Craniocerebral Trauma: Introduction
Among the vast array of neurologic diseases, cerebral trauma ranks high in order of frequency and gravity. In the United States, trauma is the leading cause of death in persons younger than 45 years of age and more than half of these deaths are a result of head injuries. According to the American Trauma Society, an estimated 500,000 Americans are admitted to hospitals yearly following cerebral trauma; of these, 75,000 to 90,000 die and even larger numbers, most of them young and otherwise healthy, are left permanently disabled.
The basic problem in craniocerebral trauma is at once both simple and complex: simple because there is usually no difficulty in determining causation, namely, a blow to the head, and complex because of a number of delayed effects that complicate the injury. As for the trauma itself, little can be done, for it is finished before the physician or others arrive on the scene. At most there can be an assessment of the full extent of the immediate cerebral injury, an evaluation of factors conducive to complications and further lesions, and the institution of measures to avoid such additional problems. Specifically, the neck can be stabilized and adequate perfusion and oxygenation can be secured. But of the disastrous intracranial phenomena that can be initiated by head injury, few offer possibilities of treatment. New techniques of cellular biology are exposing phenomena that are set in motion by traumatic injury of nerve cells and glia. Some of these changes may be reversible, but at the moment, such knowledge is limited.
It is a common misconception that craniocerebral injuries are matters that concern only the neurosurgeon and not the general physician or neurologist. Actually, 80 percent of head injuries are first seen by a physician in an emergency department, and fewer than 20 percent ever require neurosurgical intervention of any kind, and even this number is decreasing. The neurologist must be familiar with the clinical manifestations and the natural course of primary brain injury and its complications and have a sound grasp of the underlying physiologic mechanisms. Such knowledge must also relate to the interpretation of CT and MRI, both of which have greatly enhanced our ability to deal with traumatic brain injury. The present chapter reviews the salient facts concerning craniocerebral injuries and outlines a clinical approach that the authors have found useful over the years. Matters pertaining to spinal injuries, often coexistent with head trauma, are considered in Chap. 44.
Definitions and Mechanisms
The very language that one uses to discuss certain types of head injuries divulges a number of misconceptions inherited from previous generations of physicians. Certain terms have crept into the medical vocabulary and have been retained long after the ideas for which they stood have been refuted, attesting to the disadvantage of premature adoption of explanatory terms rather than descriptive ones. The word concussion, for example, implies a violent shaking or jarring of the brain and a resulting transient functional impairment. Yet despite numerous postulates of physical changes within nerve cells, axons, or myelin sheaths (vibration effects, formation of intracellular vacuoles) that putatively occurred with concussion, confirmation of their existence has proved difficult in humans and experimental animals.
In all attempts to analyze the mechanisms of closed, or blunt (nonpenetrating), head injury, one fact is preeminent: there must be the sudden application of a physical force of considerable magnitude to the head. Unless the head is struck, the brain suffers no injury except in the rare instances of violent flexion–extension (whiplash) of the neck and possibly in explosion-blast injury with a sudden extreme increase of atmospheric pressure. In military medical practice, blast injuries assume great importance and in theory, challenge many concepts of loss of consciousness in closed head injury; i.e., there is no contact with, or sudden acceleration or deceleration of the cranium. The mechanical factors of importance in brain injury are the differential mobility of the head on the neck, and of the brain within the cranium, the tethering of the upper brainstem that allows movement of the cerebral hemispheres around that vertex, and the striking of parts of the brain on dural septa and bony prominences. As to concussive injuries, it is useful to point out that concussions usually involve a physical force that imparts motion to the stationary head or more commonly, a hard surface that arrests the motion of a moving head, i.e., concussion does not occur if the head remains stationary. This sudden deceleration or acceleration of the cranium is the mechanism of most civilian head injuries, and they are notable in two respects: they often induce at least a temporary loss of consciousness, and the brain may suffer gross damage even though the skull is not penetrated, i.e., contusion, laceration, hemorrhage, and swelling. A theory that brings coherence to all of these physical and gross neuropathologic changes and their relation to transient loss of consciousness (concussion) and prolonged coma has only been tentatively formulated.
In contrast to closed head injury, high-velocity missiles penetrate the skull and cranial cavity, or rarely, the skull may be compressed between two converging forces that crush the brain without causing significant displacement of the head or the brain. In these circumstances, the patient may suffer severe and even fatal injury without preceding loss of consciousness. Hemorrhage, destruction of brain tissue, and, if the patient survives for a time, meningitis or abscess are the principal pathologic changes created by injuries of these types. They offer little difficulty to our understanding. Figure 35-1 illustrates these various types of head injuries.
Figure 35-1.
Mechanisms of craniocerebral injury. A. Cranium distorted by forceps (birth injury). B. Gunshot wound of the brain. C. Falls (also traffic accidents). D. Blows on the chin (“punch-drunk”). E. Injury to skull and brain by falling objects. (From Courville.)
The relation of skull fracture to brain injury has been viewed in changing perspective throughout the history of this subject. In the first half of the last century, fractures dominated the thinking of the medical profession, and cerebral lesions were regarded as secondary. Later, it became clear that the skull, although rigid, is still flexible enough to yield to a blow that injures the brain without causing fracture. Therefore the presence of a fracture, although a rough measure of the force to which the brain has been exposed, is not an infallible index of the presence of cerebral injury (see further on in discussion of predictive features for imaging abnormalities with concussion). Even in immediately fatal head injuries, autopsy reveals an intact skull in 20 to 30 percent of cases. Of course, many patients suffer skull fractures without serious or prolonged disorder of cerebral function, partly because the energy of a blow is dissipated in the fracture. Indeed, this diffusion of the impact might be expected to reduce underlying brain damage.
