Fig. 16.1
Targeted drug development in neurodegenerative diseases
Once identified, candidate compounds are optimized for selectivity and affinity using in vitro models. Subsequently, testing in laboratory animals that model a particular disease is undertaken to establish therapeutic efficacy in vivo.
As a part of the drug development process it is necessary to establish the physicochemical properties of the candidate compound, including its chemical composition, stability, solubility, and the facility by which it can be consistently manufactured with a high degree of purity for use in the clinical trial setting. Preclinical toxicology studies on at least two animal species including one large animal specials is required, with chronic as well as acute dosing being evaluated. Pharmacokinetic studies are also critical, including studies of potentially active metabolites and investigations of potential drug-drug interactions. Regulatory requirements include demonstration that the drug candidate material can be manufactured, stored and delivered according to the highest specifications. The results of such studies provide the basis for submission of an Investigational New Drug (IND) or Clinical Trial Authorization (CTA) application to the appropriate regulatory authority to enable commencement of human clinical trials.
16.3.1 Cellular Based Models
Disease related pathways can be modelled in vitro using cultured cell lines with particular and relevant properties. For example immortalized HEK 293 cells, (derived from human embryonic kidney cells transformed with adenovirus) and the SHSY5Y (derived from human neuroblastoma cells) have many properties of immature neurons and are used for studies of gene expression and protein interactions. Other human cell lines including embryonic teratocarcinomas (NT2, hNT) and human H4 neuroglioma cell lines have been used to study protein misfolding and autophagy.
These immortalised cell lines although useful, are limited to modelling biological pathways that are variably important in both neuronal and non-neuronal structures. For more detailed analysis of specific neuronal functions (e.g. axonal transport), or to evaluate the properties of specific neuronal subpopulations, primary neuronal cultures developed from embryonic animals can be used. As there is now compelling evidence for glial involvement throughout the spectrum of neurodegenerative disease (See Chap. 1), systems that can model neuronal-glial interactions are used such as organotypic slices of brain or spinal cord. These cultures can be generated from both wild type and from transgenic animals expressing alterations in genes associated with neurodegenerative disease.
The advent of stem cell technology, particularly the development of induced pluripotent stem cells, has enabled development of cell lines derived directly from patients. Expansion and differentiation of iPS cells can generate large numbers of functional neurons in vitro, which can then be used to study the disease of the donating patient. iPS systems have been developed from patients with Alzheimer’s disease, Parkinson’s disease, amyotrophic lateral sclerosis, frontotemporal dementia, Huntington’s disease, spinal muscular atrophy and other neurodegenerative diseases. Advancements in reprogramming technology now enable investigators to study patient specific neurons that have been differentiated into specific cell types vulnerable to the disease being studied.
Interpretation the effects of a compound in vitro requires caution, as the information provided relates solely to modulation of specific targeted pathways. Translation to the more complex in vivo system is preferable prior to progression of a compound for late pre-clinical and early human studies. Indeed as most neurodegenerations are not cell autonomous, and are most likely due to disruptions at the level of large integrated networks, the extrapolation of findings to the infinitely more complex human system can only be undertaken with extreme caution.
16.3.2 In Vivo Models
Although in vitro models can provide detailed information regarding biochemical pathways, more complex integrated systems are also necessary to dissect the biological effects of candidate compounds. In neurodegenerative diseases, relatively simple organisms can provide an important resource to screen for in vivo modifiers of putative pathogenic processes. Simple organisms such as Drosophila melanogaster, Caenorhabditis elegans (C. elegans) and zebrafish have been developed as useful models for AD, PD, ALS, and Huntington’s disease. For example, an eye-specific overexpression of human Tau has been developed in Drosophila melanogaster that has identified signalling pathways that are dysregulated in models of proteinopathies. Similarly, a range of informative C. elegans models of neurodegeneration have been developed manifesting abnormal behavioural or pathological phenotypes that partially recapitulate cellular, molecular and pathological aspects of several distinct human neurodegenerative processes. Zebrafish are useful as vertebrate models. Because they are suitable for large scale phenotypic screening, zebrafish have been used for mutagenesis studies, facilitating both selection of possible new targets, in addition to target validation and large scale drug screening.
