Chapter 38 Circadian Rhythms in Sleepiness, Alertness, and Performance
Circadian Rhythms
Subjective Measures of Sleepiness and Alertness
Before discussing circadian rhythmicity in waking functions, it is important to define terms relating to waking function. In this chapter, neurobehavioral functions include sleepiness, behavioral alertness, and performance. Sleepiness refers to subjective reports of the desire to sleep or of efforts to resist sleep. Behavioral alertness refers to the degree to which an organism can quickly and reliably use selective and sustained attention. Performance refers to cognitive functions ranging in complexity from psychomotor vigilance and working memory to logical reasoning and decision making. The interaction of the circadian and sleep–wake systems in regulating sleepiness and performance is described in the second part of this chapter. Sleep propensity1,2 is not covered in this chapter, because our focus is on effortful waking cognitive performance and its associated subjective states.
Many different subjective measures of sleepiness and alertness can assess circadian rhythmicity in neurobehavioral variables, as long as the scale requests ratings on the immediate state of the subject. These include visual analogue scales (VAS)3; Likert-type rating scales such as the Stanford Sleepiness Scale (SSS)4 and the Karolinska Sleepiness Scale (KSS)5; and adjective checklists such as the Activation-Deactivation Adjective Check List (AD-ACL)6 and Profile of Mood States (POMS).7 Despite structural differences among these scales, all self-reports of sleepiness tend to be highly intercorrelated and because they are relative psychometrics, they are subject to a number of sources of variance. For example, as shown in Figure 38-1, the effects of cognitive performance on subjective alertness are evident only when sleep loss has commenced and this effect is modulated by circadian variation.
Objective Measures of Cognitive Performance
Many studies of circadian rhythms rely on objective performance measures to track the temporal dynamics of endogenous circadian rhythmicity. Classic examples of such measures include search-and-detection tasks8 and simple and choice reaction time tasks,9 sorting,10 logical reasoning,11 memory access,12 and real-world tasks such as meter reading accuracy13 and school performance.14 Typically, response speed and accuracy to a series of repetitive stimuli are analyzed, although the sensitivity of the performance metric used to track circadian variation will depend heavily on whether the task is work-paced versus subject-paced, on speed versus accuracy tradeoffs in performance metrics,15 on the rate and number of responses acquired during the task, on whether the task metrics reflect performance variability or mean performance, and on the overall technical precision of the measurement. Even short-duration, work-paced tasks that precisely measure variability in performance can be used to demonstrate circadian variation.16
Earlier studies have concluded that different tasks17,18 and different task outcomes19,20 may yield distinct peak phases of circadian rhythmicity, suggesting that many distinct circadian rhythms using different clock mechanisms exist.21,22 This hypothesis, however, has not been substantiated to a reasonable degree of scientific certainty. Under strictly controlled laboratory conditions, most intertask differences in circadian variation disappear.23,24 As illustrated in Figure 38-2, under controlled sleep deprivation conditions, the circadian rhythms of neurobehavioral performance variables co-vary with each other and with subjective sleepiness. Importantly, these rhythms mimic the circadian profile of core body temperature, one conventional marker of the biological clock.25,26 Under entrained conditions, higher and lower core body temperature values typically correspond to good and poor performance, respectively.24,27,28
Masking and Other Influences
Subjective measures of sleepiness and alertness are vulnerable to numerous confounding influences that can “mask” their circadian rhythmicity. Masking refers to the evoked effects of noncircadian factors on measurements of circadian rhythmicity. The context in which such measurements are taken (i.e., the environmental and experimental conditions) is a major source of masking effects. Masking can alter or obscure a circadian rhythm, or it can create the appearance of a circadian rhythm. Masking factors specifically affecting sleepiness and alertness include: demand characteristics of the experiment,29 distractions by environmental stimuli and noise,30 boredom and motivational factors,31–33 stress,34 food intake,35,36 posture and activity,37,38 ambient temperature,32 lighting conditions,39,40 and stimulant drug intake (e.g., caffeine, modafinil, amphetamine).41–43
Physical, mental, and social activities can represent masking factors that interact with endogenous circadian rhythms in neurobehavioral functions. This is illustrated in Figure 38-1: The effects of performing cognitive tests on subjective estimates of alertness are apparent at certain circadian phases during sleep deprivation. Subjects report feeling less alert after they are challenged to perform. Thus, prior activity can influence subjective estimates and can interact with circadian effects if not properly controlled when measuring the rhythmicity of subjective states.