Nevertheless, fractures cannot be dismissed without further comment for several reasons. Overall, brain injury is estimated to be 5 to 10 times more frequent with skull fractures than without them and perhaps 20 times more frequent with severe and multiple fractures. Fractures assume further importance in providing an explanation for cranial-nerve palsies, and in creating potential pathways for the ingress of bacteria and air or the egress of cerebrospinal fluid (CSF leak). In these respects, fractures through the base of the skull are of special significance, and are considered below.
Figure 35-2 illustrates the major sites and directions of basilar skull fractures. One can readily perceive the possibilities of injury to cranial nerves. Fractures of the base are difficult to detect in plain skull films and may be missed by other imaging techniques, but their presence should be suspected in the presence of any one of a number of characteristic clinical signs. Fracture of the petrous pyramid often deforms the external auditory canal or tears the tympanic membrane, with resultant leakage of CSF (otorrhea); or, blood may collect behind an intact tympanic membrane and discolor it. If the fracture extends more posteriorly, damaging the sigmoid sinus, the tissue behind the ear and over the mastoid process becomes boggy and discolored (Battle sign). Basal fracture of the anterior skull may also cause blood to leak into the periorbital tissues, imparting a characteristic “raccoon” or “panda bear” appearance. The presence of any of these signs calls for CT scanning of the skull base using bone window settings to detect a fracture.
Figure 35-2.
The course of fracture lines through the base of the skull. Arrows indicate point of application and direction of force. (From Courville.)
The existence of a basal fracture is also indicated by signs of cranial nerve damage. The olfactory, facial, and auditory nerves are the ones most liable to injury, but any one, including the twelfth, may be damaged. Anosmia and an apparent loss of taste (actually a loss of perception of aromatic flavors as the elementary modalities of taste are unimpaired) are frequent sequelae of head injury, especially with falls on the back of the head. In the majority of cases the anosmia is permanent. If unilateral, it will not be noticed by the patient. However, mechanism of these disturbances is thought to be a displacement of the brain and tearing of the olfactory nerve filaments in or near the cribriform plate, through which they course, rather than being attributable to a fracture. A fracture in or near the sella may tear the stalk of the pituitary gland with resulting diabetes insipidus. Rarely, such a fracture may cause bleeding from a preexisting pituitary adenoma and produce the syndrome of pituitary apoplexy (see Chap. 31). A fracture of the sphenoid bone may lacerate the optic nerve, with blindness from the beginning. The pupil is unreactive to a direct light stimulus but still reacts to a light stimulus to the opposite eye (consensual reflex). The optic disc becomes pale, i.e., atrophic, after an interval of several weeks. Partial injuries of the optic nerve result in scotomas and a troublesome blurring of vision.
Complete oculomotor nerve injury is characterized by ptosis and diplopia, a divergence of the globes with the affected eye resting in an abducted and slightly depressed position, loss of medial and most of the vertical movements of the eye, and a fixed, dilated pupil, as described in Chap. 13. The most common symptom is diplopia that is worse on looking down and compensatory tilting of the head indicating a trochlear nerve injury. In a series of 60 patients with head injury, Lepore confirmed that fourth-nerve palsy was the most common cause of diplopia, occurring unilaterally twice as often as bilaterally, followed in frequency by damage to one or both third nerves, then, least often, a unilateral or bilateral sixth-nerve palsy. Five of his patients had palsies that reflected damage to more than one nerve and seven had supranuclear disorders of convergence. The long, circumferential subarachnoid course of the fourth nerve is usually given as the explanation for its frequent injury, but this mechanism has never been validated. These optic and ocular motor nerve disorders must be distinguished from those caused by displacement of the globe or entrapment of an extraocular muscle as a result of direct injury to the orbit.
Injury to the ophthalmic and maxillary divisions of the trigeminal nerve may be the result of either a basal fracture across the middle cranial fossa or a direct extracranial injury to the branches of the nerves. Numbness and paresthesia of the skin supplied by the nerve branch or chronic neuralgia can be troublesome sequelae of these injuries.
The facial nerve may be involved in one of two ways. In the first type of injury, associated with transverse fractures through the petrous bone, there is an immediate facial palsy, probably caused by contusion or transection of the nerve. Surgical anastomosis has sometimes been successful in restoring function in this circumstance. The second, more common type, is associated with longitudinal fractures of the petrous bone, the facial palsy then often being delayed for several days, a sequence that may be misinterpreted as progression of the intracranial traumatic lesion. This latter type is usually transitory, and its mechanism is not known.
Injury to the eighth cranial nerve because of petrous fractures results in a loss of hearing or in postural vertigo and nystagmus coming on immediately after the trauma. Deafness as a result of nerve injury must be distinguished from the high-tone hearing loss due to cochlear injury and from deafness caused by bleeding into the middle ear and disruption of the ossicular chain (conduction deafness). Also, vertigo must be distinguished from the very common symptom of posttraumatic dizziness discussed in a later section. The rare condition of fracture through the hypoglossal canal causes weakness of one side of the tongue. It should be kept in mind that blows to the upper neck may also cause lower cranial-nerve palsies, either by direct injury to their peripheral extensions or as a result of carotid artery dissection in the cervical segment of the artery.
A basal fracture through the sphenoid bone may lacerate the internal carotid artery or one of its intracavernous branches where it lies in the cavernous sinus. Within hours or a day or two, a disfiguring pulsating exophthalmos develops as arterial blood enters the sinus and distends the superior and inferior ophthalmic veins that empty into the sinus. The orbit feels tight and painful, and the eye may become partially or completely immobile because of pressure on the ocular nerves traversing the sinus (see Fig. 14-5). The sixth nerve is affected most often, and the third and fourth nerves less often. Also, there may be a loss of vision as a result of ischemia of the optic nerve and retina, although the mechanism has not been entirely clear; congestion of the retinal veins and glaucoma are potential factors in the visual failure. Some 5 to 10 percent of fistulas resolve spontaneously, but the remainder must be obliterated by interventional radiologic means (by a detachable balloon inserted into the carotid artery via a transfemoral catheter) or by a direct surgical repair of the fistula (see Stern).
