1CHAPTER 1
Clinical Neurophysiology: An Overview
Edward C. Mader, Jr., Daniella Miller, and Piotr W. Olejniczak
CLINICAL NEUROPHYSIOLOGIC TESTING
Clinical neurophysiology (CNP) is a time-honored medical specialty that continues to make great strides, bolstered by rapid advances in neuroscience, biomedical engineering, and computer technology. It encompasses a wide range of methods and techniques for recording, presenting, and analyzing neurophysiologic signals in order to diagnose sensory, motor, autonomic, and central nervous system disorders. Testing performed in CNP or procedures used in current neurological practice include a variety of modality-specific and mixed-modality tests (Table 1.1).
Modality-specific CNP tests are performed to assess specific functional modalities using biomedical instruments that measure changes in neurophysiologic signals that occur spontaneously or with activation. Spontaneous fluctuations in electrical and magnetic fields during brain cortical activity are detected with EEG and magnetoencephalography (MEG) (1,2). Changes in electrical potentials with nerve and muscle activity are measured with electromyography (EMG) and nerve conduction studies (NCSs) (3). Subtle changes in the function of signaling pathways can be detected with evoked potentials (EPs), of which the most clinically useful are somatosensory (SSEPs), brainstem auditory (BAEPs), visual (VEPs), and motor (MEPs) evoked potentials (4). SSEPs are elicited by electrical stimulation of the median nerve (M-SSEP), the tibial nerve (T-SSEP), and rarely from other peripheral nerves. MEPs are activated by means of transcranial electrical stimulation (TES-MEP) or transcranial magnetic stimulation (TMS-MEP). The brain’s ability to anticipate or respond to cognitive events is examined using event-related potentials (ERPs), such as the Bereitschaftspotential (BSP), contingent negative variation (CNV), P300 (P3), and mismatch negativity (MN) (5). CNP autonomic function testing includes measurement of heart rate variability with deep breathing (HR-DB), recording of blood pressure (BP) and heart rate (HR), response to head-up tilt (HUT) and Valsalva (VAL) maneuver, and sudomotor function by means of the quantitative sudomotor axon reflex test (QSART) and the thermoregulatory sweat test (TST) (6).
Mixed–modality (aka multi-modality) CNP tests utilize two or more test modalities to assess complex states (e.g., sleep, coma), to track multiple physiologic parameters, or to obtain more accurate results. During sleep studies, scalp EEG, chin EMG, and EOG (electrooculogram) allow tracking of sleep–wake stages; additional physiologic processes, such as nasal airflow, ventilatory effort, snoring, blood oxygen saturation, ECG, and anterior tibialis EMG, are recorded during polysomnography (PSG) (7). As a rule, neurophysiologic intraoperative monitoring (NIOM) is unimodal with the test modality chosen based on the structures at risk during the procedure, but multimodal NIOM may be superior for selected surgical procedures, such as combining SSEP and MEP monitoring during surgery on the spine (8). In multimodal neuromonitoring (MNM), cerebral blood flow, brain tissue oxygenation, and brain tissue metabolism are monitored, in addition to intracranial pressure (ICP) and EEG, to improve outcome in acute brain injury (9). The multimodal approach, known as coregistration, involves superimposing the data of two or more test modalities to achieve a level of accuracy that is not attainable if each modality is analyzed separately (10). Examples of coregistration techniques are electric source imaging (ESI), magnetic source imaging (MSI), and combined electric-magnetic source imaging (EMSI).
Specific test methods or protocols are selected based on test objectives, patient characteristics, clinical setting, and other factors (11–14). While the routine approach is sufficient in most cases, some clinical problems and situations require modifications or refinements in the routine method. For example, the following EEG methods and protocols are aimed at different clinical scenarios: routine scalp EEG, extended EEG recording, ambulatory EEG, neonatal EEG, brain death study, ICU-EEG monitoring, IOM-EEG monitoring, long-term monitoring (LTM) of video and EEG in the epilepsy monitoring unit (EMU), electrocorticography (ECoG), stereo-EEG, and coregistration techniques, such as ESI and EMSI. It may be prudent to combine different test modalities to obtain more accurate results, such as simultaneous EEG and EMG recording to better define the seizure type and EEG recording during HUT to distinguish postsyncopal convulsions from epileptic seizures and psychogenic events.
