Fig. 1.1
Clinical Classification of Neurodegenerative conditions
Eponymous classifications (e.g., Alzheimer disease, Parkinson disease, and Huntington disease) remain useful in a clinical setting, as the diagnosis generates a framework for clinical discussion, prognostication, and disease management.
However, it is increasingly recognized that neurodegenerative diseases can also be subdivided into categories based on pathological or genetic characteristics, as outlined in Fig. 1.1, and as the field advances, diagnostic categories for some diseases will accordingly require some adjustment.
A number of common themes have emerged in the pathogenesis of various neurodegenerative diseases. Whether these commonalities are simply secondary processes, which reflect the fact that neurons have a limited repertoire by which to die, or whether they reveal important initiating upstream mechanisms remains unclear.
In the following section, some putative common pathogenic molecular mechanisms will be discussed, but it is worth bearing in mind that it is not currently possible to distinguish between mechanisms that initiate disease and those that contribute to disease progression. Both may yield targets suitable for therapeutic intervention, but only the former can yield knowledge that will lead to disease prevention.
1.4 Common Themes in Genetics
Familial aggregation of specific neurodegenerative diseases is well recognized, with both autosomal dominant and autosomal recessive inheritance observed. Excepting the trinucleotide repeat disorders, which exhibit Mendelian inheritance often with full penetrance and anticipation, neurodegenerative disorders have a small percentage of familial cases and a large percentage of apparently sporadic cases. Sporadic and familial cases are usually phenotypically and histologically indistinguishable, although the onset of familial cases tends be earlier. This suggests that genetic mutations accelerate the molecular processes that lead to late-onset sporadic disease.
A number of causative genes have been discovered for specific neurodegenerative diseases. An understanding of gene function has helped to elaborate mechanisms of disease, as well as providing a platform for research into similar mechanisms in other neurodegenerative diseases. For example, mutations in APP, Presenilin 1, and Presenilin 2, which occur in early onset alzheimer disease (AD), and ADAM10 in late-onset AD, cause altered protein production and increased aggregation of β-amyloid protein (Aβ). Similarly, mutation of α-synuclein leads to aggregation of the protein in Lewy bodies, a feature also seen in the most common cause of inherited PD, caused by mutation of LRRK2. Mutations in genes associated with oxidative stress pathways, SOD1 and DJ1, have been implicated in familial amyotrophic lateral sclerosis (ALS) and PD, respectively. The discovery of TARBDP, FUS and an intronic repeat in C9ORF72 in both ALS and frontotemporal dementia (FTD)-ALS has established a role for RNA dysfunction in several neurodegenerative diseases.
Genome-wide association studies and high-throughput sequencing have identified susceptibility genes in many neurodegenerative conditions. Moreover, overlap between susceptibility genes has been reported. APOE4 is well established as a risk factor for early and late-onset AD. However, meta-analysis has shown that presence of the allele is also linked to PD and FTD. It has also been shown that the number of APOE4 alleles correlates with age of disease onset. In addition, genes that cause neurodegenerative disease can also act as susceptibility factors in others. For example, more than 34 CAG repeats in the ataxin gene 2 are known to cause spinocerebellar ataxia type 2 (SCA2), whilst an intermediate number of repeats (27–33) have been associated with an increased risk for ALS.
1.5 Common Themes in Neuropathology
Thorough pathological diagnosis depends on the pathologist having access to accurate clinical information as well as tissue analysis. Pathological diagnostic criteria presume that phenomena involved in degeneration tend to occur together, but invariably they are evident at different time points during the disease. Thus, interpretation of the neuropathological data must take into account when in the clinical course of the disease the pathological assessment has been performed.
