of increased secretion. This feature persists through middle and late adult life and then disappears. The secretion of cortisol and particularly of thyroid-stimulating hormone peaks at the onset of sleep. High concentrations of cortisol are also characteristically found on awakening. Melatonin, elaborated by the pineal gland, is produced at night and ceases upon retinal stimulation by sunlight (see Chap. 27). Prolactin secretion increases during the night in both men and women, the highest plasma concentrations being found soon after the onset of sleep. Secretion of prolactin is influenced by sleep stages. Circadian mechanisms and the stages of sleep alter testosterone secretion and are therefore disrupted by sleep disorders, especially in younger individuals. Also, an increased sleep-associated secretion of luteinizing hormone occurs in pubertal boys and girls.
Hobson originally proposed that the basic oscillation of the sleep cycle is the result of reciprocal interaction of excitatory and inhibitory neurotransmitters. Single-cell recordings from the pontine reticular formation suggest that there are two interconnected neuronal populations whose levels of activity fluctuate periodically and reciprocally. During wakefulness, according to this conceptualization, the activity of aminergic (inhibitory) neurons is high; because of this inhibition, the activity of the cholinergic neurons is low. During NREM sleep, aminergic inhibition gradually declines and cholinergic excitation increases; REM sleep occurs when the shift is complete. It is likely that these monoaminergic neuronal circuits are modulated by input from hypocretin (also called orexin)-secreting neurons of the hypothalamus, but the details of this control system are not entirely known. Hypocretin, a peptide that assumes great importance in the pathophysiology of narcolepsy, is discussed further on.
A refinement of these views has elaborated the complex interaction of specially functioning nuclei in the hypothalamus, pons, and basal forebrain. Reciprocal connections among these areas, modulated by input from regions of the brain that sense environmental conditions, allow the organism to adapt sleep cycles to its needs and to external circumstances. The suprachiasmatic nucleus (SCN) of the hypothalamus has no direct influence on the sleep cycle but does integrate ambient light cues, and thereby affects various circadian rhythms, including sleep, as discussed in Chap. 27. Experiments in animals and analyses of cases of von Economo encephalitis (that caused a pathologic sleep syndrome) have indicated that the ventrolateral preoptic nucleus of the hypothalamus (VLPO) sends fibers to all the other major cell groups of the hypothalamus and brainstem that are engaged in arousal. Damage to the VLPO results in pathologic wakefulness, and the virtual absence of sleep. The SCN has only minimal projections to the VLPO and to orexin-containing neurons (see further on), but it does strongly innervate the subparaventricular zone (SPZ) and the dorsomedial hypothalamic nuclei. The last of these areas integrates feeding, temperature, light, and other cues from SPZ and SCN. The brain contains a three-stage pathway for the control of the sleep rhythm.
The above-described interconnections and the action of the integrated system that causes the sleep state have been summarized by Saper and colleagues and schematically in Fig. 19-4, taken from their publication. The current conceptualization is one of an unstable “flip-flop” switch that depends on mutual inhibition of the monoaminergic system and the VLPO. The state of the switch is indirectly stabilized by the orexin neurons. In this model, the awake state is maintained by monoaminergic activity (locus ceruleus, tuberomammillary nucleus [TMN], and the raphe nuclei) that inhibits the VLPO. Sleep occurs when the VLPO is activated, which reciprocally removes the tonic inhibitory action of the monoaminergic system. The orexin neurons act through the monoaminergic system as a stabilizing influence to prevent rapid transitions from one state to the other.
Figure 19-4.
Schematic representation of the “flipflop” mechanism of transition between sleep and waking, which is determined by the state of activity of the ventrolateral preoptic nucleus (VLPO). A. During wakefulness, the monoaminergic nuclei (LC, locus ceruleus; TMN, tuberomammillary nucleus; raphe nuclei) inhibit the VLPO, thereby relieving the inhibition of the monoaminergic cells, and that of the orexin (ORX) neurons. Because the VLPO neurons do not have orexin receptors, orexin serves to reinforce the monoaminergic tone, rather than directly inhibiting the VLPO. B. During sleep, firing of VLPO neurons inhibits the monoaminergic cell groups, thereby relieving their own inhibition. This inhibits the orexin neurons, further preventing monoaminergic activation that might interrupt sleep. The mutual inhibition between the VLPO and the monoaminergic cell groups forms a flip-flop switch, which produces sharp transitions in state, but is relatively unstable. The addition of the orexin neurons stabilizes the switch. (Reproduced with permission from Saper, Scammell, and Lu.)