2TABLE 1.1: Clinical Neurophysiologic Tests in Current neurological Practice
EEG Routine scalp EEG (adult/pediatric/neonatal) Ambulatory scalp EEG monitoring Electrocorticography Stereo-electroencephalography Epilepsy monitoring unit EEG ICU-EEG monitoring Intraoperative EEG monitoring | Cortical electrical potential (EEG) EEG recorded on the scalp EEG recorded with subdural electrodes EEG recorded with depth electrodes EEG recorded on the scalp or intracranially Same as scalp EEG Same as scalp EEG |
MEG Spontaneous MEG Evoked MEG | Cortical magnetic flux density (MEG) Cortical magnetic flux density (MEG) |
EMG Needle EMG Single-fiber EMG Surface EMG | Motor unit action potential Single-muscle-fiber action potential Myogenic electrical potential (surface EMG) |
NCS Sensory nerve conduction studies Motor nerve conduction studies Including repetitive stimulation and F-wave studies Sensory–motor reflex studies including H reflex, blink reflex, and LLR studies Intraoperative CMAP monitoring | Sensory nerve action potential CMAP: M wave CMAP: M wave, F wave CMAP: H wave CMAP: R1 and R2 responses, LLR response Same as M-NCS |
EP studies Pattern-shift VEPs Flash VEPs FVEPs BAEPs SSEP via median/tibial nerve stimulation MEP via transcranial electric/magnetic stimulation Intraoperative MEP monitoring Intraoperative SSEP monitoring Intraoperative BAEP monitoring Intraoperative FVEP monitoring | Cortical electrical potential (P100, N100) Electrical potentials I, II, III, IV, V, VI Auditory nerve (I), brainstem (II–V) potential Peripheral nerve (N9/N8), cord (N13/N22), subcortical (P14, N18/P31, N34), cortical (N20/P37) Direct wave, indirect waves, and CMAP: M wave Same as MEP Same as M-SSEP/T-SSEP Same as BAEP Same as FVEP |
Event-related potential studies Readiness or BSP CNV Oddball paradigm MN | 3Cortical electrical potential (BSP or premotor potential) Cortical electrical potential (CNV) Cortical electrical potential (P300 or P3) Cortical electrical potential (MN) |
Autonomic function tests HR variability with deep breathing Head-up tilt or tilt table test Valsalva maneuver Quantitative sudomotor axon reflex test Thermoregulatory sweat test | Cardiac electric potential (ECG) BP, HR BP, HR Sweat volume Sweat distribution |
Polygraphic sleep studies PSG Multiple sleep latency test Maintenance of wakefulness test | Sleep stage: scalp EEG, chin EMG, and EOG Other parameters (PSG): respiratory airflow and effort, blood oxygenation, ECG, tibialis anterior EMG, etc. |
Multimodal neuromonitoring Multimodal intensive care neuromonitoring Multimodal intraoperative neuromonitoring | Intracranial pressure, brain perfusion, oxygenation, etc. Two or more types of signal or test parameters |
Multimodal coregistration Electric source imaging Magnetic source imaging Electric and magnetic source imaging | EEG coregistered with brain neuroimage MEG coregistered with brain neuroimage EEG and MEG coregistered with brain neuroimage |
Note: The first seven categories comprise modality-specific tests, and the last three categories consist of mixed-modality tests (see text for explanation). Listed under each test category are test methods or protocols aimed at specific clinical settings and test objectives. The right column shows the signal of interest or physiologic parameter(s) recorded during neurophysiologic testing.
BAEP, brainstem auditory evoked potential; BP, blood pressure; BSP, Bereitschaftspotential; CMAP, compound motor action potential; CNV, contingent negative variation; EMG, electromyography; EOG, electrooculogram; EP, evoked potential; FVEP, flash visual evoked potential; HR, heart rate; LLR, long-latency reflex; MEG, magnetoencephalography; MEP, motor evoked potential; MN, mismatch negativity; M-NCS, motor nerve conduction study; M-SSEP, median nerve somatosensory evoked potentials; NCS, nerve conduction study; PSG, polysomnography; SSEP, somatosensory evoked potentials; T-SSEP, tibial nerve somatosensory evoked potentials; VEP, visual evoked potentials.
4CNP tests measure specific biosignals or physiological parameters (Table 1.1) allowing clinicians to probe and assess selected facets of the patient’s functional anatomy (Table 1.2). The biosignals or physiological parameters recorded reflect the function of specific anatomical structures (Table 1.3). Based on recorded signal characteristics (Figures 1.2 and 1.3), the clinical neurophysiologist can tell if anatomical structures, circuits, or pathways are functioning normally or are affected by pathological processes (Tables 1.4 and 1.5).
Brain function is reflected in the EEG, MEG, ERP, and EP cortical and subcortical components (1,2,6,8). Even when EEG and MEG signals arise from the same cellular processes in the cerebral cortex, there are differences in the way EEG and MEG data are recorded and interpreted (15). A reference is required to record EEG, but not MEG. Because the skull and scalp do not distort magnetic fields as much as electrical fields, the spatial resolution of MEG is superior to EEG (1,2). EEG can detect activity in “more” brain areas by recording radial dipoles, but MEG is more sensitive to tangential dipolar activity in the superficial cortical sulci, making it superior for source localization; for example, MEG is used for MSI and more precise localization of the epileptogenic focus (2). While EEG is widely used in clinical practice, MEG is available only in far fewer centers. The constant interaction between cortical and subcortical structures implies that diencephalic-brainstem disturbances will also affect the EEG/MEG. Assessment of modality-specific brain structures is performed with VEP, BAEP, SSEP, LLR, ERP, and other CNP tests (see later) (3–6). Brain function assessment is the goal of IOM-EEG, sleep studies, and MNM of cerebral perfusion, oxygenation, and metabolism (1,7–9).