Not all carotid–cavernous fistulas are traumatic. They may occasionally occur with rupture of an intracavernous saccular aneurysm or in Ehlers-Danlos disease, where the connective tissue is defective; or the cause may be unexplained. Occasionally, a dural-based arteriovenous fistula opens in the region of the cavernous sinus after an injury; most such cases are spontaneous and they cause less in the way of orbital swelling and congestion.
If the skin over a skull fracture is lacerated and the underlying meninges are torn, or if the fracture passes through the inner wall of a paranasal sinus, bacteria may enter the cranial cavity, with resulting meningitis or abscess formation. CSF that leaks into the sinus presents as a watery discharge from the nose (CSF rhinorrhea). The nasal discharge can be identified as CSF by testing it for glucose with diabetic test tape (mucus has no glucose) or by the presence of fluorescein or radionuclide-labeled dye that is injected into the lumbar subarachnoid space and then absorbed by pledgets placed in the nasal cavity. Mucus, when absorbed onto a handkerchief and allowed to dry, will leave the material stiff, whereas CSF, will not. A more elaborate test is to detect tau protein in the discharge; it is present only in CSF and not in mucus or blood. Most cases of acute CSF rhinorrhea heal by themselves. An indwelling lumbar drain for a few days may aid the process. If the condition is persistent or is complicated by an episode of meningitis, repair of the torn dura is indicated. The prophylactic use of antibiotics to prevent meningitis in cases of nasal CSF leak is controversial, but many neurosurgeons continue this practice, particularly in children.
A collection of air in the cranial cavity (aerocele) is a common occurrence following skull fracture or any extended neurosurgical procedure. The pocket of air is apparent by CT scan in the epidural or subdural space over the cerebral convexities or between the hemispheres, and serves only to warn of a potential route for the entry of bacteria into the cranium. Small collections of air are usually absorbed without incident, but a large volume may act as a mass and cause clinical deterioration after injury (tension pneumocranium; Fig. 35-3). Inhalation of 100 percent oxygen has a slight salutary effect, but aspiration of the air is required if the collection is causing clinical signs.
Depressed skull fractures are of significance only if the underlying dura is lacerated or the brain is compressed by indentation of bone. They then are surgically elevated, preferably within the first 24 to 48 h.
Much has been written about the mechanisms of concussion in closed head injury and its definition has undergone serial revision. In the past, a transient loss of consciousness and amnesia after a blow to the head had been considered necessary to qualify as concussion but lesser degrees of mild confusion, incoordination, or even symptoms such as headache and fatigue that follow mild head injury are now encompassed under the term. Whether all these problems derive from the same mechanism cannot be stated with confidence.
The mechanism of concussive “cerebral paralysis” has been interpreted in various ways throughout medical history in light of the state of knowledge at a particular period of time. The favored hypotheses for the better part of a century were “vasoparalysis” (suggested by Fischer in 1870) or an arrest of circulation by an instantaneous rise in intracranial pressure (ICP) (proposed by Strohmeyer in 1864 and popularized by Trotter in 1932). Jefferson, in his essay on the nature of concussion (1944), convincingly refuted these vascular hypotheses. Later, Shatsky and coworkers, by the use of high-speed cineangiography, showed displacement of vessels but no arrest of circulation immediately after impact.
Beginning with the work of Denny-Brown and Russell in 1941, the physical factors involved in head and brain injuries were subjected to careful analysis. These investigators demonstrated that in monkey and cat the concussion resulted when the freely moving head was struck by a heavy mass. If the head was prevented from moving at the moment of impact, the same degree of force invariably failed to produce concussion. The importance of head motion was verified by Gennarelli and colleagues, who were able to induce concussion in primates by rapid acceleration of the freely moving head without impact, a condition that rarely occurs in humans.
Holbourn, a Cambridge physicist, from a study of gelatin models under conditions simulating head trauma, deduced that when the head is struck, movement of the partly tethered but suspended brain always lags (because of inertia), but inevitably the brain moves also, and when it does it must rotate in an arc because of attachment to the neck. Ommaya and Gennarelli (1974) proved the correctness of this assumption by photographing the brain through a transparent calvarium at the moment of impact. The brain was thus subjected to stresses set up by rotational forces mainly in the sagittal plane, centered at its point of tethering in the high midbrain. The torque at the level of the upper reticular formation would explain the immediate loss of consciousness, as described later. An extensive and scholarly review of the pathophysiology of concussion was done by Shaw (although we are uncertain of the validity of his view of a seizure-concussive mechanism).
The core features of loss of consciousness or confusion are notable for being immediate after trauma (not delayed even by seconds) and for being entirely reversible. This is the sense in which concussion was used to mean a reversible traumatic paralysis of nervous function and any physiologic explanation of the syndrome would have to incorporate this temporal sequence. However, the effects of concussion on brain function may last for a variable time (seconds, minutes, hours, or longer) and to set arbitrary limits on the duration of loss of consciousness, i.e., to consider a brief loss as indicative of concussion and a prolonged loss as indicative of contusion or other traumatic cerebral lesion, is unsound physiologically. As pointed out by Symonds, any such difference is quantitative, not qualitative. It is true that in the more prolonged states of stupor or coma, there is a far greater chance of finding hemorrhage and contusion, which undoubtedly contribute to the persistence of coma and the likelihood of irreversible change. Finally, the optimal condition for the production of concussion, demonstrated originally by Denny-Brown and Russell, is a sudden change in the momentum of the head; i.e., either movement is imparted to the stationary head by a blow or movement of the head is arrested by a hard, unyielding surface.
Rotational movements of the brain also provide a reasonable explanation for the occurrence of surface injuries in specific locations, i.e., where the swirling brain comes into contact with bony prominences on the inner surface of the skull (petrous and orbital ridges, sphenoid wings), and of injuries to the corpus callosum, which is flung against the falx. Not well explained by any of these mechanisms are concussions after blast injuries, a serious problem in military medicine. This syndrome possibly resurrects the notion that a shock wave travels through the brain and disrupts neural function throughout the cerebral hemispheres or in the reticular formation of the midbrain.