Apart from the overall diurnal sleep cycle, evidence from animal studies suggests that the physiologic mechanisms and transitions between NREM and REM sleep are governed by the pontine reticular formation and are influenced by acetylcholine. Cholinergic neurons are found in two major loci in the parabrachial region of the dorsolateral pontine tegmentum—in the pedunculopontine group of nuclei and the lateral dorsal tegmental group. The cholinergic cell groups project rostrally, but the precise anatomy of this projection system has not been defined. Cells from these groups make up parts of the ascending reticular activating system.
Despite the heuristic value of Hobson’s reciprocal interaction hypothesis, some of its features remain controversial. Although it is generally agreed that cholinergic mechanisms selectively promote REM sleep, and its components—rapid eye movements, absence of activity in the antigravity muscles (i.e., atonia), and desynchronized EEG—the role of amines has been more difficult to establish. Thus, lesions of the locus ceruleus and raphe nuclei, which contain neurons rich in norepinephrine, do not greatly alter REM sleep. Nevertheless, a considerable body of pharmacologic data suggests that a decrease in monoamines causes an increase in REM activity and vice versa. Insofar as the bulk of cholinergic and aminergic neurons are found in the pedunculopontine group of nuclei, Shiromani and colleagues have suggested that interaction between these neurons occurs in the region of the pedunculopontine nuclei rather than in the medial pontine reticular formation, as suggested by Hobson and associates.
Solms (1995, 1996) and others have questioned the traditional view that dreaming and REM sleep are obligately or even closely connected and has presented an alternative model. Among patients with cerebral lesions that eliminate or disrupt REM sleep, he cites several cases in which dreaming was retained. Conversely, in 9 of his patients with basal forebrain (frontal) lesions, dreaming was lost, at least for a time, while REM periods remained undisturbed through the night. This same observation had been made in patients who had prefrontal leukotomies. Solms has proposed that the dopaminergic systems in the basal forebrain areas elicit or modulate dreaming. This view is supported by reports of diminished dreaming in patients being treated with dopaminergic blockers and the enhancement of dreaming reported by patients taking L-dopa or dopamine agonsits. Notable in this regard is the fact that major intracortical dopaminergic pathways originate in the frontal lobes.
In regard to the neurophysiology of the EEG and sleep rhythms, much has been gleaned from intracellular recordings in animals. As with the earlier-described anatomic and neurochemical data, the degree to which similar electrophysiologic changes are reflected in humans is not known. Most of the integrated rhythms of sleep that are recorded at the surface of the brain, including the background activity of slow-wave sleep and the faster and more synchronized sleep spindles and vertex waves, have their origins in the thalamus. Steriade and colleagues have performed a substantial amount of the modern work in this area and summarize it in their review. They make clear that these complex EEG rhythms, while apparent in nascent form in certain isolated neurons, are oscillations that arise from integrated ensembles of cells, with the thalamus as a nexus. It is evident that there is as yet no agreement concerning the integration of all these brainstem and thalamic–hypothalamic mechanisms in the production of sleep or of dreams.
These questions have been pondered endlessly by physiologists and psychiatrists (and philosophers). Parkes has reviewed the main theories—body restitution, facilitation of motor function, consolidation of learning and memory—and tends to agree with the ungrammatical but unambiguous conclusion of Popper and Eccles that “Sleep is a natural repeated unconsciousness that we do not even know the reason for.” There is no convincing proof, for example, of the popular notion that we stabilize learned material while asleep, nor can one logically entertain the notion that the function of sleep is to produce dreams, at least until the utility and meaning of dreams become known.