Direct Effects on the Biological Clock
Many stimuli also affect the biological clock itself by phase-shifting other circadian rhythms such as melatonin and body temperature, and therefore can affect circadian rhythmicity of neurobehavioral measures. Such stimuli—which have varying effects on the body depending upon their magnitude and the time of administration—include light, exercise, exogenous melatonin, triazolam administration, food intake, social cues, and auditory stimuli (reviewed in Goel44,45). As such, it is important to also minimize or control potential zeitgebers (i.e., time givers) in laboratory studies designed to measure neurobehavioral performance.
The Midafternoon Dip
Some individuals display a short-term afternoon dip in the circadian profiles of neurobehavioral variables and core body temperature, which is called the midafternoon, siesta, postlunch, or postprandial dip. This dip has been observed in field studies (e.g., see Bjerner et al.13) and in controlled laboratory experiments (e.g., see Monk et al.46) independent of food intake, although it has not been found consistently.47,48
Field studies of human performance support the existence of a midafternoon dip,49 but such data cannot be considered definitive because of the uncontrolled influence of differential amounts of activity by individuals over time (i.e., exposure). The most compelling evidence for the existence of a midafternoon dip derives from studies on sleep propensity50,51 and from the natural timing of daytime naps.52 Nevertheless, because of the inconsistent observation of the midafternoon dip in performance and sleepiness, the phenomenon remains poorly understood, and its relationship to the biological clock remains unclear.
The Practice Effect and Other Artifacts
The well-known practice effect that occurs when cognitive tasks are performed repeatedly can limit (mask) the ability to detect circadian rhythmicity in these tasks. This is illustrated in Figure 38-3, which shows performance on a standard serial addition-subtraction task improving across three consecutive days. The practice effect can be difficult to distinguish from the circadian rhythm of performance. This problem might possibly be circumvented by testing subjects in different orders across times of day—the practice effect is then balanced out by averaging over subjects. This approach assumes that the practice effect and the circadian performance rhythm are additive and have the same relationship in every subject. Because this assumption has remained untested, it is unclear whether practice effects on cognitive tests can be averaged out reliably. Thus, a better method for addressing this problem may be to train subjects to asymptotic performance levels before attempting to assess circadian rhythmicity in cognitive performance.
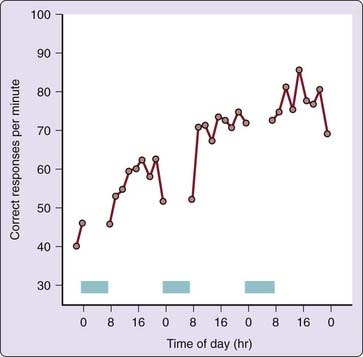
Figure 38-3 Practice effect in average cognitive throughput performance for 29 healthy adult subjects tested every 2 hr from 7:30 AM until 11:30 PM each day, during a 3-day period in which they were allowed up to 8 hr of sleep per night (blue bars). The performance task was the serial addition/subtraction test, which is part of the Walter Reed performance assessment battery.54a In this task, subjects were presented with a rapid sequence of two single digits (0 to 9) followed by an operator (plus or minus), and they were instructed to enter only the least-significant single digit of the algebraic sum, unless the result was negative, in which case 10 was to be added to the answer first. Note the substantial learning curve (i.e., practice effect) across days, resulting in a doubling of the mean correct responses within 25 trials. This effect dominates the performance profile, obscuring circadian changes within days. Practice effects “contaminate” nearly all tasks involving cognitive operations.
Practice effects are not the only masking factors that may hide, accentuate, or otherwise distort circadian rhythmicity in task performance. Many of the same variables that mask circadian rhythmicity in subjective estimates of sleepiness and alertness can also mask circadian variation in performance. The effects of masking vary from distortions of the range of circadian variation to changes in the shape of the circadian curve; even concealment of the circadian rhythm is possible (as shown in Fig. 38-3, in which sleep prevents measurement of nocturnal performance and the practice effect obscures circadian variation in diurnal performance). Thus, it is difficult to extract meaningful information about the amplitude (i.e., magnitude) and the phase (i.e., timing) of the circadian rhythm in performance measures without understanding the masking effects that influence these variables.
Not only is performance often affected by learning, aptitude, and other masking factors (e.g., lighting, background noise, demand characteristics, as listed previously), but it is also affected by subjects changing their performance strategy on a task.53 Thus, it can be difficult to distinguish the circadian rhythm in task performance from that of simultaneous changes in performance strategy, unless a task prevents the subject from adopting alternative performance strategies. The same difficulty applies to compensatory effort (that is, increased effort to keep up performance), the effects of which are particularly notable if subjects are informed about their results during a performance task (i.e., performance feedback).