These views on the site and mechanism of concussion are not fully accepted but have been supported by a number of additional physiologic observations. Foltz and Schmidt, in 1956, suggested that the reticular formation of the upper brainstem was the anatomic site of concussive injury. They showed that in the concussed monkey, lemniscal sensory transmission through the brainstem was unaltered, but its effect in activating the reticular formation was blocked and that the electrical activity of the medial reticular formation was depressed for a longer time and more severely than that of the cerebral cortex.
What was further noteworthy in most of these cases, and in those reported by Jellinger and Seitelberger, was the presence of additional lesions in the region of the reticular activating system and small hemorrhagic softenings in the corpus callosum, superior cerebellar peduncles, and dorsolateral tegmentum of the midbrain. As discussed further on, Strich (1956) interpreted the extensive white matter lesions, both in the hemispheres and in the upper brainstem, to represent a degeneration of nerve fibers that had been stretched or torn by the shear stresses set up during rotational acceleration of the head, as had been postulated earlier by Holbourn. She suggested that if nerve fibers are stretched rather than torn, the lesions may be reversible and may play a part in the mechanism of concussion. Symonds elaborated on this view and saw in the shearing stresses, which are maximal at the point where the cerebral hemispheres rotate on the relatively fixed upper brainstem, the explanation of concussion.
In its fullest form, the characteristic clinical signs of concussive brain injury are the immediate abolition of consciousness, suppression of supportive reflexes (falling to the ground if standing), transient arrest of respiration, a brief period of bradycardia, and fall in blood pressure following a momentary rise at the time of impact. Rarely, if these abnormalities are sufficiently intense, death may occur at the moment of impact, presumably from respiratory arrest. In its mildest form, there is no apparent loss of consciousness or collapse, only a brief period of stunned disorientation, staggering, and subsequent amnesia during which the individual appears outwardly normal. The vital signs usually return to normal and stabilize within a few seconds even if the patient remains unconscious.
Brief tonic extension of the limbs, clonic convulsive movements lasting up to approximately 20 s, and other peculiar movements may occur immediately after the loss of consciousness (see McCrory et al). These “concussive convulsions” are probably of little prognostic significance and have not been shown to confer an increased risk of later seizures. McCrory and colleagues noted an association between motor and convulsive movements and facial impact, and we have seen this feature several times in teenagers who collided while pursuing a ball.
In the period during which the patient is unconscious and for a few moments afterwards, the plantar reflexes are extensor. After a variable period of time, the patient begins to stir and opens his eyes. Corneal, pharyngeal, and cutaneous reflexes, originally depressed, return, and the limbs withdraw from painful stimuli. Gradually, contact is made with the environment and the patient begins to obey simple commands and respond slowly to questions. Memories are not formed during this period; the patient may even carry on a conversation, which he cannot later recall. Finally, there is ostensibly full neurologic recovery corresponding to the time when the patient can form consecutive memories of current experiences.
The time required for the patient to pass through these stages of recovery may be only a few seconds or minutes, several hours, or possibly a limited number of days; but again, between these extremes there seem to be only quantitative differences. To the observer, such patients are comatose only from the moment of injury until they open their eyes and begin to speak; however, for the patient, the period of unconsciousness in one limited perspective extends from a point before the injury occurred (retrograde amnesia) until the time when he is able to form consecutive memories at the end of the period of anterograde amnesia. The duration of the amnesic period, particularly of anterograde amnesia, is but one index of the severity of the concussive injury. Although momentary “stunning” without loss of consciousness represents the mildest degree of concussion, it is not known if it shares the same mechanism as overt loss of consciousness. The aftereffects of concussion in causing anxiety, sleep disturbance, mental fogginess and cognitive difficulty, and dizziness are common to both and are discussed further on.
This is a topic of current interest and various guidelines regarding return to play have been published. A recent summary from the American Academy of Neurology can be consulted (authored by Giza and colleagues). The later-life development of dementia and other neurodegenerative conditions in professional athletes is discussed further on. Many useful observations have emerged from study of athletes after head injury. Foremost among these observations is that athletes who have had concussion are more likely than other players to have another concussion in the same playing season (Guskiewicz et al); whether this is a reflection of constitutional incoordination or the person’s style of play, or another factor is not known. Second, most prospective studies show a decline in reaction time and in other neuropsychologic tests after concussion, which returns to baseline over several days or weeks. Third, there is an indication from several series of concussions in National Collegiate Athletic Association and National Football League players that the number of recollected concussions is proportional to the degree of impairment on neuropsychologic tests (McCrea et al). Similar results have been found in other pursuits such as jockeying (Wall et al), but there are few adequate prospective studies.
The appropriate duration of removal from play has been the subject of numerous and largely arbitrary systems. The basis of most rules has been an appropriate conservatism that requires the absence of cerebral symptoms both at rest and under physical stress testing such as running or repetitive squatting. The duration of loss of consciousness and of amnesia was formerly a major component of the decision about return to play. More current guidelines focus instead on slowness in answering questions, uncertainty about plays or game assignments, and clumsiness, with or without loss of consciousness or amnesia. All such players are removed from the game. After medical evaluation, which may include imaging and neuropsychologic testing, a program of physical and cognitive “rest” is followed by graduated physical and mental activity under observation and a return to a lower level if symptoms occur (McCrory et al). Specifically, light aerobic exercise is followed by sport-specific training and noncontact, then contact, drills.