Muscle, peripheral nerve, and lower motor neuron function is routinely tested with needle EMG and NCS (3,16). During needle EMG, motor unit action potential (MUAP) number and firing rate increase with the force of muscle contraction. Each MUAP represents activation of a motor unit (i.e., one motor neuron, its nerve fiber, and all muscle fibers innervated by branches of the same nerve fiber). NCS is performed to assess signal conduction along fast-conducting large-diameter nerve fibers: S-NCS for sensory and M-NCS for motor nerve fibers. Neuromuscular junction transmission is evaluated by repetitive nerve stimulation and single-fiber EMG (3,16). F-wave recording extends the utility of M-NCS for evaluating lower motor neurons, motor nerve roots, and proximal motor nerves. These motor structures, along with the sensory nerve roots and central components of reflex pathways, can be evaluated with the H reflex, long-latency reflex, or blink reflex (3,17).
Modality-specific neural pathway or circuit activity is reflected in the EP or ERP signals that are generated when components of the pathway or circuit are activated (4,5,8). The dorsal column pathway is evaluated with M-SSEP and T-SSEP (18), the visual pathway with pattern-shift or flash VEP (19), the auditory pathway with BAEP (20), and the central motor pathway with TES-MEP or TMS-MEP (21). IOM-SSEP and IOM-MEP can be combined if both dorsal and ventral territories of the spinal cord are surgically at risk (22). The neurophysiological substrates of ERP have not yet been identified with certainty. BSP and CNV correlate with movement preparation and motor processing in the frontal lobe, P300 with selective attention and stimulus discrimination in the parietal lobe, and MN with sound feature discrimination, sensory learning, and perceptual accuracy (5,23).
Autonomic regulation of somatic and visceral function by the parasympathetic and sympathetic nervous system is mediated via slow-conducting small-fiber autonomic nerves that innervate most organs and tissues (6,24). Some autonomic function tests are organ specific, such as pupillometry, lacrimation tests, tests of saliva production, gastric motility tests, and urodynamic studies. In CNP, autonomic testing is performed to evaluate parasympathetic cardiovagal reflexes (HR-DB and VAL), sympathetic vasomotor reflexes (VAL and HUT), and sympathetic sudomotor function (QSART and TST). QSART evaluates the sympathetic axon reflex and TST evaluates the integrity of the sympathetic thermoregulatory pathway from the hypothalamus to eccrine sweat glands (6,24).
The sensitivity of CNP tests is remarkable and most tests will show abnormal results before the onset of clinical signs and symptoms. Unfortunately, CNP tests are also sensitive to technical factors and to noise. Exceptional care should therefore be exercised always to avoid overinterpreting neurophysiologic data. The specificity of CNP tests is limited to the identification of physiological disturbances or disorders in functional anatomy. To reach an etiologic diagnosis, neurophysiological data should always be interpreted in the context of the clinical history, physical examination, and other laboratory test results.
5TABLE 1.2: Classification of Clinical Neurophysiologic Tests Based on Functional Anatomy or Neural Pathway Tested
Tests of global/focal cortical, diencephalic, and/or brainstem function EEG—spontaneous, scalp-based cortical activity, including influence of diencephalic and brainstem structures ECoG—focal/regional brain activity, including influence of diencephalic and brainstem structures Stereo-EEG—focal limbic or deep-brain activity influenced by other cortical and noncortical structures Epilepsy monitoring unit EEG—same as EEG, ECoG, or stereo-EEG ICU-EEG—same as EEG IOM-EEG—same as EEG MEG—same as EEG, but superior for detecting localized cortical activity MNM—global cerebral perfusion, oxygenation, metabolism, neocortical activity (see EEG) Sleep studies—same as EEG, with emphasis on the function of the brain’s sleep and wake centers |