Regarding neurophysiologic changes during dreaming, Braun and colleagues used positron emission tomography (PET) to study REM sleep; they observed selective activation of the extrastriate visual cortices and limbic–paralimbic regions, with attenuation of activity in the primary visual cortex and frontal association areas. Based on these and similar studies, several authors have speculated that the suppression of frontal lobe activity during dreaming, at a time when visual association areas and their paralimbic connections are activated, might explain the uncritical acceptance of the bizarre visual content, the disordered temporal relationships, and the heightened emotionality that characterize dreams. This would be in keeping with Hobson’s view of dreams as a form of delirium that is appealing to us. As an alternative that links dreams to inherent meaning for the individual, Solms suggested that activation of frontal dopaminergic systems during dreaming, the same pathways that participate in most biologic drives, implies that dreams express latent wishes and drives—a psychoanalytical interpretation expressed by Freud in his book The Interpretation of Dreams.
Deprived of sleep, experimental animals will die within a few weeks, no matter how well they are fed, watered, and housed (Rechtschaffen et al), but whether a similar degree of sleep deprivation leads to death in humans is unknown. Nevertheless, humans beings deprived of sleep do suffer a variety of very unpleasant symptoms quite distinct from the effects of the usual types of insomnia.
Despite many studies of the deleterious emotional and cognitive effects of sleeplessness, we still know little about them. If deprived of sleep (NREM and REM) for periods of 60 to 200 h, human beings experience increasing sleepiness, fatigue, irritability, and difficulty in concentration. Performance of skilled motor activities also deteriorates; if the tasks are of short duration and slow pace, the subject can manage them, but if speed and perseverance are demanded, he cannot. Self-care is neglected, incentive to work wanes, sustained thought and action are interrupted by lapses of attention, judgment is impaired, and the subject becomes decreasingly inclined to communicate. With sustained deprivation, sleepiness becomes increasingly more intense, momentary periods of sleep (“microsleep”) become more intrusive, and the tendency to all types of accidents becomes more marked. Eventually, subjects fail to perceive inner and external experiences accurately and to maintain their orientation. Illusions and hallucinations, mainly visual and tactile ones, intrude into consciousness and become more persistent as the period of sleeplessness is prolonged. This may be a component of the decompensation of individuals with bipolar psychiatric disease, sometimes triggering manic episodes.
Neurologic signs of sleep deprivation include a mild and inconstant nystagmus, impairment of saccadic eye movements, loss of accommodation, exophoria, a slight tremor of the hands, ptosis of the eyelids, expressionless face, and thickness of speech, with mispronunciations, and incorrect choice of words. The EEG shows a decrement of alpha waves, and closing of the eyes no longer generates alpha activity. The seizure threshold is reduced, and seizure foci in the EEG may be activated. Rarely and probably only in predisposed persons, loss of sleep provokes a psychotic episode (2 to 3 percent of 350 sleep-deprived patients studied by Tyler); however, many sleep specialists dispute the production of psychosis.
During recovery from prolonged sleep deprivation, the amount of sleep obtained is never equal to the amount lost. This is probably a result of the intrusion of brief sleep periods during the waking state and represents a sizable amount of time if summated (it is virtually impossible to deprive a human being or animal totally of sleep). When falling asleep after a long period of deprivation, the subject rapidly enters N3 (NREM) sleep, which continues for several hours at the expense of N2 and REM sleep. But by the second recovery night, REM sleep rebounds and exceeds that of the predeprivation period. N3 seems to be the most important sleep stage in restoring the altered functions that result from prolonged sleep deprivation.
The effects of differential REM sleep deprivation are more difficult to interpret than the effects of total or near-total deprivation. Some subjects in whom REM sleep is prevented night after night show an increasing tendency to hyperactivity, emotional lability, and impulsivity—a state that has been compared to the heightened activity, excessive appetite, and hypersexuality of animals deprived of REM sleep. However, in humans, monoamine inhibitors are able to completely suppress REM sleep for months to years without obvious harm. Differential deprivation of NREM sleep (N3) leads, instead, to hyporesponsiveness and excessive daytime sleepiness as noted.