In contrast to concussion, in cases of traumatic brain injury that are fatal or very serious, the brain is usually bruised (contused), swollen, or lacerated, and there are hemorrhages, both meningeal and intracerebral, as well as hypoxic-ischemic lesions. A majority of patients who remain in a coma for more than 24 h after a head injury are found to have intracerebral hematomas and contusions. Of these lesions, the most frequent are contusions of the surface of the brain beneath the point of impact (coup lesion) and the sometimes more extensive lacerations and contusions on the side opposite the site of impact (contrecoup lesion), as shown in Fig. 35-4. Blows to the front of the head may produce mainly coup lesions, whereas blows to the back of the head may cause mainly contrecoup lesions. Blows to the side of the head produce either coup or contrecoup lesions, or both. Irrespective of the site of the impact, the common sites of cerebral contusions are in the frontal and temporal lobes, as illustrated in Figs. 35-4 and 35-5. The inertia of the malleable brain—which causes it to be flung against the side of the skull that is struck, to be pulled away from the contralateral side, and to be impelled against bony promontories within the cranial cavity, explains these coup–contrecoup patterns. Relative sparing of the occipital lobes in coup–contrecoup injury has been explained by the smooth inner surface of the occipital bones and subadjacent tentorium, as pointed out by Courville.
Figure 35-4.
Mechanisms of cerebral contusion. Arrows indicate point of application and direction of force; dark red areas indicate location of contusion. A. Frontotemporal contusion consequent to frontal injury. B. Frontotemporal contusion following occipital injury. C. Contusion of temporal lobe because of contralateral injury. D. Frontotemporal contusion as a result of injury to opposite temporooccipital region. E. Diffuse mesial temporooccipital contusion caused by a blow on the vertex. (From Courville.)
Figure 35-5.
Distribution of contusions emphasizing the frontal and frontotemporal distribution in 40 consecutive autopsy cases collected by Courville. (From Courville.)
The contused cortex is diffusely swollen and hemorrhagic, most of the blood being found around parenchymal vessels. On CT scanning, the lesions appear as edematous regions of cortex and subcortical white matter admixed with areas of increased density representing leaked blood (Fig. 35-6). The bleeding points may coalesce and give the appearance of a unitary clot in the cortex and immediately adjacent white matter. The predilection of these lesions for the crowns of convolutions attests to their traumatic origin (being thrown against the overlying skull) and distinguishes them from cerebrovascular and other types of cerebral lesions. There may be ball hemorrhages within the hemispheres that are independent of contusions as discussed below. Not surprisingly, such deep areas of bleeding are common in patients receiving anticoagulant or antiplatelet medications.
Of equal importance are axonal lesions that occur at the time of impact or evolve soon afterwards. Strich (1961) described the neuropathologic findings in patients who died months after severe closed head injuries that had caused immediate and protracted coma. In all of her cases, in which there were no signs of skull fracture, raised intracranial pressure, or gross subarachnoid hemorrhage, she observed an uneven but diffuse degeneration of the cerebral white matter that has become the basis of all subsequent work on diffuse axonal shearing (diffuse axonalinjury, DAI). In cases of shorter survival (up to 6 weeks), she observed ballooning and interruption of axis cylinders. These findings were subsequently confirmed and expanded by Nevin, by Adams and colleagues (1982), and by Gennarelli and coworkers, the last of these groups also working with monkeys.
The extension of Strich’s concept, that postulates diffuse axonal injury throughout the cerebral white matter as the main cause of persistent unconsciousness, has been widely adopted. In relation to concussion, shearing lesions are also seen in the midbrain and lower thalamus in severe injury, providing some evidence for this neuronal dysfunction in these regions as the cause of concussion.
In most cases of severe head injury, there is damage to the corpus callosum by impact with the falx; necrosis and hemorrhage are sometimes visible by CT and can be seen to spread bilaterally to adjacent white matter (Fig. 35-7). There may also be scattered hemorrhages in the white matter along lines of force from the point of impact to the contralateral side. The degeneration of white matter from diffuse axonal injury can be remarkably diffuse, with no apparent relationship to focal destructive lesions, although differentiating it from secondary wallerian change that originates in a surface or callosal contusion can be difficult. Investigations using MRI, such as the series by Kampfl and colleagues, suggest that diffuse axonal injury may be the substrate of the persistent vegetative state. However, in most cases of severe cranial injury and protracted coma, there have been major sites of injury in the midbrain and subthalamus, i.e., in the zones subjected to the greatest torque, and these latter lesions may be the critical ones in persistent coma and vegetative state (Adams et al 2000, Ropper and Miller). This was true of the cases of persistent coma described by Jellinger and Seitelberger. Notable, again, was that these deep lesions coincided with the postulated locus of reversible concussive paralysis.
Primary brainstem hemorrhages due to torsion and tearing of tissue at the time of impact are distinguished from the secondary hemorrhages that are a result of the effects of downward displacement of the brainstem. Duret originally emphasized the medullary location of these secondary hemorrhages, but the term Duret hemorrhage has come to signify all brainstem hemorrhages when there is mass effect that distorts the brainstem.
In addition to contusions and extradural, subdural, subarachnoid, and intracerebral hemorrhages, closed head injury induces variable degrees of vasogenic edema that increases during the first 24 to 48 h and sometimes, small zones of infarction that have been attributed to vascular spasm caused by subarachnoid blood surrounding basal vessels. The frequency and importance of this type of secondary cerebral infarction have been debated. A retrospective imaging study by Marino and colleagues found that 17 of 89 patients had regions of stroke after moderate or severe head injury. Most were in the distribution of a major branch or penetrating cerebral vessel or in a watershed territory.
The presence of intracranial hypertension has also been associated with a higher incidence of infarction. Marmarou and colleagues demonstrated that brain swelling after head injury is essentially the result of edema and not of an increase in cerebral blood volume, as has long been postulated. In children, and in some cases in adults, the cerebral edema may be massive and lead to secondary brainstem compression.