Tests of muscle, peripheral nerve, motor neuron, and/or reflex function Needle EMG—motor units activated by volition, spontaneous activity of muscle fibers/motor units Single-fiber EMG—neuromuscular junction and muscle fiber activity Surface EMG—multiple motor units activated spontaneously or volitionally S-NCS—large-fiber sensory peripheral nerve fibers M-NCS—large-fiber motor peripheral nerve fibers, neuromuscular junction, and muscle fibers Repetitive stimulation—same as motor NCS, with emphasis on neuromuscular junction transmission F-wave study—large-fiber motor peripheral nerve fibers, muscle fibers, multiple motor units IOM-CMAP—same as motor NCS H reflex—large-fiber peripheral sensory nerve fibers and multiple motor units (H wave) Long-latency reflex—large-fiber sensory pathway, somatomotor cortex, corticospinal tract, multiple motor units Blink reflex—trigeminal nerve V1 branch, nuclei and tracts in pons and medulla, facial nerve, orbicularis oculi fibers |
Tests of central sensory/motor pathway and/or focal cortical function VEP—QSART, quantitative sudomotor axon reflex testion, visual cortex BAEP—auditory pathway: ear, auditory nerve, cochlear nucleus, lateral lemniscus, inferior colliculus M-SSEP—somatosensory pathway: median nerve, dorsal column, medial lemniscus, thalamus, somatosensory cortex T-SSEP—somatosensory pathway: tibial nerve, dorsal column, medial lemniscus, thalamus, somatosensory cortex TES-MEP—motor pathway: motor cortex, corticospinal tract, multiple motor units TMS-MEP—motor pathway: motor cortex, corticospinal tract, multiple motor units IOM-SSEP—same as M-SSEP/T-SSEP IOM-MEP—same as TES-MEP/TMS-MEP BSP—frontal lobe premotor cortex circuits involved with movement preparation and execution CNV—frontal lobe premotor cortex circuits involved with movement preparation P300—parietal lobe circuits for selective attention and stimulus discrimination MN—temporal lobe auditory cortex circuits for sound feature discrimination ESI, MSI, or EMSI—focal area of cerebral cortex generating signal of interest (e.g., epileptiform discharges) |
6Tests of autonomic function HR-DB—parasympathetic cardiovagal reflexes HR-VAL—parasympathetic cardiovagal pathways BP-VAL—sympathetic baroreflex afferent and efferent pathways BP-HUT—sympathetic baroreflex afferent and efferent pathways QSART—sympathetic postganglionic sudomotor axon reflex TST—sympathetic central, preganglionic, postganglionic pathways and eccrine sweat glands |
Note: Modality-specific tests assess the function of specific structures or neural circuits and mixed-modality tests assess multiple functional modalities or complex states (see Table 1.1). Physiological function is assessed by measuring signals generated by specific anatomical structures during a brief time interval (routine method) or by tracking how signals change over time (continuous monitoring).
BAEP, brainstem auditory evoked potential; BSP, Bereitschaftspotential; BP-HUT, blood pressure head-up tilt; BP-VAL, blood pressure Valsalva; CMAP, compound motor action potential; CNV, contingent negative variation; ECoG, electrocorticography; EMG, electromyography; EMSI, Electric and magnetic source imaging; ESI, electric source imaging; HR-DB, heart rate variability with deep breathing; HR-VAL, heart rate Valsalva; IOM, intraoperative monitoring; MEP, motor evoked potential; M-NCS, motor nerve conduction studies; NCS, nerve conduction study; MEG, magnetoencephalography; MN, mismatch negativity; MNM, multimodal neuromonitoring; M-SSEP, median nerve somatosensory evoked potential; MSI, magnetic source imaging; QSART, quantitative sudomotor axon reflex test; S-NCS, sensory nerve conduction study; T-SSEP, tibial nerve somatosensory evoked potential; TES-MEP, transcranial electrical stimulation MEP; TMS-MEP, transcranial magnetic stimulation motor evoked potential; TST, thermoregulatory sweat test; VEP, visual evoked potential.