Electroencephalographic and Ocular Measures
The circadian rhythm in cognitive performance reflects functional changes in the brain. Evoked potentials or event-related potentials (ERPs)—the brain potentials associated with reaction to a stimulus—have been used to measure alertness. Event-related potential measurements must be taken consecutively and frequently (i.e., many stimuli must be presented) to average out the background electroencephalogram (EEG). Therefore, ERPs are usually recorded during repetitive search-and-detection and reaction time tasks. Diurnal changes in the amplitude and the location of ERP waves have been interpreted as reflecting circadian variations in alertness.54b,55 Hemispheric differences have been detected,56 suggesting separate circadian rhythms for the left and right hemispheres. Interpretation of ERP data is difficult due to masking from a variety of sources (e.g., see Geisler and Polich57).
Circadian variations in alertness have also been associated with changes in the background EEG during wakefulness. The amount of theta and alpha activity (i.e., EEG activity in the frequency bands from 4 to 8 Hz and from 8 to 12 Hz, respectively) in the resting EEG with eyes remaining either open or closed (to avoid artifacts from blinking) have been related to alertness level.58–60 However, difficulties in the recording and analysis of waking EEG61,62 have limited its interpretability. Furthermore, significant effects in the EEG occur primarily when alertness is much lower than what is normally encountered at the trough of circadian variation—such as when subjects are sleep deprived.63
The EEG has also been used to measure the latency to fall asleep (as a measure of sleep propensity) at various times of day. These sleep latency tests include the Multiple Sleep Latency Test (MSLT)1 and the Maintenance of Wakefulness Test (MWT)49 as well as variants of these paradigms.
Slow eyelid closures, slow rolling eye movements (SEMs), and a number of other ocular variables relate to sleepiness.5,64–68 Pupil diameter relates to autonomic tone (i.e., pupils dilate with greater sympathetic dominance), and autonomic tone co-varies with sleep pressure.69 Pupillometry may therefore also yield estimates of sleepiness, but only if environmental light and other confounding influences on pupil diameter are strictly controlled. The various ocular measures of sleepiness have been investigated primarily under conditions of considerable sleep loss, when the observed effects are more substantial than those found across the circadian cycle. Whether ocular measures can be used reliably for detecting circadian fluctuations in sleepiness remains to be determined.
Interindividual Variability in Circadian Rhythms
Subjects show interindividual differences in the free-running circadian period (tau; τ).70 Subjects also demonstrate interindividual differences in circadian amplitude26,71 and circadian phase25,26,71 that appear to be due mostly to genetic influences.71 There are several standardized methods for assessing interindividual differences in circadian rhythms. One recently developed and accurate method using molecular techniques can determine individual differences in τ, amplitude and phase-resetting, which relate to diurnal phase preference, using cultured human fibroblasts from skin biopsies or blood samples.72–74 Standard physiological estimates of circadian phase include the dim light melatonin onset (DLMO)75 and core body temperature minimum.25,26 The aforementioned methods are important for characterizing interindividual variation in circadian rhythmicity.
Morningness-Eveningness
Morningness-eveningness (i.e., the tendency to be an early “lark” or a late “owl”) is perhaps the most frequently measured interindividual variation in circadian rhythmicity. Morning- and evening-type individuals differ endogenously in the circadian phase of their biological clocks.25,26 Self-report measures, such as the Horne-Östberg Morningness-Eveningness Questionnaire (MEQ)76 and its variants (e.g., see Smith et al.77), and more recently developed scales such as the Munich Chronotype Questionnaire,78,79 which differentiates timing of activities on workdays versus free days, are the most commonly used measures of circadian phase preference because of their convenience and cost effectiveness.
Age affects morningness-eveningness as shown in laboratory studies80 and more naturalistic population-based settings (reviewed in Roenneberg et al.79 and Foster and Roenneberg et al.81). In addition, gender has been reported to influence morningness and eveningness with women showing a greater skew toward morningness than men.79,82,83 This difference in circadian phase preference is an enduring trait, with a significant genetic basis.84–86 As such, chronotype is a phenotypic aspect of circadian rhythmicity in humans.87
The genetic basis of morningness-eveningness in the general population has been investigated by targeting several core circadian genes which have yielded mixed results.88 For example, the 3111C allele of the CLOCK gene 5′-UTR region has been associated with eveningness and delayed sleep timing in some studies89,90 but not others.91–93 Similarly, the variable number tandem repeat (VNTR) polymorphism in PERIOD3 (PER3), another core clock gene, has been linked to diurnal preference, but not uniformly.94–99 In addition, the 111G polymorphism in the 5′-untranslated region of PERIOD2 (PER2) has been associated with morning preference,100 as has the T2434C polymorphism of PERIOD1 (PER1).101 Because morningness-eveningness represents a continuum, it is likely this trait is polygenic (influenced by several genes), with each gene contributing to the determination of circadian phase preference. Thus, further studies investigating other clock genes, as well as replication of the PER and CLOCK findings, are needed to establish precisely the molecular genetic components of behavioral circadian phase preference.