Analysis of larger and more complex organisms are also required for pre-clinical drug development. Genetically modified animals with uniform genetic background can provide standardised controlled environments that permit molecular analysis of neurodegenerative pathways.
Transgenic rodents have been developed using human pathogenic mutations including the SOD1 mouse for ALS; AD mouse models including human mutations in amyloid precursor protein (APP) presenilin and tau; PD models including mutations in LRRK2 and HD models containing trinucleotide repeat expansions in huntingtin. To a greater or lesser extent, these models can be shown to imitate diverse neurodegeneration related pathologies, and are used for both basic and therapeutic investigations. A very wide variety of preclinical trials of potential therapeutic agents have been undertaken using these models including over 140 substances in AD between 2001 and 2011, and up to 250 in ALS during the same period.
However, translation of effective therapies in transgenic mouse models to human neurodegenerative disease has been singularly unsuccessful to date. Likely reasons for this failure include issues related to drug availability, lack of information regarding specific targets, and lack of pharmacodynamics markers in either models or humans that reflect adequate target engagement.
16.4 Clinical Phase of Drug Discovery
Following successful completion of the requirements for Investigative New Drug (IND) in the US or a Clinical Trial Authorisation (CTA) in Europe, clinical trials of a lead compound can be initiated.
Clinical trials have been traditionally divided into four main phases i.e. phases I–IV (see Table 16.1). However, Phase 0 first in human microdosing studies may also be undertaken as a means to identify promising candidates for further evaluation in Phase I–II trials. In Phase 0 trials, a small number of healthy volunteers are treated with single sub-therapeutic doses to ensure that pharmacokinetics of the drug in humans recapitulates those of the pre-clinical studies.
Table 16.1
Standard phases for clinical trials
Clinical trials |
---|
Phase 1: safety (controls- unaffected by disease) |
Phase 2: proof of concept (small numbers with disease) |
Phase 3: pivotal (large numbers, required for regulatory approval) |
Phase 4: post marketing |
Phase 1
Phase 1 studies are conducted either in healthy individuals or in patient populations for whom the drug is being developed. The purpose is to evaluate safety, tolerability, pharmacokinetics and pharmacodynamics, and identification of side effects of the new compound in humans. Depending on the delivery method, a small placebo group may be included to provide information about adverse events not specific to the therapeutic agent. In general, small numbers of patients are exposed to single or multiple doses of the experimental agent, with small doses evaluated first and doses escalating toward a maximum tolerated dose. More recently, the continuous reassessment method (CRM) has been developed, which assigns patient dose levels according to a Bayesian statistical model. CRM models have the dual advantages of potentially reducing the number of patients exposed to drug in Phase 1, and ensuring that patients are dosed at levels more likely to be clinically relevant.
Phase 2
Phase 2 studies are usually placebo controlled and doubled blinded and are conducted in the target patient population. The purpose is dose finding, tolerability and early evidence of activity. Phase 2 trials can also be undertaken to search for better outcomes or to test biomarkers that can be used for more pivotal Phase 3 studies. While clinical efficacy is evaluated, a primary focus of Phase 2 trials is most often on assessment of pharmacodynamic markers. A pharmacodynamic marker is usually an objective measure of a drug’s ability to engage a specific target and to modulate it in some way. Thus, a pharmacodynamic marker may be a change in gene activation profile, evidence of up or down regulation of an enzymatic pathway, or excitation or blocking of a specific receptor. Such markers may not have a direct impact on the disease state being studied; determining whether such a relationship exists is a question for phase 3. Phase 2 studies can be sometimes divided into Phase 2A and Phase 2B, with the former designed for dosing and the latter for PD activity. The majority of new therapeutic agents fail at the Phase 2 stage.
Phase 3
This is the pivotal phase of a new drug and is designed to prove efficacy. The design is double blinded and placebo controlled and takes place in a number of different centres. The drug is administered to larger groups of people to confirm effectiveness and characterize side effects. Comparisons may be against placebo, or best available care. For example, in ALS, the only drug proven to affect disease progression is Riluzole, so that most studies compare patients taking riluzole plus the new agent versus patients taking riluzole alone. Phase 3 trials are generally expensive, costing upwards of €60 million. The outcome of Phase 3 trials are presented to the regulatory authorities for approval of the compound as a new therapeutic agent.