TABLE 1.3: Generators and Sources of Signal and Test Parameters Measured in Clinical Neurophysiology
EEG/MEG EEG, ECoG MEG | Cortical electrical potentiala Cortical magnetic flux densityb | Cortical pyramidal cell synaptic potential Cortical pyramidal cell electric currents |
EMG/NCS Needle EMG Single-fiber EMG Surface EMG | Motor unit action potential MFAP Myogenic electric potential | MFAP MFAP MFAP |
Sensory NCS Motor NCS F waves H reflex Long-latency reflex Blink reflex | Sensory nerve action potential CMAPc CMAP: F wavesb CMAP: H responseb CMAP: LL responsesb CMAP: R1 and R2 responses | Nerve fiber action potential MFAP MFAP MFAP MFAP MFAP |
EP/ERP | 7 | |
VEP | Cortical potential (P100, N100) | Cortical pyramidal cell synaptic potential |
FVEP BAEP | Electrical potentials I, II, III, IV, V, VIc Auditory nerve action potential (I) Brainstem farfield potential (IIc, III, IV, V) | Nerve fiber and synaptic potentials Nerve fiber action potential Brainstem electric potential |
M-SSEP | Peripheral nerve potential (N9) Cervical cord dorsal horn potential (N13) Subcortical potential (P14, N18)c Somatosensory cortical potential (N20) | Nerve fiber action potential Dorsal horn cell synaptic potential Synaptic/action potential Cortical pyramidal cell synaptic potential |
T-SSEP | Peripheral nerve potential (N8) Thoracic cord dorsal horn potential (N22) Subcortical potential (P31, N34)c Somatosensory cortical potential (P37) | Nerve fiber action potential Dorsal horn cell synaptic potential Synaptic/action potential Cortical pyramidal cell synaptic potential |
MEP | Pyramidal tract potential (D wave, I waves) Compound motor action potential (CMAP) | Nerve fiber action potential MFAP |
ERP | Cortical potential | Cortical pyramidal cell synaptic potential |
Autonomic tests HR-DB HUT/VAL QSART/TST | Electrocardiographic potential (ECG) Arterial BP (mmHg), heart rate (/min) Eccrine gland output (sweat) | Cardiac MFAP Not applicable Not applicable |
Sleep studies | 8 | |
EEG (scalp) EMG (chin) EOG (near eye) | Cortical electric potentiala Myogenic electric potential Oculoelectric dipole | Cortical pyramidal cell synaptic potentiala MFAP Corneal–retinal potential |
Other parametersd | Respiratory airflow Respiratory effort Blood oxygenation Cardioelectric potential (ECG) Tibialis anterior muscle potential | Not applicable Not applicable Not applicable Cardiac MFAP MFAP |
Neuromonitoring Unimodal NIOM Multimodal NIOM MNM | Same as EEG, CMAP, MEP, SSEP, etc. ≥2 modalities, e.g., IOM-SSEP and MEP ICP, brain perfusion, brain tissue oxygenation, cellular metabolism, etc. | Same as modality-specific test Same as modality-specific test Not applicable Not applicable |
Coregistration ESI MSI EMSI | Same as EEG + electromagnetic Same as MEG + electromagnetic Same as EEG and MEG + electromagnetic | See EEG above See MEG above See EEG and MEG above |
Note: This table shows (a) CNP test or test modality (left column); (b) the macroscopic generator and signal or physiologic parameter measured by the test (middle column); and, whenever applicable, (c) the microscopic SGU and the microscopic signal or “building block” of the macroscopic signal (right column).
BAEP, brainstem auditory evoked potential; BP, blood pressure; CMAP, compound motor action potential; ECoG, electrocorticography; EMG, electromyography; EMSI, electric-magnetic source imaging; EOG, electrooculogram; EP, evoked potential; ERP, event-related potentials; ESI, electric source imaging; FVEP, flash visual evoked potentials; HR, heart rate; HR-DB, heart rate variability with deep breathing; HUT, head-up tilt; ICP, intracranial pressure; IOM-SSEP, intraoperative somatosensory evoked potentials monitoring; MEG, magnetoencephalography; MEP, motor evoked potential; MFAP, muscle fiber action potential; MNM, multimodal neuromonitoring; MSI, magnetic source imaging; M-SSEP, median nerve somatosensory evoked potentials; NCS, nerve conduction study; NIOM, neurophysiologic intraoperative monitoring; QSART, quantitative sudomotor axon reflex test; SGU, signal-generating unit; SSEP, somatosensory evoked potentials; TST, thermoregulatory sweat test; T-SSEP, tibial nerve somatosensory evoked potentials; VAL, Valsalva; VEP, visual evoked potentials.
aNonsynaptic and nonneuronal mechanisms may also play a role in EEG/MEG generation.
bLate responses are CMAPs that occur after the classic CMAP known as M wave.
cGenerator(s) of FVEP waves I–VI, BAEP wave II, and SSEP subcortical components remain controversial.
dPhysiologic parameters recorded during PSG in addition to EEG, EOG, and chin EMG (list is incomplete).
Translating bioelectric and biomagnetic signals into physiological information requires a priori knowledge of where and how signals are generated (discussed earlier) and how signals spread from the generator to the recording site. Once generated, bioelectric and biomagnetic fields propagate from the generator to other parts of the body. This mechanism of signal propagation, known as volume conduction, must be distinguished from another mechanism of signal propagation, known as neural transmission. These two mechanisms of signal propagation in biological tissue are quite distinct and result in waveforms with different spatiotemporal characteristics (Figure 1.4).