Both gross and microscopic tissue examination can help to identify pathological processes and can also differentiate features that are purely degenerative, and those that emerge from the innate responses to protect and repair. Finding the site of the earliest visible alteration helps in establishing the diagnosis. For example, degeneration in the hippocampal and frontal lobe pyramidal neurons is associated with AD, in the dopaminergic neurons of the substantia nigra with PD, in the upper and lower motor neurons of the pyramidal system with ALS and in the medium-sized spiny GABAergic neurons of the striatum with HD.
Dementia coupled with mainly limbic atrophy suggests Alzheimer disease (AD), while mild atrophy implies Lewy body disease. Moderate cognition decline in the setting of asymmetric, motor and sensory impairment, with reduced metabolic activities, and atrophy prevailing around the central sulcus is indicative of corticobasal degeneration. However, if these changes involve the lateral half of the putamen, caudal to the mammillary body, multiple system atrophy (MSA) is the most likely diagnosis. Despite the topographical differences in neurodegenerative diseases, gross histopathological findings are similar – there is regional atrophy with gliosis and neuronal loss as well as abnormal accumulation of protein.
A large subset of neurodegenerative diseases display protein aggregates. Ubiquitinated neuronal nuclear inclusions occur in polyglutaminopathies including Huntington disease (HD). Either parenchymal or vascular accumulation of Ab occurs with aging, as well as in the occurrence of AD in children with Down syndrome. Neostriatal large neurons are rather resistant compared to medium size neurons in HD, but they degenerate in progressive supranuclear palsy and in AD. Loss of spinal motor neurons is typical in amyotrophic lateral sclerosis (ALS), whereas glial cells degenerate in MSA. TDP-43-positive neuronal cytoplasmic inclusions, originally thought to be characteristic of ALS and subsequently FTD, are also detected in AD, PD HD and SCA2, though the location of the inclusions varies with disease. Lewy bodies are a hallmark of Parkinson disease with or without dementia and involve many classes of neurons.
In summary, the three practical steps that are useful while appraising the pathologic phenotypes of neurodegenerative diseases are:
1.
Identifying the sites or systems where the brunt of the tissue loss occurs, which might be revealed on neuroimaging at some time points during the disease, or eventually on post-mortem examination. However, also consider other regions which may allow subclassification of the disease, such as the characteristic extra motor pathology in the hippocampus, associated specifically with C9ORF72 repeat expansion.
2.
Cataloging the cells undergoing degeneration.
3.
Identifying the abnormal aggregates, their cellular (neuronal vs. glial cells) and topographic propensities (extracellular, cytoplasmic, or nuclear).
General experience confirms that these steps are crucial for assigning the most appropriate diagnosis to most of the currently classifiable neurodegenerative diseases.
1.6 Selective Vulnerabilities
Cells are constantly placed under stress because of intrinsic metabolic processes and also because of a number of extrinsic factors. Neurons are even more vulnerable as they facilitate neurotransmission and maintain the metabolic needs required for long axonal projections. Larger neurons with myelinated axons extending long distances appear to be most vulnerable. These neurons have high energy requirements, are especially reliant on axonal transport, and have a larger surface area for exposure to environmental toxins. Coupled with the fact that once damaged they cannot regenerate, neurons in general represent a vulnerable group of cells.
Why are certain subgroups of neurons more susceptible than others?
A number of hypotheses have been proposed to account for specific selective susceptibility of certain neurons to specific pathological processes (Table 1.1). Neuroblasts arise from the neuroectoderm at 8 weeks of fetal life and then proceed to differentiate into highly specialized neurons. Even though all neurons of an individual have the same genes, it is the gene expression profile that determines the highly specialized function of a specific neuron. This degree of specialization is thought to render individual neurons more susceptible to anoxia and oxidative stress, and factors that lead to selective vulnerability include the type of neuron and the neuronal microenvironment.