Because the need for sleep varies considerably from person to person, it is difficult to decide what constitutes sleep deprivation. Certain individuals apparently function well on 4 h or even less of sleep per 24-h period, and others, who sleep long hours, claim not to obtain maximum benefit from it.
Sleep Disorders
The term insomnia signifies a chronic inability to sleep despite adequate opportunity to do so; it is used popularly to indicate any impairment in the duration, depth, or restorative properties of sleep. There may be difficulty in falling asleep or remaining asleep, awakening may come too early, or there may be a combination of these complaints. Precision as to what constitutes pathologic insomnia is impossible at the present time because of our uncertainty as to the exact amounts of sleep required, and the role of sleep in the economy of the human body. All that can be said is that some form of sleeplessness is a frequent complaint (20 to 40 percent of the population) and is more prominent in the elderly and in women. Only a small proportion of persons who perceive their sleep to be inadequate seek professional help or use sleeping pills, according to Mellinger and colleagues.
Two general classes of insomnia can be recognized—one in which there appears to be a primary abnormality of the normal sleep mechanism, and another in which the sleep disturbance is secondary to, or perhaps more accurately comorbid with, a medical or psychologic disorder. Polysomnographic studies have defined yet another subgroup who actually sleep enough, but who perceive their sleep time to be shortened or disrupted (“paradoxical insomnia”).
This term is reserved for the condition in which nocturnal sleep is disturbed for prolonged periods and none of the symptoms of anxiety, depression, pain, or other psychiatric or medical diseases can be invoked to explain the sleep disturbance. In some patients, like those described by Hauri and Olmstead, the disorder is lifelong. Unlike the rare individuals who seem to be satisfied with 4 h or even less of sleep a night, insomniacs suffer the effects of partial sleep deprivation and resort to medications, alcohol, and their lives come to revolve around sleep to such an extent that they have been called “sleep pedants” or “sleep hypochondriacs.” Although statements on the quantity and quality of sleep given by insomniacs are often not to be taken as entirely valid, Rechtschaffen and Monroe have confirmed that most of them do, indeed, sleep for shorter periods, move and awaken more often, spend less time in N3 sleep than normal persons, and show a heightened physiologic arousal. Personality inventories have disclosed a high incidence of psychologic disturbances in this group, but whether these are cause or effect is not clear.
Furthermore, a category of “conditioned’, or “psychophysiological” insomnia has been denominated, in which a situational trigger for insomnia has ceased, but the sleep disorder persists. Although insomniacs, regardless of the cause, tend to exaggerate the amount of sleep lost, primary insomnia should be recognized as an entity and not passed off as a neurotic quirk.
This common type of insomnia, which is often transitory, can be ascribed to pain or some other recognizable bodily disorder, such as drug or alcohol abuse or, most commonly, to anxiety, worry, or depression. Of the medical disorders conducive to abnormal wakefulness, certain ones stand out—pain in the joints or in the spine, abdominal discomfort from peptic ulcer and carcinoma, pulmonary and cardiovascular insufficiency, and the nocturia engendered by prostatism. The “restless legs” syndrome and periodic leg movements of sleep are not considered in this category and have their own physiology, phenomenology, and treatment.
The disorder known as the restless legs syndrome may regularly delay the onset of sleep and usually occurs in its early stages. Ekbom called it asthenia crurum paresthetica and also, anxietas tibiarum. This disorder is surprisingly prevalent, affecting more than 2 percent of the population. The patient may complain of unpleasant aching and drawing sensations in the calves and thighs, often associated with creeping or crawling feelings; other descriptions have included “worms,” “internal itch,” and “coldness,” and the legs may feel tired, heavy, and weak. The symptoms are provoked by rest, and rapidly, but temporarily, relieved by moving the legs. An urge to move the legs can be suppressed voluntarily for a brief period but is ultimately irresistible. Moving the limbs alleviates the sensation briefly. It is interesting that a small proportion of patients have similar symptoms in the arms after many years of symptoms. There may be variants of nocturnal restlessness in other parts of the body such as the abdomen, as suggested by Pérez-Díaz and colleagues. Their patients described an unpleasant abdominal musculature restlessness that required movement for relief and was eliminated with dopamine agonists.
