© Springer International Publishing Switzerland 2015
David C. Hess (ed.)Cell Therapy for Brain Injury10.1007/978-3-319-15063-5_55. Clinical Development of MultiStem® for Treatment of Injuries and Diseases of the Central Nervous System
Utilization of an Adult Stem Cell Product for Mitigating Adverse Aspects of Neuroinflammation
(1)
Athersys, Inc., 3201 Carnegie Avenue, 44115 Cleveland, Ohio, U.S.
Keywords
MAPCIschemic strokeNeuroinflammationT regulatory cellsSpinal cord injuryTraumatic brain injurySpleenInterleukin-10MacrophageClinical trialIntroduction
Because of the dynamic biology and potential for working through multiple mechanisms of action, there has been accumulating interest in developing stem cells as therapeutic agents for the treatment of central nervous system (CNS) injury and disease over the past decade [1–5]. Adult-derived stem cells have been isolated from multiple tissues by many laboratories [3, 4], and have been shown to demonstrate a number of favorable characteristics including genetic stability, extensive expansion capacity, and low immunogenicity profiles that support allogeneic utility [5]. Stem cells isolated from other developmental stages (embryonic, fetal, placental, amniotic fluid, etc.), through other distinct “stemness protocols” (induced pluripotency, genetic modification, somatic cell transfer, etc.) or via unique autologous cell isolation procedures or proprietary cell isolation devices, are also the focus of intense academic and industrial research, in an attempt to develop meaningful cell therapies for patients across a spectrum of disease and injury [6].
Regardless of methodology of cell derivation or source of the cell type under consideration, guidance provided by the Food and Drug Administration (FDA) make it clear that isolating, characterizing, and, when relevant, expanding the cell of interest, in a consistent, validated manner must be achieved prior to translating the therapy into patients [7]. Prior to submission of a Biologics License Application (BLA) following the completion of clinical trials for a potential new therapy, a potency assay, based on understanding the mechanism of action for how the cellular product is providing benefit, must be elaborated. And herein lies the challenge to scientists currently working to develop cellular therapeutics—how are cell therapies working? A vast literature demonstrating different cell types, isolated from different tissues, delivered via different routes, in different animal models, in different injury models, at different times, at different doses, almost universally demonstrate cell-mediated benefit—but how and why? If the culmination of these data is true and accepted at face value, is the basic biology underlying the mechanism of action for each cell type conserved? Is the mechanism for one cell type the same when administered through different routes? In different injury and disease models? At different times? Is it tissue replacement via the transplanted cells? Neoangiogenesis? Modulation of the immune system? All of the above? Something as yet undescribed?
Scientists at Athersys, in collaboration with leading academic researchers in the USA and Europe, have focused on studying multipotent adult progenitor cells (MAPC), and the clinical formulation of these cells known as MultiStem. Multiple publications describing the basic biology of these cells have been published. In parallel, experiments comparing and contrasting MAPC to other stem cell populations have been performed and reported. As the biology of MAPC was realized, preclinical studies in a variety of animal models of disease testing the safety and efficacy of the cells were performed. The composite results published led to a refined understanding of the multiple mechanisms through which MAPC may provide benefit when administered and subsequently, clinical translation and testing of the MultiStem product in humans. This chapter outlines the salient biology regarding MAPC, and a summary of the results highlighting the cell’s effectiveness in animal models of CNS injury and disease. Results from those studies led to a conserved hypothesis for a mechanism of benefit for MAPC in acute CNS injury models, and helped define clinical endpoints being collected in the current ongoing phase I/II study enrolling patients having suffered an acute ischemic stroke treated with MultiStem (NCT01436487).
Properties of MAPC
MAPC are an adherent, population of adult stem cells, normally isolated from bone marrow, originally characterized, and described more than a decade ago [8]. Proprietary media formulations and growth conditions have been established and optimized to maintain the distinctive identity, long-term culture expansion, and potency of MAPC isolated from rodent [9] and human tissue [10, 11]. MAPC can be isolated from sources other than bone marrow [12], and have been reported to reconstitute tissues outside of the mesenchymal lineage including hematopoietic cells [13] and neuroectodermal cells [14], among others [8]. MAPC are 15–20 μm in diameter, distinctive from both bone marrow mononuclear cells (~5 μm ) and traditional, mesenchymal stem cells (MSC; 30–35 μm) [15, 16]. Although numerous adult stem cell populations have been isolated and characterized over the past decades [3], MAPC have a unique secretome [17], as well as extended differentiation capability [18], transcriptome [18, 19], and microRNA (miRNA) profiles [10, 19] when compared to other adult adherent cells. Additional aspects of MAPC biology that have been reported and relevant to clinical development follow.