9TABLE 1.4: Spatiotemporal Characteristics of Bioelectric Signals Measured in Clinical Neurophysiology
EEG | Cortical electric potentiala | Stationary nearfield |
MEG | Cortical magnetic flux densitya | Stationary nearfield |
Needle EMG Single-fiber EMG | Motor unit action potential MFAPb | Traveling nearfield Traveling nearfield |
Sensory NCS Motor NCS Reflexes: H, LL, and blink | Sensory nerve action potential CMAPc CMAPc | Traveling nearfield Traveling nearfield Traveling nearfield |
Pattern-shift VEP | Cortical potential (P100, N100) | Stationary nearfield |
BAEP | Auditory nerve action potential (I) Brainstem farfield potential (IIc, III, IV, V) | Traveling nearfield Stationary farfield |
M-SSEP/T-SSEP | Peripheral nerve action potential (N9/N8) Cervical cord dorsal horn potential (N13/N22) Subcortical potential (P14, N18/P31, N34)d Somatosensory cortical potential (N20/P37) | Traveling nearfield Stationary nearfield Stationary farfield Stationary nearfield |
TES-MEP/TMS-MEP | Corticospinal tract action potential (D wave, I waves) CMAP | Traveling nearfield Traveling nearfield |
ERP | Cortical electric potential | Stationary nearfield |
ECGe | Cardiac electric potential | Stationary farfieldd |
aNonsynaptic and non-neuronal mechanisms may play a role in the generation of EEG, MEG, ERPs, and cortical EPs
bThe micro/macro stereotype is not applicable since the microscopic signal (MFAP) is also the signal that is recorded.
cLate responses (F waves, H reflex, LL response, R1/R2 responses) are CMAPs that occur after the classic CMAP or M wave.
dNo definite consensus on the generator(s) of BAEP wave II and SSEP subcortical components and of FVEP waves I-VI
eECG is a traveling nearfield signal near its generator but behaves as a farfield stationary signal when recorded in CNP.
Note: For each CNP test or test modality (left column), the macroscopic signal recorded (middle column) and the spatiotemporal characteristics of the recorded signal (right column) are shown.
BAEP, brainstem auditory evoked potential; CMAP, compound motor action potential; EMG, electromyography; ERP, event-related potentials; MEG, magnetoencephalography; MFAP, muscle fiber action potential; M-SSEP, median nerve somatosensory evoked potentials; NCS, nerve conduction study; TES-MEP, transcranial electrical stimulation motor evoked potential; TMS-MEP, transcranial magnetic stimulation motor evoked potential; T-SSEP, tibial nerve somatosensory evoked potentials; VEP, visual evoked potentials.
10TABLE 1.5: Classification of Electrophysiologic Abnormalitiesa
Signal amplitude |
Abnormally low amplitude Loss of SGUs—e.g., low SNAP with loss of nerve fibers, EEG attenuation with loss of pyramidal cells Decrease in number of SGUs activated—e.g., low CMAP amplitude with loss of nerve fibers SGU asynchrony—e.g., low CMAP amplitude due to temporal dispersion of signals in motor nerve fibers Changes in volume conductor—e.g., edema increasing distance between generator and electrode Abnormally high amplitude Increase in number of SGUs activated—e.g., giant MUAP with muscle reinnervation of fibers Pathologic hypersynchrony of SGUs—e.g., high-amplitude epileptiform waves, giant N20 or P100 Decrease in impedance of conducting medium—e.g., accentuation of fast EEG waveforms with skull defects |
Signal conduction time |
Abnormally long latency Conduction slowing in peripheral nerve—e.g., prolonged SNAP, CMAP, SSEP, or P100 absolute latency Conduction slowing in central tract—e.g., increase in BAEP III-V and SSEP N13-N20 interpeak latencies, temporal dispersion and asynchronous activation of SGUs—usually only mild prolongation in latency Abnormally short latency Not easy to account for with pathology; a technical reason must be excluded—e.g., electrode placement or artifact |
Wave morphology or configuration |
Abnormal wave morphology Combination of abnormalities—e.g., wave amplitude, duration, contour, number of phases/turns Increase in wave duration and number of phases SGU desynchrony—e.g., long CMAP duration due to temporal dispersion of signals in motor nerve fibers Short wave duration and sharp contour SGU hypersynchrony—e.g., EEG and MEG epileptiform discharges, spikes, and sharp waves |
11Wave activation, modulation, and repetition |
Abnormal activation or recruitment Reduced SGU activation—e.g., decreased MUAP recruitment with motor neuron or nerve fiber pathology Increased SGU activation—e.g., increased MUAP recruitment due to muscle fiber pathology Reduced and increased SGU activation—e.g., release of latent slow rhythms with loss of pyramidal cell input Abnormal spontaneous activity Hyperexcitability of SGUs—e.g., fibrillation potentials, positive sharp waves, complex repetitive discharge Hyperexcitability of non-SGU circuit components—e.g., fasciculation potentials, myokymia Hyperexcitability of circuit—e.g., EEG or MEG epileptiform activity Abnormal repetition rate Perturbation in network dynamics—e.g., disorganization of EEG or MEG rhythms with encephalopathy |
Spatial characteristics |
Abnormal spatial distribution Often due to the same factors affecting the time-domain characteristics of waves Apparent displacement of signal generator Technical factors—e.g., improper electrode placement State change—e.g., drowsiness can manifest as “anterior displacement” of EEG alpha rhythm. |
Clinical and physiological context |
Physiological state Often due to the same factors affecting the time-domain characteristics of waves Age or developmental period Maturational—e.g., dysmature features in the neonatal EEG |
aWhen the above framework is used to classify abnormalities in the “raw” MEG record, one must be aware of its limits; for example, magnetic signals are not sensitive to changes in volume conductor properties. The above framework is not suitable for signals that are the product of complex transduction events between the biological source and the signal-sensing probe.
