Chapter 146 Chronobiologic Monitoring Techniques
Basic Concepts and Terminology
As simple and straightforward as it would seem to be to measure the period length of the human circadian rhythm, the field of circadian rhythms research has undergone continual upheaval as methodologic errors have been repeatedly revealed and new paradigms designed to correct them. In humans, for example, there have been continued corrections of the period length of the free-running circadian rhythm length, or period, which is now estimated at slightly greater than 24 hours, 24.18 hours being the most recent value.1
Methodologic Questions in the Field of Circadian Rhythms
During the preceding 5 decades, various estimates have placed the human circadian period length anywhere from 13 to 65 hours.2 Also, greater interindividual inconstancy in circadian variables in humans had been observed than that observed in other mammals. These findings showed a disparity between humans and nonhuman mammals, in which circadian rhythms are remarkably stable from day to day. In humans, there have been continued corrections of the period length of the circadian rhythm of activity, which has been most recently estimated at slightly greater than 24 hours, with a precision not that different from our mammalian relatives.
Disagreements continue over which stimuli are capable of acting as zeitgebers to entrain or shift circadian rhythms. Initially, only bright light was considered to be capable of shifting the timing of circadian rhythms.3 Subsequently, light of moderate intensity was described as being capable of shifting circadian rhythms,4 and, most recently, studies now maintain that ordinary room light is capable of inducing a circadian shift.5,6 Some authors have claimed that extraocular light is capable of influencing circadian rhythms. One study demonstrated that behind-the-knee (popliteal region) bright light is capable of inducing shifts in circadian timing,7 but there have been multiple failures to replicate this finding.8–11 As chronobiology research progressed, a series of modifications of experimental design successively eliminated some masking artifacts and external zeitgebers that previously introduced artifact.1
The field has also been inundated by controversy over what constitutes a zeitgeber, or a stimulus capable of entraining a circadian rhythm. There is evidence that nonphotic stimuli are capable of entraining individuals.12 Exercise,13,14 social activity,15 feeding schedule,16 ambient temperature,17 napping in darkness,18 administration of melatonin,19 and knowledge of time of day or night20 each has the capacity to entrain human and nonhuman subjects. Some studies suggest a major role for such nonvisual zeitgebers,12 whereas other studies imply a more modest role.21 The extent to which melatonin is capable of shifting circadian rhythms was controversial until recently.22
Another area of research involves separating behavioral and biological properties of circadian rhythms from other physiologic processes. Many behavioral and biological phenomena are influenced by the sleep-wake cycle, or the duration of prior wakefulness. This is known as the homeostatic model, which describes an increasing pressure to sleep with increased duration of wakefulness and a decreased pressure to sleep with increased duration of sleep.23 Circadian rhythm studies must separate variance in a biological or behavioral parameter into homeostatic and circadian components; that is, they must identify what portion of the parameter’s variance is circadian and what portion is homeostatic.24,25 Neither the circadian nor the homeostatic models can explain in a compelling manner why humans are most wide awake in the hour or two preceding sleep and sleepiest in the hour after morning awakening.
An additional area of continued controversy is what biological metric is best used to approximate the properties of the underlying core pacemaker, such as period length. Core body temperature24 and melatonin26 secretory profiles have been used in most studies. Some research uses dim-light melatonin onset as the marker of the beginning of a new circadian cycle, others use dim-light melatonin offset,26 and many use the temperature nadir. However, there is no obvious point that can be identified as the minimum of temperature or the onset of melatonin secretion. Temperature fluctuates from moment to moment, and the minimum or maximum must be extracted mathematically. Melatonin rises gradually after sunset, and an arbitrary level must be selected to denote onset or offset. In addition, it must be emphasized that extracting circadian rhythm data relies on mathematical criteria and data analytic techniques selected in each experimental protocol.1
Paradigms
Fixed Light-Dark Schedules, Double Plotted
The DD paradigm has been successful in demonstrating the remarkable consistency of a given species’ free-running activity rhythm in constant darkness or dim light, successfully elucidating the period length of its biological clock.1 Often, the timing of light and dark are abruptly changed to measure the animal’s reaction, which can take several days to adapt. Such paradigms are at the core of much genetic research, in which normal (wild-type) animals (+/+) are compared with heterozygous (+/−, −/+) or homozygous (−/−) knockouts. Studies reveal genetic phenotypes (behavioral expression) because knockout animals display altered periodicity, diminished rhythmicity, or lack of any circadian rhythm in running, feeding, or drinking behavior.