Immunomodulatory Properties
One of the most conserved and translationally important aspects of adult stromal cell biology is the ability to modulate the immune system. Scientific characterization of this class of cells in regard to immunomodulation has been extensive and thoroughly reviewed [20–23], a complete description of which is beyond the scope of this chapter. A summary of some of the immunomodulatory biology characteristics of these cells includes the ability to inhibit T cell activation, B cell activation, natural killer cell activation, dendritic cell maturation, as well as to differentially effect the secretion of a number of inflammatory cytokines.
MAPC are a member of this class of cells and, as such, display immunomodulatory mechanisms equivalent to those listed above, both in vitro and in vivo [20, 24–27], despite evidence demonstrating their distinctiveness from other cells of this class [18]. MAPC also express no detectable major histocompatibility class (MHC) II molecules on their cell surface, making them nonimmunogenic, with no activation of host T cells in in vitro or in vivo testing [24–27]. More recently, it was reported that the use of MAPC in an allogeneic, heterotypic rat heart transplant model leads to MAPC-mediated tolerance and immune acceptance of the heart, when transplanted sequentially into a third animal [16]. This tolerance was shown to be via MAPC-mediated effects on macrophage and T regulatory cell (Treg) populations carried forth in the heart into the recipient.
The nonimmunogenic, tolerizing, and immunomodulatory properties of MAPC led to the translation of the clinical cell product into a completed phase I study for prophylactic treatment of patients at risk for graft-versus-host disease (GvHD) [28], and ongoing clinical studies in a phase I study of solid organ transplant and a phase II study in treatment of ulcerative colitis. Continuing to study and understand the exact molecular mechanisms through which MAPC mediate the repertoire of immunomodulatory functions and applying this knowledge to clinical settings will be paramount in successfully moving these, as well as other cell therapies through the developmental pipeline for treatment of diseases where immune cell dysfunction underlies pathology [29].
Vasculogenic Properties
Numerous cell therapies, either allogeneic or autologous, including different adult stromal cell types, cardiac stem cells, and “cardiotrophic” stem cells, among others, have completed phase I studies in man and are into later-stage clinical evaluation for the treatment of acute myocardial infarction (AMI) or cardiac repair [30, 31]. Although given at different times, different doses, and via different routes across trials, these cell therapies have been generally safe and well tolerated, with a trend towards efficacy.
The evaluation of the MAPC clinical product, MultiStem, in a phase I open-label, dose escalation study of patients suffering AMI [32] was supported by preclinical safety [33] and efficacy data accessing the cells in rodent and pig models of AMI [34–36]. Data from these animal studies demonstrated that percutaneous administration of MAPC leads to a statistically significant improvement in cardiac function of cell-treated versus vehicle-treated animals.
In an attempt to determine the mechanism of benefit following cell administration observed in those studies, analyses of cardiac tissue from cell- versus vehicle-treated animals was completed. An increased neovascularization of the peri-infarct region of cell- versus vehicle-treated animals was observed [33, 34]. Subsequent studies in other models demonstrate that MAPC make relevant blood vessels in vitro [18, 37], more patent blood vessels when transplanted in normal mice [18], more effective revascularization in mice with peripheral limb ischemia injury [38], and increased neovascularization of the ischemic penumbra in mice following an ischemic stroke [39], when compared with equivalent dosages of other stromal cell populations.
In parallel, comparative analyses of MAPC with other adult cell types by both protein secretion and transcriptional profile were performed [17, 18]. Data from these studies were used to help identify three proteins differentially expressed and secreted by MAPC that initiate neo-angiogenesis and vessel formation [40]. The identification of these proteins, and bioactive doses required for vessel formation, has resulted in the development of a multiplex assay for surrogate potency of the cells for clinical manufacturing and lot release in treatment of cardiovascular injury.