Phase 4
This phase is undertaken post-marketing to generate detailed datasets relating to the effect of the drug on different populations, and to collect side effects following longer term use.
16.5 Clinical Trial Design
Clinical trials are designed with a primary end point, which most often is a measure of drug safety in phase 1, pharmacodynamic activity in phase 2, and efficacy in phase 3. The number of patients recruited is based on power calculations, in which the expected effect of the drug is estimated and the number of patients needed to be treated accordingly calculated to reach a statistically significant threshold. The duration of the trial is generally pre-defined, and following completion of assessment of the final enrolled patient, the database is locked for analysis.
As clinical trials are extremely expensive, and patient recruitment can be challenging, a number of different types of trial design have been proposed, primarily for phase 2 studies (Table 16.2). The goal of a Phase 2 study is not necessarily to produce conclusive evidence of efficacy, and a suggestion of drug activity may be sufficient for a sponsor or investigator to decide that further studies are warranted.
Table 16.2
Different types of trial design, aimed at reducing required sample size
Trial designs |
---|
Futility |
Lead in |
Adaptive |
Sequential |
Combined assessment of function and survival (CAFS) (In Amyotrophic Lateral Sclerosis) |
Futility Design
One possible approach is to apply what is called a “futility design”. Rather than stating that the goal of the study is to show evidence of efficacy or pharmacodynamic activity, the purpose of a futility study is to determine whether it is worthwhile going forward. It may be very important to determine whether further investigation of a drug would be considered unwise either for clear lack of efficacy or for safety. For example, if all treated subjects did worse on all measures than all placebo subjects in a small study, it would be unlikely that the drug would be efficacious if more subjects were studied; ie futility would have been demonstrated. Futility designs require that the traditional null hypothesis used in statistical comparisons be changed; the null hypothesis in a futility study is that it is not futile to go forward. If the null hypothesis is rejected, futility is concluded. Demonstration of non futility requires far fewer subjects than demonstration of efficacy; however, depending on the measure used, non futility may be demonstrated even when two comparison groups are identical.
16.5.1 Pick the Winner Design
Another approach is to use what has been called a “pick the winner” design’ to make decisions regarding which drug or dose level should be progressed to a phase 3 study. Such designs may or may not involve a placebo group. Some compare several groups that may either be given different doses of the same drug or different drugs over a defined time period. At the end of the study, the best performing group is declared the “winner,” and further studies are then performed using that drug or dose and comparing it to placebo in a phase 3 study. The potential advantages of such a design are that many doses or drugs can be tested concurrently; however, there are several limitations. First, there will always be a winner, so that even if chance dictates the best performing group, going forward with further testing will always be the recommended outcome. Second, especially if multiple drugs are compared together, it is possible that in fact more than one active compound is among the agents tested. In that situation, only one drug will be chosen for further testing even though two or more are active and potentially important. A pick the winner design was recently employed in a phase II clinical trial of coenzyme Q in ALS
16.5.2 Crossover Design
Lead-in or crossover designs have also been suggested for phase 2 studies. Crossover studies have not been deemed appropriate for disease modification, as ALS is a progressive disease with clinical features that are likely to vary over time. Thus, a therapeutic agent that is neuroprotective is likely to have a more beneficial effect if given early in the disease than late, so that the effects of therapy after a crossover may not be the same as the effect noted before the crossover. However, for drugs that may exert a symptomatic benefit, crossover designs can be very efficient. Recent crossover studies in ALS include evaluations of nuedexta for pseudbulbar affect and bulbar function, as well as a phase 2A study of tirasemtiv to improve motor function.