Phase-Shifting Protocols
Many studies have examined the capacity of light of varying intensities and durations to alter the timing of sleep, melatonin secretion, or the temperature nadir. These studies share in common a baseline in which environmental light is controlled but typically is similar to the timing of natural environmental light. Often the baseline consists of dim light (ranging from about 70 lux, equivalent to romantic restaurant lighting, to as low as 1.5 lux, similar to the light from a candle) in different studies. In the experimental condition, light is then delivered to the subject in what would normally be the dark or night portion of the circadian rhythm.27 Light is delivered for the same duration but at a different time during this experimental condition for 1 or several days. In some studies there is then a subsequent condition in which the subject is in constant dim light or in a constant routine (described later).
Circadian variables such as wheel running in rodents and hamsters, the timing of sleep, or the timing of melatonin secretion are monitored during each condition.1–3 The magnitude of the change in a circadian variable after the altered timing of light is measured. A phase response curve (see Fig. 146-1) shows the magnitude of response to light exposure (typically 1 to 3 hours) at different times throughout the circadian cycle in changing the timing of a circadian output variable.28 Most studies show circadian rhythm shifts in response to light occurring only if the light is delivered at specific times, typically at or near normal hours of darkness, and indicate that mammals are not responsive to bright light administered during normal hours of daylight.29 Other studies claim there is no dead zone and that bright light delivered at any hour, including normal daylight hours, has a phase-shifting effect.30