Fatigue worsens restless legs syndrome, and there is a tendency for it to be worse in warm weather. In a few patients, mainly older ones with a severe form of the nighttime disorder, these movements and an associated myoclonus spill over into wakefulness and are accompanied by restlessness, foot spasms, foot stamping, body rocking, and marching that are only partly under voluntary control. The daytime phenomena may require several medications used simultaneously for control.
The syndrome is idiopathic and persists for years. Iron-deficiency anemia and low ferritin levels are associated with the syndrome in many instances, as is thyroid disease, pregnancy, and certain drugs, such as antidepressants and antihistamines. Occasionally, it is a prelude to a peripheral neuropathy, particularly in relation to uremia. Also, reduced levels of iron in the cerebrospinal fluid (CSF) have been found with restless legs syndrome and with periodic limb movements of sleep (see below). The basis for this relationship is not well defined, but it makes it advisable to check for reduced iron stores and anemia in most patients. One hypothesis is that a disturbance of iron storage in the basal ganglia causes a decrement in dopamine binding by dopamine receptors and transporters, as has been described in studies using PET and single-photon emission tomography (SPECT) scans. Another potential relationship, unproved, is that iron is a cofactor for the enzyme, tyrosine hydroxylase, which is required to produce dopamine.
A closely related disturbance is periodic leg movements of sleep. Like the restless legs syndrome, it may result in sleep deprivation and daytime somnolence or, more often, in disturbance of a bed partner. However, the diagnosis of periodic leg movements depends on finding them during polysomnographic recordings, whereas restless leg syndrome is identified on clinical grounds. Originally described as “nocturnal myoclonus,” periodic leg movements are slower than myoclonic jerks. They consist of a series of repetitive movements of the feet and legs occurring every 20 to 90 s for several minutes to an hour; mainly the anterior tibialis is involved, with dorsiflexion of the feet and big toes, sometimes followed by flexion of the hip and knee. The movements are similar to the triple-flexion (Babinski) response, which can be elicited in normal sleeping persons. These movements produce frequent microarousals or, if severe and periodic, full arousals. The patient, usually unaware of these sleep-related movements at the time they occur, is told of them by a bed mate or suspects their occurrence from the disarray of the bedclothes. Periodic leg movement is closely associated with the restless legs syndrome and many sleep specialists consider it an integral part of the syndrome, but it also occurs independently with narcolepsy, sleep apnea, following the use of tricyclic and serotonin reuptake inhibiting antidepressants, L-dopa, and withdrawal from anticonvulsants and sedative-hypnotic drugs. Approximately eighty percent of individuals with restless leg syndrome will display periodic leg movements, but the opposite is not the case, as only twenty to thirty percent of patients with periodic leg movements have restless leg syndrome.
A seminal genetic finding by Stefansson and colleagues derived from several populations, including the homogenous Icelandic, is that a nucleotide variant in a short segment of chromosome 6p is associated with periodic leg movements of sleep. This was found to hold in those with and without restless legs syndrome. If nothing else, as pointed out by the authors, this establishes that periodic limb movements are a distinct entity as defined in the era of genomics. The biologic significance and frequency in other populations of this variant is not yet known. Nonetheless, we continue to be impressed at the frequent cooccurrence of the two conditions and several shared underlying conditions such as iron deficiency, and treatments that are effective in both.
A search for iron deficiency, and its correction if present, is indicated in almost all cases. A large number of symptomatic medications have proved helpful in the treatment of both the restless legs syndrome and periodic leg movements. As a first choice, many practitioners favor treatment with dopamine agonists such as pramipexole (0.25 to 0.75 mg) or ropinirole (0.5 to 1.5 mg), either one taken 1.5 to 2 h before bedtime. Long-acting combinations of L-dopa/carbidopa (12.5/50 or 25/100 mg dose) taken at bedtime have also been successful, but L-dopa, and sometimes the dopamine agonists, causes some patients to develop the movements earlier, i.e., in the daytime, which become more intense and spread to other body parts. A longer acting dopamine agonist, rotigotine patch is available to treat patients who have this augmentation phenomenon. The latter is also helpful for periodic leg movements.