Note: Graphical features, clinical context, and pathophysiology are all taken into consideration in this classification scheme. Macroscopic abnormalities appear in the record as aberrations in amplitude, latency, or configuration of otherwise physiological waves, as a change in activation, modulation, or repetition pattern of physiologically recurring waves, as distortion of the spatial characteristics of waves, or as normally appearing but out-of-context waves in terms of physiologic state or age. Each of these abnormalities occurs because of impairment in SGU or non-SGU circuit components or because of changes in the properties of the volume conductor.
BAEP, brainstem auditory evoked potential; CMAP, compound motor action potential; MEG, magnetoencephalography; MUAP, motor unit action potential; SGU, signal-generating unit; SNAP, sensory nerve action potential; SSEP, somatosensory evoked potentials.
12SIGNAL RECORDING AND GRAPHICAL DISPLAY
The signal that must be recorded and analyzed, or the “signal of interest,” is often simply called “signal.” Any other signal contaminating and potentially distorting the signal of interest is considered “noise.” Noise entering the recording system may appear on the record as artifact. Because signal and noise are physically similar (both are electromagnetic signals), neurophysiologic instruments are intricately engineered to maximize the signal-to-noise ratio during signal recording and processing (25). Moreover, CNP technologists and specialists are trained to distinguish signal from artifact by visual inspection and, if necessary, digital reformation of the record. Chapter 2 is dedicated to instrumentation and signal processing. In this chapter, it is sufficient to mention that neurophysiological recording involves four processes: analog signal acquisition, analog signal conditioning, analog-to-digital conversion, and digital signal processing (Figure 1.1).
Signal analysis and interpretation is performed by professionals trained in CNP. Neurophysiologic data are usually presented and analyzed in the time domain (Figure 1.2). Recorded signals are displayed as waveforms on a computer or oscilloscopic screen (paper recording is nearly obsolete and is omitted from our discussion). CNP records contain one or more lines of tracing, called channels. Each channel is a graph of signal amplitude versus time, with waves rising above and falling below the baseline, indicating fluctuations in signal amplitude with time. When differential amplification is employed in biopotential recording, the wave amplitude at any given time is proportional to the voltage difference between the amplifier inputs, G1 and G2, multiplied by the amplification factor or gain (see Chapter 2) (25).
Quantitative analysis of “raw” data can extract additional information that can complement or supplement basic time-domain data. The fast Fourier transform (FFT) converts data in the time domain to an alternative representation of the same data in the frequency domain (26). Decomposing EEG into its frequency components and using compressed spectral array (CSA) to track the changes in amplitude/power of EEG frequency bands is a useful quantitative EEG (qEEG) technique for detecting cerebral ischemia and seizures during ICU-EEG monitoring (11). EEG trending in neonates is commonly performed using amplitude-integrated EEG (aEEG), a qEEG technique that involves filtering, rectification, smoothing, and compression of time-domain data. Quantitative analysis of MUAP, compound motor action potential (CMAP), HR-DB, and other data can also be performed. Although waveform analysis in the time domain is still the bread and butter of the clinical neurophysiologist, rapidly advancing computer technology and machine learning, and the increasing availability of automated algorithms, will inevitably transform the future practice of CNP.
Spontaneously generated signals represent oscillating physiologic processes, appear graphically as sinusoidal waves, and are referred to as rhythmic activity or rhythms (Figure 1.2A) (27). In EEG/MEG, sustained rhythmic activity is considered “background.” Background activity, not individual waves, serves as the framework for time-domain analysis. Additional waves and rhythms may appear spontaneously or with activation and are mixed with, or replace, the background. Both background activity and emergent waves are signals of interest that are analyzed collectively. The most important graphical descriptor of rhythmic activity is frequency, the number of wave repeats per second, which is reported as Hertz (Hz) or cycles per second (cps or c/s). Frequency is described in precise terms (e.g., 60 Hz) or in terms of frequency bands, including EEG/MEG: less than 4 Hz is delta, 4 to less than 8 Hz is theta, 8 to 13 Hz is alpha, and greater than 13 Hz is beta (28). By interpreting a wave as a basic unit of a rhythm, an isolated waveform can be assigned a frequency that is equal to the reciprocal of its duration.
FIGURE 1.1: Signal recording in clinical neurophysiology. The analog signal recorded includes the signal of interest and noise. Signal recording involves four general processes: (1) analog signal acquisition, (2) analog signal conditioning, (3) analog-to-digital conversion, and (4) digital signal processing. The end product of the recording process is a graphical representation or record of the signals (see Figure 1.2).