With fractures of large bones in cases of generalized trauma, with or without head injury, after 24 to 72 h there may be an acute onset of pulmonary symptoms (dyspnea and hyperpnea) followed by coma with or without focal signs or seizures. This sequence is a result of systemic fat embolism, first of the lungs and then of the brain. Cranial trauma is not required. In some cases the onset of pulmonary symptoms is associated with a petechial rash over the thorax, especially in the axillae and also in the conjunctivea and 1 in 3 cases is said to show fat globules in the urine. The specificity of the last of these findings has been questioned. Respiratory distress is the most important and often the only feature of the fat embolism syndrome, evident in the chest film as fluffy infiltrates in both lungs. In the brain, multiple small fat emboli cause widespread petechial hemorrhages and small infarctions, involving both white and gray matter and a few larger infarcts. Most patients with fat embolism recover spontaneously in 3 or 4 days, although a mortality rate of up to 10 percent is cited, usually related to underlying systemic and bony injuries. Treatment, aside from respiratory support, is supportive. Heparin, which had been used in the past, is not considered effective.
Approach to the Patient with Head Injury
The physician first called on to examine a patient who has had a closed head injury will generally find one of three clinical conditions as indicated by the headings below, each of which is dealt with differently. It is usually possible to categorize the patient by assessing the mental and neurologic status when first seen and at intervals after the accident. The Glasgow Coma Scale is used as a rapid reference to accomplish this purpose (Table 35-1) but does not substitute for a fuller neurologic examination. The scale registers three aspects of neurologic function: eye opening, verbal response, and motor response to various stimuli. The scale uses a summed score with a maximum of 15; a score of 7 or less is considered to reflect severe trauma and a poor clinical state, 8 to 12, moderate injury, and higher scores, mild injury. The scores provided by this scale correspond roughly with the outcome of the head injury as discussed further on, but its main utility is in recording sequential changes in the patient’s clinical state with an easily learned and reproducible tool.
EYES OPEN | |
Never | 1 |
To pain | 2 |
To verbal stimuli | 3 |
Spontaneously | 4 |
BEST VERBAL RESPONSE | |
No response | 1 |
Incomprehensible sounds | 2 |
Inappropriate words | 3 |
Disoriented and converses | 4 |
Oriented and converses | 5 |
BEST MOTOR RESPONSE | |
No response | 1 |
Extension (decerebrate rigidity) | 2 |
Flexion abnormal (decorticate rigidity) | 3 |
Flexion withdrawal | 4 |
Localizes pain | 5 |
Obeys | 6 |
Total | 3–15 |
This is the most frequently encountered clinical situation. Roughly, two degrees of disturbed function can be recognized within this category. In one, the patient was not unconscious at all but only stunned momentarily, “saw stars,” or was briefly disoriented. This injury is insignificant when judged in terms of life or death and brain damage, although, as we point out further on, there is still the small possibility of a skull fracture or the later development of an epidural or subdural hematoma. Moreover, some patients are liable to a troublesome posttraumatic syndrome consisting of headache, giddiness, fatigability, insomnia, and nervousness that can appear soon after or within a few days of the injury. This problem is discussed in a later section. In the instance of consciousness that was temporarily abolished for a few seconds or minutes, recovery may already be complete, or the patient may be in one of the stages of partial recovery described earlier. Even though mentally clear, there is amnesia for events immediately preceding and following the injury. The latter produces a circumscribed confusional state that is usually confined to inattention and may be ongoing when the patient is first examined. It is characterized by a dazed appearance and repetitive questions from the patient about the circumstances that led to his being found.
In most such mild cases, a brief assessment for mental clarity, weakness, ocular abnormalities, and Babinski signs is appropriate, but there is little need of extensive neurologic consultation and hospitalization is not required, provided that a responsible family member is available to report any change in the clinical state. In only a small group of these patients, mainly in those who are slow in regaining consciousness or who have severe headache, vomiting, or a skull fracture, is there significant risk of intracerebral hemorrhage or other delayed complications.
Whether to obtain imaging of the head routinely in such patients is an unresolved problem. In our litigious society, the physician is inclined to obtain a CT scan. If there is no subarachnoid blood (a common finding) or intraparenchymal clot or contusion, and the patient is mentally clear there is little chance of developing an extradural hemorrhage. The presence of a fracture may increase these odds but most studies, such as the one by Lloyd and colleagues have found that the presence of a skull fracture in children proves to be a relatively poor indicator of intracranial injury. The exception is a fracture through the squamous bone and the groove of the middle meningeal artery, which represents a risk for arterial bleeding and epidural hemorrhage.
With the current focus on the cost-effective use of ancillary studies, criteria that justify obtaining a cranial CT following minor forms of head trauma have been developed. We have generally advised a CT in cases of head injury that was associated with prolonged loss of consciousness (more than 1 min), severe and persisting headache, nausea and vomiting, a confusional state, and any new, objective neurologic signs, but these are admittedly arbitrary criteria. The CT scan may be particularly important in elderly patients with minor head trauma, in whom the presence of an intracranial lesion (mainly subdural hematoma) may not be predicted by clinical signs. However, in children, it may be advisable to perform the scans more liberally. This is underscored by the results of a study of 215 children with minor head trauma conducted by Simon and colleagues: 34 children with no known loss of consciousness and a Glasgow Coma Scale score of 15 nonetheless displayed intracranial lesions, 3 of whom required surgery.
Several studies in adults have given broad guidance in choosing which patients to scan (“New Orleans Criteria” and “Canadian CT Head Rule”; Table 35-2). They include features that are sensitive but not specific for intracranial injury, such as age above 60 years, intoxication, more than 30 min of retrograde amnesia, suspected skull fracture, seizure, anticoagulation, and dangerous mechanism of injury, (see Smits et al and Stiell et al). These two validated schemes for assisting in the determination of need for CT scanning in the emergency department are included for the reader’s reference but they should be viewed as broad guidelines with fairly high sensitivity for important lesions on the CT, but low specificity so that most CT scans done under these advisories can be expected to be normal. These issues are addressed in a review by Ropper and Gorson.