A major problem, recently recognized, is one of “augmentation,” or enhancement of the restless leg syndrome with the long-term use of this class of drugs. This is less prominent with some of the other numerous drugs that have been effective including gabapentin, pregabalin, clonazepam (0.5 to 2.0 mg), temazepam (30 mg), taken 30 min before retiring and extended release gabapentin which are available to minimize the augmentation phenomenon. Other drugs—e.g., baclofen, opioids, carbamazepine seem to be helpful in certain patients, but they are infrequently required. The lengthy list of medications that have been effective is given in Earley’s review. It is sometimes useful to give a medication in 2 divided doses, the first early in the evening, and the second just before sleep or, in severe cases, during the night by setting an alarm clock before the anticipated time of symptoms.
Among the secondary insomnias, those caused by some type of psychologic disturbance are particularly common. Domestic or business worries may keep the patient’s mind in turmoil (situational insomnia). A strange bed or unfamiliar surroundings may prevent drowsiness and sleep. Under these circumstances, the main difficulty is in falling asleep, with a tendency to sleep late in the morning. These facts emphasize that conditioning and environmental factors (social and learned) are normally involved in readying the mind and body for sleep.
Illnesses in which anxiety and fear are prominent symptoms also result in difficulty in falling asleep and in light, fitful, or intermittent sleep. Disturbing dreams are frequent in these situations and may awaken the patient. Exceptionally, a patient may even try to stay awake in order to avoid them. In contrast, depressive illness produces early morning waking and inability to return to sleep; the quantity of sleep is reduced, and nocturnal motility is increased. REM sleep in depression, although not always reduced, comes earlier in the night. If anxiety is combined with depression, there is a tendency for both the above patterns to be observed. Yet another common pattern of disturbed sleep can be discerned in individuals who are under great tension and worry or are overworked and tired out. These people sink into bed and sleep through sheer exhaustion, but they awaken early with their worries and are unable to get back to sleep.
Chronic and even short-term use of alcohol, barbiturates, and certain nonbarbiturate sedative-hypnotic drugs markedly reduces REM sleep as well as stages 3 and 4 of NREM sleep (N3). Following withdrawal of these drugs, there is a rapid and marked increase of REM sleep, sometimes with vivid dreams and nightmares. “Rebound insomnia,” a worsening of sleep compared with pretreatment levels, has also been reported upon discontinuation of short-half-life benzodiazepine hypnotics, notably triazolam (Gillin et al) and including the newer sleep agents mentioned below. Furthermore, a form of drug-withdrawal or rebound insomnia may actually occur during the same night in which the drug is administered. The drug produces its hypnotic effect in the first half of the night and a worsening of sleep during the latter half of the night, as the effects of the drug wear off; the patient and the physician may be misled into thinking that these latter symptoms require more of the hypnotic drug or a different one. Alcohol taken in the evening acts in the same way. Rebound insomnia must be distinguished from the early morning awakening that accompanies anxiety and depressive states.