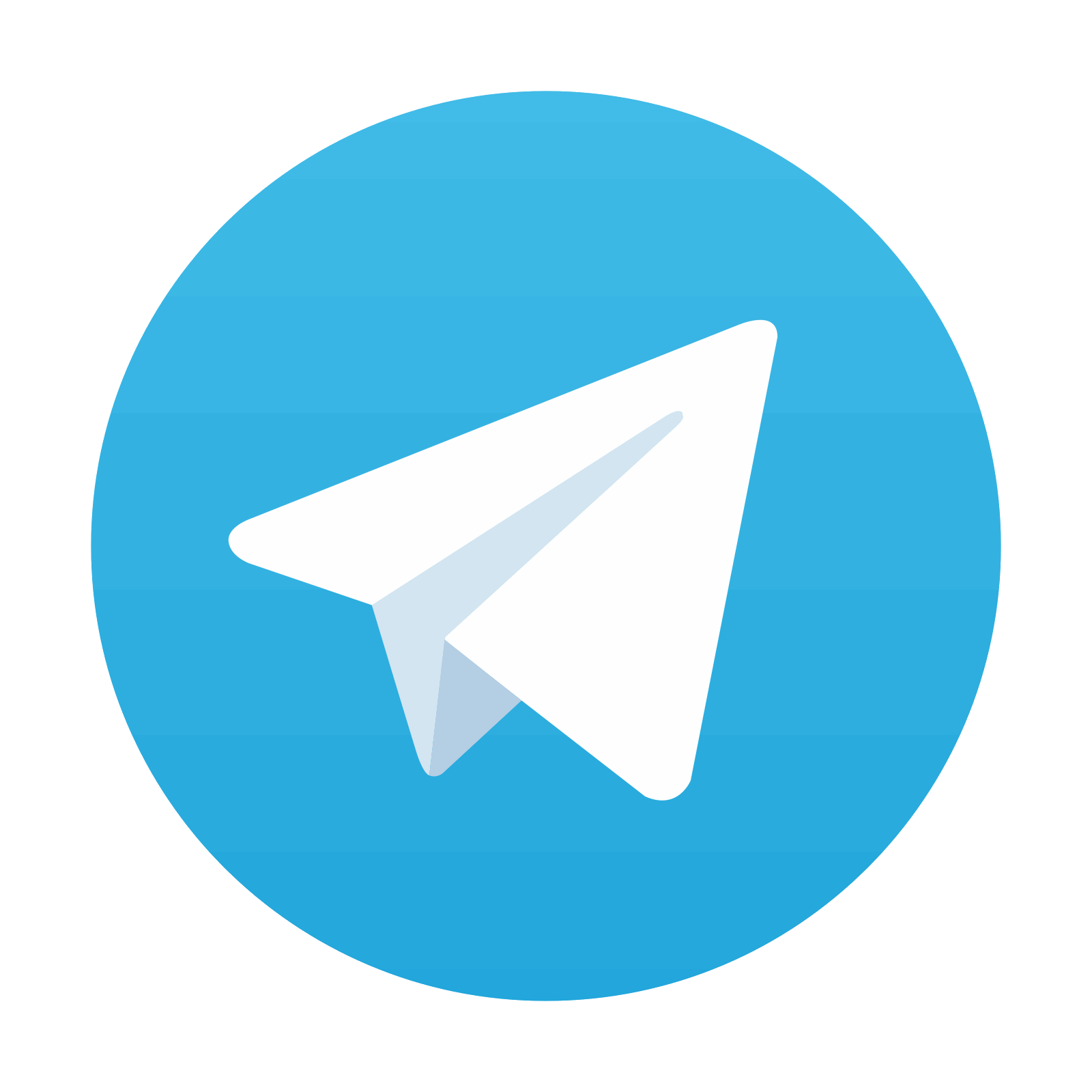
Stay updated, free articles. Join our Telegram channel

Full access? Get Clinical Tree
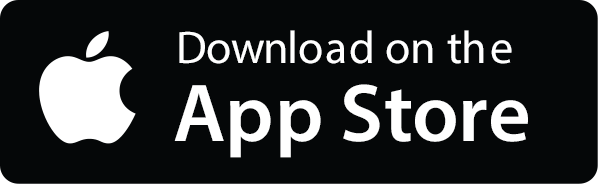
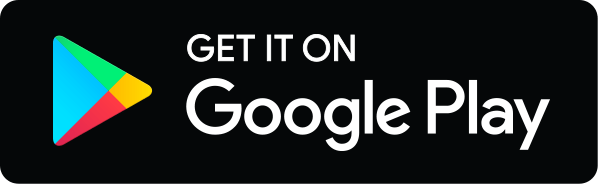