Evaluation of MAPC Efficacy in Acute Models of CNS Injury
As a function of understanding the immunomodulatory properties of MAPC, scientists at Athersys initiated a series of collaborative experiments in animal models of acute CNS injury and disease with leading academic translational researchers. The hypothesis that an infusion of cells could downregulate the inflammatory response following administration while potentially providing trophic support for at-risk neurons and brain tissue after injury via vasculogenesis was attractive. The efficacy of MultiStem has subsequently been established in multiple CNS models including: hypoxic-ischemic (HI) injury, spinal cord injury (SCI), ischemic stroke, and traumatic brain injury (TBI), and results from these studies are summarized below.
HI Injury
The effect of MAPC on HI injury was assessed in neonatal rat studies performed in collaboration with Dr. Jim Carroll and Dr. David Hess at the Medical College of Georgia (now known as Georgia Regents University) [41, 42]. Data from these studies indicated that allogeneic or xenogeneic MAPC could be administered directly into the hippocampus 7 days after injury, or could be given intravenously (IV) 7 days after induction of injury, and that regardless of cell type or route, statistically significant improvements in locomotor behavior were maintained out to 4 weeks after a single-cell administration compared to vehicle-treated animals. Interestingly, animals that received cells IV had increased locomotor activity at 4 weeks when compared to animals that received the same dose of cells into the hippocampus, suggesting either enhanced benefit via the IV route or, alternatively, adverse aspects of a direct intraparenchymal administration.
Spinal Cord Injury
Previous work in a nontranslational dorsal column crush model of SCI, performed in collaboration with Dr. Jerry Silver and his colleagues at Case Western Reserve, demonstrated that administration of MAPC into the spinal cord immediately following injury results in a decrease in microglial/macrophage activation at the lesion over the first 7 days post injury [43]. There was also a concomitant decrease in axonal dieback and a statistically significant increase in axon regrowth towards the site of the lesion in cell- versus vehicle-treated rodents. Additional in vitro experiments and biochemistry supported the contention that the MAPC-mediated benefit observed in vivo was via a direct modulation of the macrophages from an M1 to an M2 phenotype, thereby decreasing macrophage-mediated dieback on the axons, which had been previously demonstrated to be the major initiator of long-range axonal retraction [44, 45].
To address the potential benefits of MAPC on SCI, additional studies were performed on a more relevant contusion model of injury. Pilot studies were performed to determine the optimal route of administration in the contusion injury model, and it was determined that IV administration was superior to direct injection into the cord following injury (DePaul et al., unpublished observations). Subsequently, we sought to determine the optimal window of administration and optimal single dose of the cells in the contusion model of SCI through a series of outcome assessments including the Basso–Beattie–Bresnahan locomotor scoring and catwalk regularity index, physiological readouts including measuring urination every 2 weeks using metabolic cages and endpoint urodynamic testing, tissue immunohistochemistry and biodistribution of labeled MAPC, and microarray analysis. The data demonstrate that a single IV dose of at least 4 million cells, administered 24 h after induction of the SCI, results in sustained and statistically significant locomotor benefit for at least 10 weeks after treatment [46]. Additionally, cell-treated animals urinated at a smaller bladder volume, had less residual volume, showed improved return to baseline pressure following a void, and had a decrease in bladder weight compared to vehicle-treated animals. Increasing the dose to 8 million cells showed no increase in additional locomotor recovery, but did improve bladder function [47].
Biodistribution analysis of labeled MAPC in collaboration with BioInVision indicated cells were found in the lungs, liver, and spleen at 24 h post IV administration. Normalizing cell counts to tissue weight showed a preferential homing to the spleen, with few cells found in the spinal column. Microarray analysis of the lesion, blood, and spleen suggests MAPC alters many injury-induced pathways including those involved in recruitment, activation, and migration of immune cells. In support of these data, we found a decrease of ED1 +macrophages by immune-histochemical staining at the lesion site 4 days post injury, when compared to untreated animals [47]. Taken as a whole, these data suggest that MAPC, when administered IV in an acute contusion model of SCI, are more likely to exert benefit through peripheral immune organ systems than via homing and direct interaction with the site of injury [46, 47].