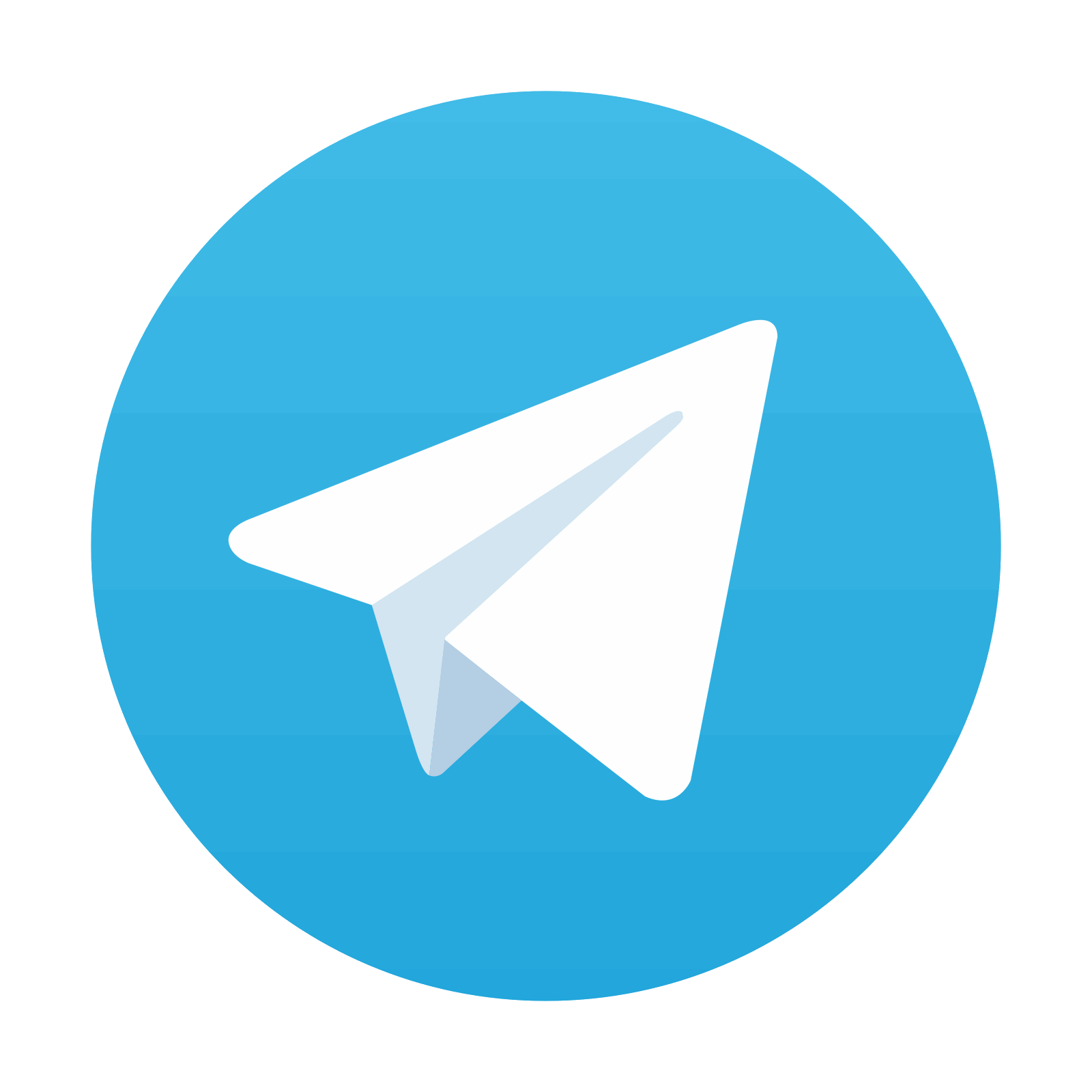
Stay updated, free articles. Join our Telegram channel

Full access? Get Clinical Tree
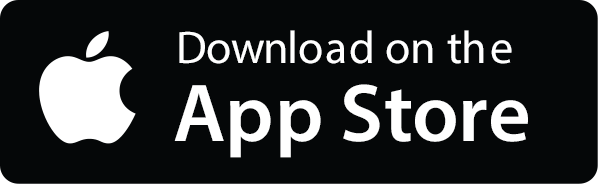
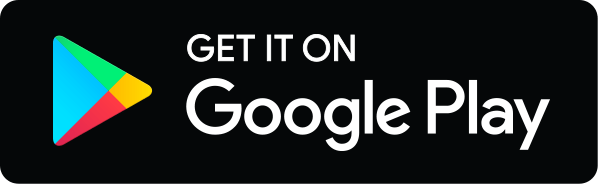