Time-Isolation Protocols
One of the more unexpected findings in circadian rhythm research is that the subjective sense of sleepiness, or the wish to sleep, is at its minimum at the normal time of sleep initiation and that sleepiness is greatest at the normal time of awakening.31–35 This propensity to be alert at the normal hour of onset of sleep has bedeviled studies attempting to assess the period length of the human circadian clock using time-isolation protocols. Under conditions of time isolation, a variety of studies showed great variability in human sleep-wake cycle length, unlike studies in any other mammal. Studies showed sleep-wake cycles as long as 36 hours and great day-to-day variability in both sleep and wake duration.36 Subjects who remained in time-isolation conditions for a sufficient interval developed desynchrony between their temperature rhythm and their sleep-wake cycle. Under normal conditions, the temperature minimum occurs somewhat after the middle of the sleep period. In subjects in temporal isolation protocols, the interval from one temperature minimum to the next has a cycle length of 24 to 25 hours, but the interval between sleep initiation on successive nights would be longer, meaning that the temperature minimum can stray elsewhere in the sleep-wake schedule.37
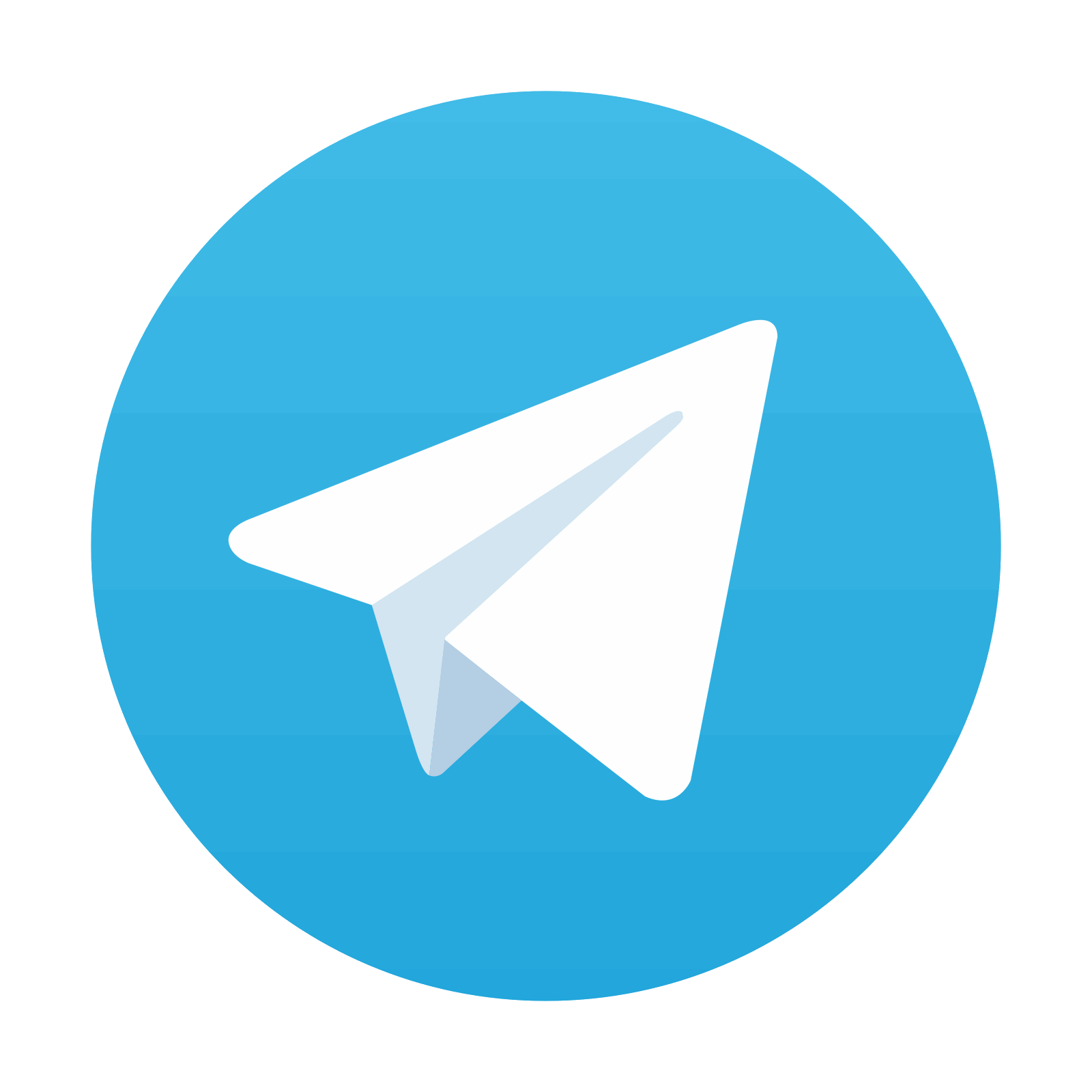
Stay updated, free articles. Join our Telegram channel

Full access? Get Clinical Tree
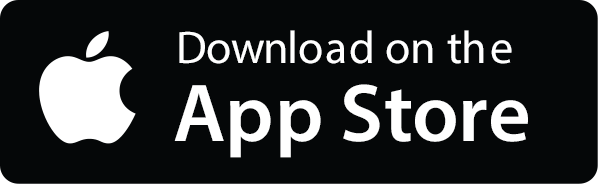
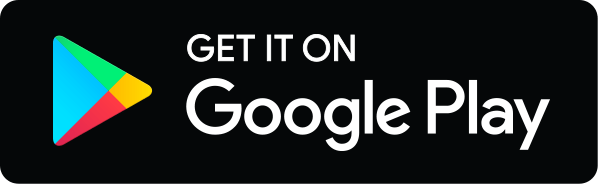