Initial Ischemic Stroke Studies
While completing the neonatal HI injury studies being conducted at Georgia Regents University, adult rat ischemic stroke studies were also performed there to address relevant translational questions regarding the use of MAPC to treat ischemic injuries of the brain [48]. Data from these studies extended the understanding of MAPC-mediated benefit to include: (1) no immunosuppression was required when administering the cells through either intraparechymal or IV routes; (2) cell-mediated benefit was observed when given via both routes; however, higher doses were required, when given IV, to reach the same level of benefit observed at lower doses given directly into the brain (4 million IV = 400,000 direct injection); (3) the window for IV administration of cells can be extended out to 7 days for sustained significant benefits; however, earlier is better for minimizing the amount of brain tissue lost following onset of the stroke (i.e., 1 day > 2 days > 7 days). These results helped us address dose ranging, route of administration, and window of therapeutic benefit criteria for the Investigational New Drug (IND) submission for using the cells to treat ischemic stroke. However, an examination of the peri-infarct regions of the cell-treated stroke-injured brain revealed the presence of very few detectable MAPC, suggesting that the direct recovery observed was not likely the result of migration of MAPC to the site of injury and support of at-risk tissue through trophic factor secretion.
Traumatic Brain Injury
In an attempt to extend our understanding of how MAPC could be providing the neuroprotection observed in the initial stroke study [48], a collaboration with Dr. Charles Cox at the University of Texas, Houston (UT-Houston), was started to investigate the potential relationship between IV-infused MAPC and the spleen following acute injuries of the CNS. Previous publications from multiple laboratories had described the central role that the spleen has in exacerbating neuroinflammation following onset of an initial “inflammatory event,” i.e., stroke, etc. [49–52].
The importance of the spleen as a target organ-mediating MAPC benefit was confirmed when cells were administered in the first 24 h following induction of TBI in rodents [53, 54]. After the injury, the blood–brain barrier (BBB) opens, contributing to edema and influx of inflammatory cells from the periphery to the CNS. Cells coming from the spleen are directly responsible for the loss of BBB integrity seen after TBI, as inducing injury in animals that had previously had their spleen removed results in little increase in BBB permeability [53]. IV infusion of MAPC blocks the spleen-mediated increased permeability of the BBB after injury; it is interesting to point out that labeled MAPC accumulate in the spleen in a dose-dependent way when IV infused after TBI injury [53]. The accumulation of MAPC into the spleen following IV administration within 24 h after acute CNS injury has now been confirmed in at least two other models in addition to TBI, ischemic stroke (Bang, unpublished results), and contusive SCI [46, 47].
The IV administration of MAPC within the first 24 h after TBI injury also results in other immune and behavioral changes. Cell-treated animals display an increase of the anti-inflammatory cytokines interleukin 4 (IL-4) and IL-10 emanating from the spleen with a simultaneous decrease in transcripts for the inflammatory cytokines IL-6 and interferon gamma when compared to TBI animals receiving vehicle [53]. There were statistically significant improvements in locomotor outcomes, and long-term spatial memory and memory retrieval (120 days post treatment) in MAPC-treated injured animals compared with vehicle-treated injured animals, and these improvements correlated with long-term diminution of microglial activation in the brains of the cell- versus vehicle-treated animals [55]. The compilation of these data lends strong support to the potential for moving MAPC into clinical development for treatment of TBI or concussive injuries.
Secondary Ischemic Stroke Injury Studies
In parallel to the TBI studies at UT-Houston, Athersys collaborated with Dr. Sean Savitz to confirm the efficacy of MAPC previously observed in rodent models of ischemic stroke [48]. A series of studies were designed to extend the translational information already established in ischemic stroke, coupled with the new observations relating to MAPC-mediated benefit via the spleen in models of TBI.
Experiments were performed in a new species of rat, via a new stroke injury protocol using a more robust series of outcome measures and confirmed that MAPC administration within 24 h of injury provided a statistically significant benefit [56]. There was also a statistically significant decrease in the inflammatory cytokines IL-6 and IL-1b when comparing cell-treated to vehicle-treated stroke animals along with a concomitant approximately sevenfold increase in IL-10 in cell-treated animals, 3 days after treatment (4 days after induction of injury). Finally, when the spleens of stroke animals given cells were compared with spleens from stroke animals given placebo, numerous statistically significant differences were observed including a preservation of splenic mass in the cell treatment, a decrease in TUNEL-positive apoptotic cells in the cell treatment, and upregulation of the transcript for IL-10 in the cell treatment [56]. These data led to an even greater focus and effort to understand the relationship between MAPC/spleen interactions following CNS injury on the molecular level.