New Orleans Criteriaa—Glasgow Coma Scale score of 15 |
Headache |
Vomiting |
Age >60 y |
Drug or alcohol intoxication |
Persistent anterograde amnesia (deficits in short-term memory) |
Evidence of traumatic soft-tissue or bone injury above clavicles |
Seizure |
Canadian CT Head Ruleb—Glasgow Coma Scale score of 13–15 for patients age 16 y and older |
High risk of neurosurgical intervention |
Glasgow Coma Scale score <15 within 2 h after surgery |
Suspected open or depressed skull fracture |
Any sign of basal skull fracture |
Two or more episodes of vomiting |
Age >54 y |
Moderate risk of brain injury detected by CT |
Retrograde amnesia for ≥30 min |
Dangerous mechanism |
Minor and seemingly trivial head injuries may sometimes be followed by a number of puzzling and worrisome clinical phenomena, some insignificant, others serious and indicative of a pathologic process other than concussion. The latter are described below. When they occur, a neurologic or neurosurgical evaluation is indicated.
These symptoms occur most often in children, who, minutes or hours after a concussive head injury, seem not to be themselves. They lie down, are drowsy, complain of headache, and may vomit—symptoms that suggest the presence of an intracranial hemorrhage. Mild focal edema near the point of impact may be seen on MRI. There is usually no skull fracture but, as Nee and colleagues point out, vomiting is associated with an increase in the incidence of skull fracture and the New Orleans and Canadian CT rules found vomiting to be a factor associated with intracranial bleeding (Table 35-2). The symptoms subside after a few hours, attesting to the benign nature of the condition in most cases but some form of cerebral imaging is required.
With falls or blows on top of the head, both legs may become temporarily weak and numb, with wavering bilateral Babinski signs and sometimes with sphincteric incontinence. Impact over the occiput may cause temporary blindness. The symptoms disappear after a few hours. It seems possible that these transient symptoms represent a direct localized concussive effect, caused either by indentation of the skull or by impact on these parts of the brain against the inner table of the skull, but a vascular mechanism cannot be excluded. The blindness and paraplegia are usually followed by a throbbing, vascular type of headache. Transient migrainous visual phenomena, aphasia, or hemiparesis, followed by a headache, are observed sometimes after minimal concussion in athletes who participate in competitive contact sports. Possibly all of these phenomena are the result of an attack of migraine induced by a blow to the head. These focal syndromes can be perplexing for a few hours, especially if it is the first such attack of migraine in a child. Possibilities to be remembered, particularly in cases of acute quadriplegia, is traumatic cord compression or the rarer cartilaginous embolism of the cervical cord (see “Fibrocartilaginous Embolism” in Chap. 44). A concussion of the cervical portion of the spinal cord is another potential mechanism of transient paraplegia.
Episodes of transient global amnesia after minor head injury have been described by Haas and Ross, as mentioned in Chap. 21. The problem in interpreting these spells is their similarity to posttraumatic amnesia and the repetitive stereotyped questioning regarding orientation that is common to both processes. A duration of 2 to 24 h and the feature of repetitive querying were suggested by Haas and Ross as differentiating the two conditions but the separation on this basis is not compelling.
The main causes of delayed hemiplegia are a late-evolving epidural or subdural hematoma and, in more severe injuries, an intracerebral hemorrhage. Most of these are associated with a diminution in the level of consciousness from the outset but there are exceptions.
Dissection of the internal carotid artery should always be considered in cases of delayed hemiplegia. The dissection may occur in the extracranial or the intracranial portion of the carotid artery and should be sought by vascular imaging study if the hemiparesis has no other explanation. In other instances, the hemiplegia has no clear explanation other than the blow to the head, perhaps related to the migraine phenomenon described earlier.
This group is smaller than the other two but is of importance because it includes a disproportionate number of patients who are in urgent need of surgical treatment. The initial loss of consciousness from concussion may have lasted only a few minutes or, exceptionally, there may have been no period of unresponsiveness at all, in which instance one might wrongly conclude that there was no concussion and little possibility of traumatic hemorrhage or other type of brain injury. Patients who display this sequence of events, in the past referred to vividly as “talk and die” by Marshall and associates (1983), have late deterioration because of the expansion of a subdural hematoma, worsening brain edema around a contusion, or the delayed appearance of an epidural clot. Among 34 such patients in the Traumatic Coma Data Bank who had this type of lucid interval, the majority showed substantial degrees of midline shift on the initial CT scan, reflecting the presence of early brain edema and contusion (Marshall et al, 1983). A somewhat related condition of delayed intracerebral hematoma (spät apoplexie), discussed further on, is a feature of a more severe initial head injury that usually produces coma from the onset. The problem of cerebral fat embolism, mentioned earlier, should be considered in these cases of delayed deterioration, especially if there is interposed respiratory failure.
Here, the central problem, set forth by Symonds, is the relationship between concussion and contusion and other forms of persisting structural brain damage. Because consciousness is abolished at the moment of injury, one can hardly doubt the existence of concussion in such cases; but when hours pass without consciousness being regained, the second half of the usual definition of concussion—that the disruption of cerebral function be transitory—is not satisfied. Pathologic examination of such cases usually discloses evidence of increased intracranial pressure and of cerebral contusions, subarachnoid hemorrhage, zones of infarction, and scattered intracerebral hemorrhages both at the point of injury (coup) and on the opposite side (contrecoup), in the corpus callosum, and between these points, along the line of force of the impact. In some patients, the diffuse axonal type of injury is prominent or, as mentioned, there are separate but strategically placed ischemic and hemorrhagic lesions in the upper midbrain and lower thalamic region. Varying amounts of blood in the subarachnoid and subdural spaces are present. Displacement of the thalamus and midbrain may be present, with compression of the opposite cerebral peduncle against the free margin of the tentorium as well as secondary midbrain hemorrhages and zones of necrosis; in some cases, there is transtentorial herniation.