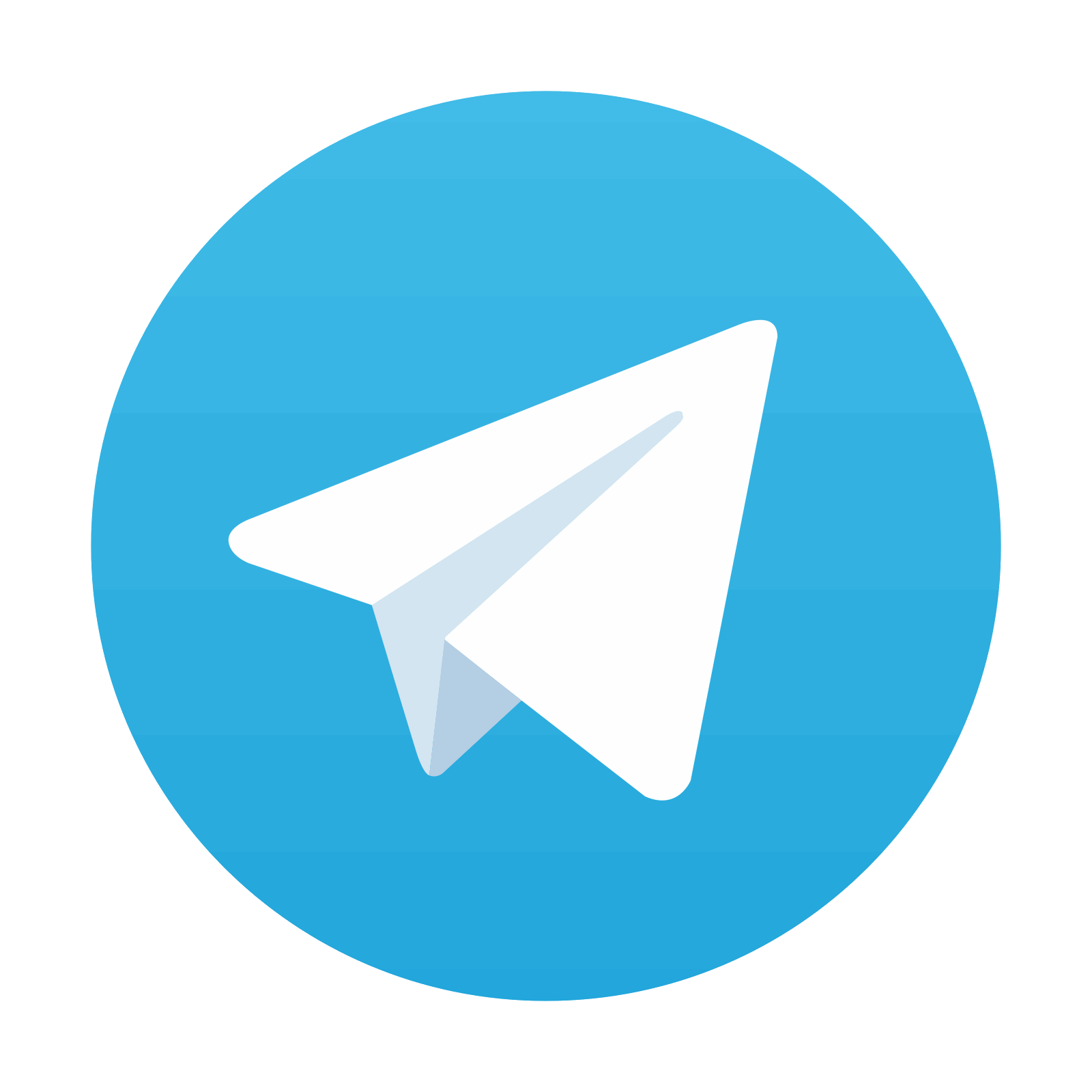
Stay updated, free articles. Join our Telegram channel

Full access? Get Clinical Tree
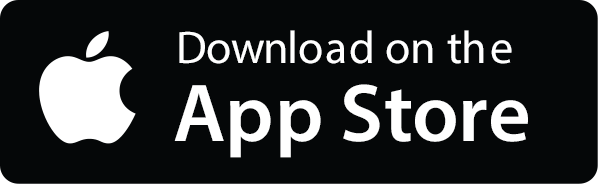
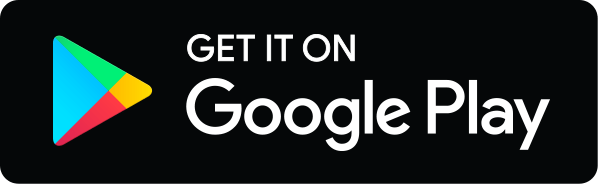