To gain perspective, the collaborators isolated brain tissue and spleen tissue from stroke-injured rats that were treated 24 h after injury with cells or placebo. Tissue was isolated at both 3 days and 28 days after treatment from three different groups of animals: sham injured, stroke injured receiving placebo, and stroke injured receiving 4 million MAPC. Nucleic acid was isolated and microarray analyses were performed. Previously, microarray analyses of the brains of stroke-injured mice that received either a hippocampal injection of 100,000 human MSC or saline, 24 h after induction of the injury, had been reported [57]. This experiment yielded an enormous amount of data regarding MSC-mediated benefit in the brain following stroke. The authors of that study concluded the observed beneficial effects of the transplanted cells were largely explained by their modulation of inflammatory and immune responses, in the brain, by modulating activation of microglia and/or macrophages.
Although similar in many ways, the MAPC microarray stroke study differed most notably in the cell type being infused (MAPC vs. MSC), the species of investigation (rat vs. mouse), the route of administration (IV vs. direct hippocampal injection), and the number of cells being infused (4 × 106 vs. 1 × 105). Data generated in the MAPC/rat stroke study similarly suggested that transplanted cells were decreasing the inflammatory status of the stroke-injured brain, while simultaneously upregulating anti-inflammatory programs and signaling pathways in the brain despite IV administration (Hamilton, unpublished data). It is hypothesized that the observed inflammatory changes in the brain were taking place as a result of decreased migration and infiltration of peripheral immune cells to the site of injury, specifically from the spleen, as quantification of microarray markers for activated T cells and M1 macrophages were upregulated hundreds of fold in vehicle-treated stroke animals, and decreased almost back to the levels of sham in cell-treated stroke animals (Hamilton, unpublished data). Migration of a significant number of activated cells from the spleen to the peri-infarct region of the brain in the first days after stroke has previously been demonstrated [58]. This observed splenocyte migration correlates with a decrease in splenic mass similar to what has been observed by several groups [56, 58, 59]. Microarray analysis of spleen tissue after stroke suggests that cell-treated animals have a normal “healthy” splenic phenotype comparable to sham-injured animals (i.e., normal bioenergetics, cell division, oxidative phosphorylation pathway signatures, etc.), while vehicle-treated stroke-injured animals have an increase in proapoptotic genes, and immunosuppressive gene ontogeny signatures not observed in cell-treated injured animals (Hamilton, unpublished).
To directly test the importance of the spleen in MAPC-mediated benefit in stroke, a series of experiments were performed comparing injured animals that received MAPC to animals that had their spleens removed 14 days prior to stroke injury, and then received IV cell infusion [60]. The first observation was that the cell-mediated functional recovery observed in stroke animals with their spleens was completely lost in splenectomized cell-treated animals [60]. Interestingly, animals that had their spleens removed had a lower initial baseline deficit score, regardless of treatment group, when compared to animals with spleens, although no deficit improvement was observed over the 4-week testing period. Also, animals that had splenectomies prior to stroke injury showed a statistically decreased infarct volume compared to animals with spleens, as had previously been reported [51]. MAPC administration to stroke-injured animals, both with or without their spleens, resulted in a statistically significant approximately fourfold preservation of brain tissue 28 days after treatment. This is remarkable in light of the fact that no significant functional recovery was seen in cell-treated animals at 28 days, despite the preservation of brain tissue [60].