Severe head injury is often associated with an immediate arrest of respiration and sometimes with bradyarrhythmia and cardiac arrest. The immediate effects on the brain of these systemic changes may in themselves be sufficiently profound to cause coma. Intracranial pressure is almost always elevated and imaging of the brain shows various degrees of brain swelling, ventricular compression, and displacement of midline structure. Also, head injury often complicates alcohol and drug ingestion, so the possibility of a toxic or metabolic encephalopathy as the cause (or a contributing cause) of stupor must always be considered.
In all of these patients, following the initial period of stabilization, the matter of interest is the clinical and imaging assessment, with the purpose of uncovering a surgically remediable lesion, namely a subdural or epidural hematoma or a treatable intraparenchymal hematoma. In most cases, the discovery of such a mass lesion leads to surgical removal. But unless it is the only lesion, the procedure often proves to be insufficient and coma is likely to persist because of the associated cerebral damage. The recognition and management of these hematomas are described further on.
In the Traumatic Coma Data Bank, which included 1,030 gravely injured patients with Glasgow Coma Scale scores of 8 or less, 21 percent had subdural hematomas, 11 percent had intracerebral clots, and 5 percent had epidural hematomas. Notable, however, half the patients had no mass lesions on the CT scan. On this basis, these patients were thought to have diffuse axonal injury. However, in 50 consecutive autopsies of severely injured patients, summarized in an earlier era by Rowbotham
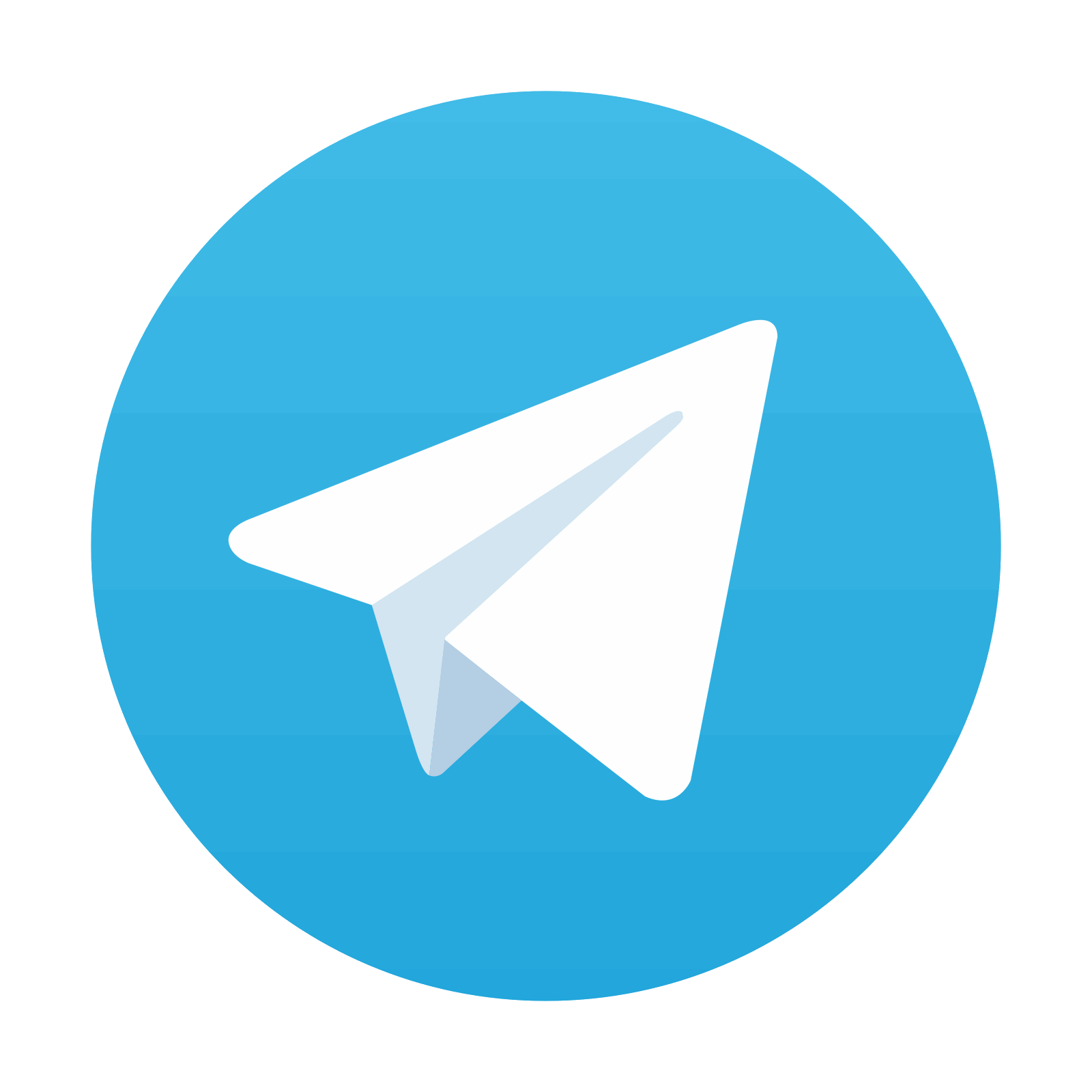
Stay updated, free articles. Join our Telegram channel

Full access? Get Clinical Tree
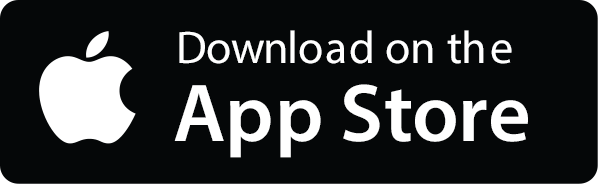
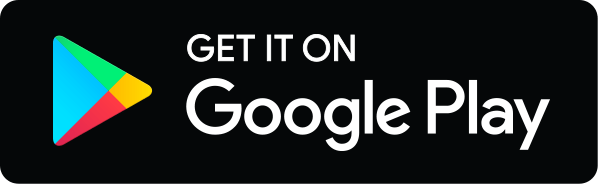