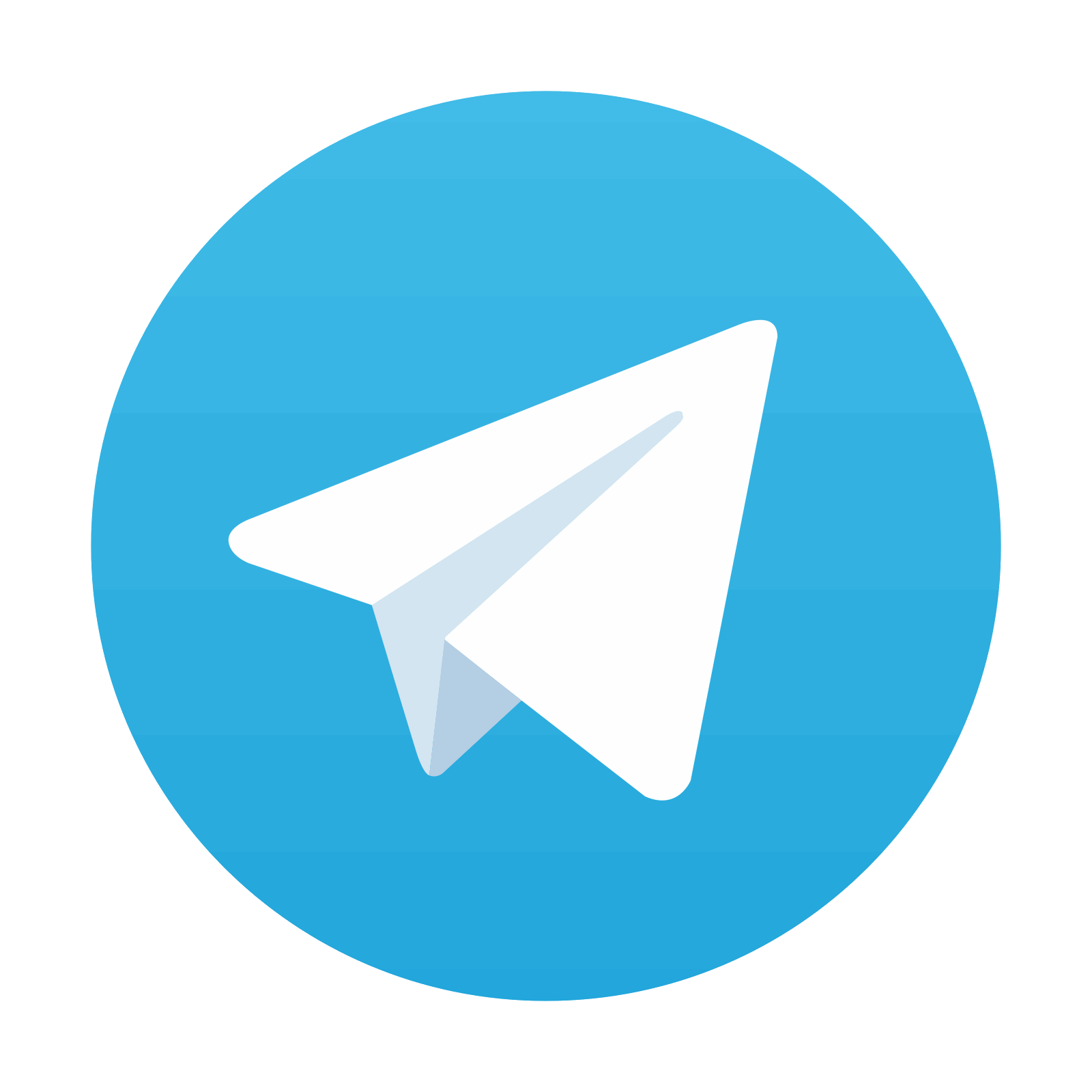
Stay updated, free articles. Join our Telegram channel

Full access? Get Clinical Tree
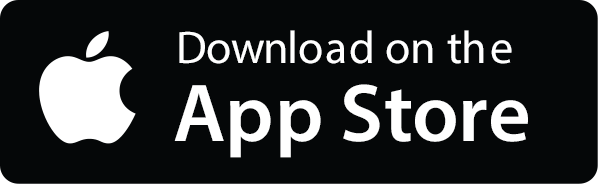
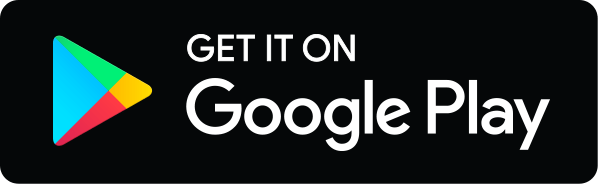