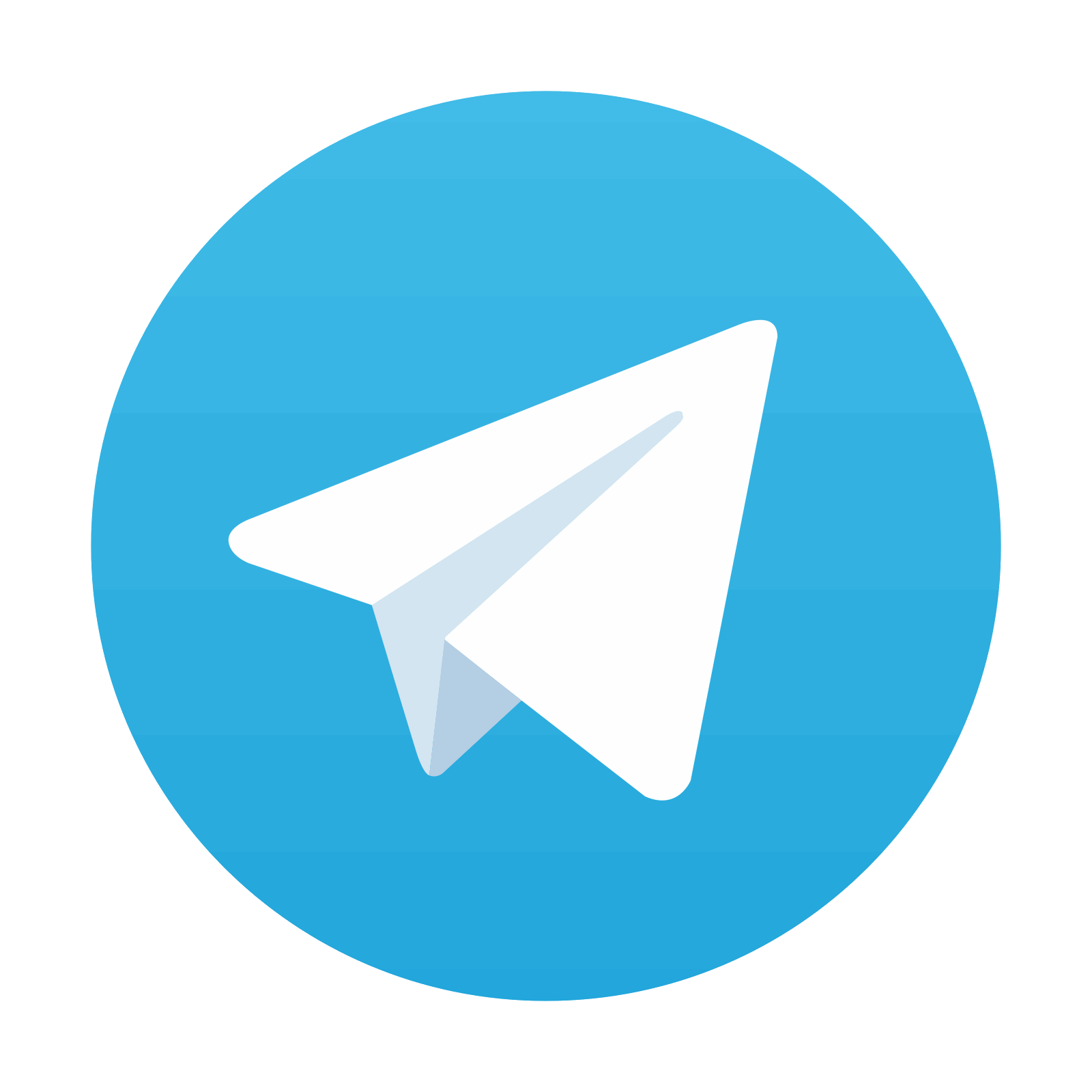
Stay updated, free articles. Join our Telegram channel

Full access? Get Clinical Tree
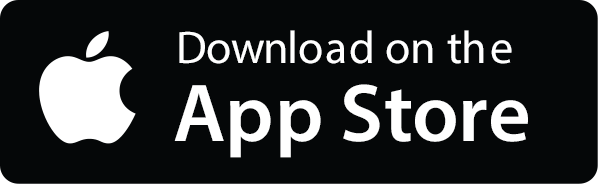
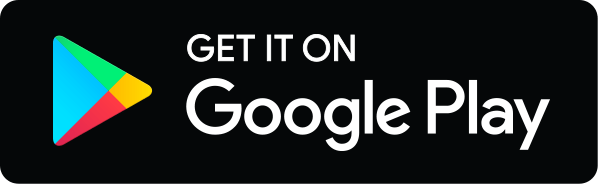