Table. 1.1
Neurologic diseases and selectively vulnerable cells
Disease | Vulnerable neuron |
---|---|
Parkinson disease | Dopaminergic neurons |
Alzheimer disease | Cholinergic neurons |
Amyotrophic lateral sclerosis | Upper and lower motor neurons |
Frontotemporal dementia | Frontotemporal cortical neurons |
Huntington disease | GABAinergic neurons |
The dopaminergic neurons located in the substantia nigra in PD are particularly prone to reactive oxygen species (ROS)-induced injury. This selective vulnerability is believed to derive from the fact that neurons in the substantia nigra have very high levels of iron and copper, both of which are capable of catalysing ROS formation. Additional evidence suggests that the substantia nigra may have low stores of antioxidant molecules such as glutathione, thereby increasing neuronal susceptibility to the damaging effects of ROS. However, this does not explain the vulnerability of neurons associated with PD in other regions of the brain such as autonomic ganglia, brainstem, and spinal cord.
In ALS, motor neurons are affected primarily, although it is now recognized that non-motor neurons are involved in cognitive dysfunction. Motor neurons are large cells and often have long axonal fibers. In lower motor neurons, these fibers may stretch over a meter in order to supply distal muscles. They require a strong cytoskeleton, neurofilament network, and efficient axonal transport system. Motor neurons are highly metabolic and exquisitely sensitive to energy demands. Any one or a multitude of these factors makes motor neurons vulnerable. As is the case in the substantia nigra, deficiencies of protective agents such as glutathione and cytosolic calcium-binding proteins can add to neuronal stress.
The different phenotypic characteristics of individual diseases remain difficult to explain. In Mendelian diseases, differences can be evident within families with the same mutation. For example, a proband with familial ALS may present with flail arm and slow progression, while another family member may present with rapidly progressive bulbar onset disease. Similarly, some patients with AD may have executive or visuospatial deficits, while others have an amnestic syndrome. In kindreds with triplication of APP, the clinical disease segregates into two distinct phenotypes: those with classical AD indistinguishable from idiopathic AD and those with vascular dementia and a stroke-like syndrome. The causes of these phenotypic variations remain to be determined.
1.7 Aberrant Protein Structure
The formation of aberrant structures by more than 20 different proteins appears to underlie a large disease group, many of which afflict the CNS (Table 1.2). Indeed, deposits of protein aggregates are histological hallmarks of many neurodegenerative diseases and consequently, the proteins involved and the mechanism of their aggregation is under intense scrutiny.
Table. 1.2
Neurologic diseases associated with aberrant protein structure
Disease | Protein deposited | Site of deposition |
---|---|---|
Parkinson disease | a-synuclein | Intracellular (Lewy neuritis and Lewy bodies) |
Multiple systems atrophy | a-synuclein | Intracellular argyrophilic inclusions in both oligodendroglia and neurons |
Hereditary cerebral amyloid angiopathy | Cystatin C | Extracellular |
Congophilic amyloid angiopathy | b-amyloid | Extracellular |
Alzheimer disease | b-amyloid | Extracellular (amyloid plaques) |
Alzheimer disease | Tau | Intracellular (paired helical filaments) |
Frontotemporal dementia | Tau | Intracellular inclusions (paired helical filaments and Pick bodies) |
Frontotemporal dementia | TDP43-ubiquitin | Cytoplasmic inclusions |
Familial British dementia | ABri | Extracellular (amyloid plaques) |
Familial British dementia | ADan | Extracellular (amyloid plaques) |
Transmissible spongiform encephalopathies | Prion protein | Extracellular amyloid plaques and/or diffuse deposits |
Amyotrophic lateral sclerosis | TDP43-ubiquitin | Cytoplasmic inclusions and ubiquitin-positive neuronal threads |
Huntington disease | Mutant huntingtin | Nuclear and cytoplasmic inclusions |
Inherited spinocerebellar ataxias | Various proteins with polyglutamine expansions | Nuclear and cytoplasmic inclusions |
The mechanism by which the attainment of an aberrant protein structure causes disease is still unclear and may involve both the loss of a vital physiological function, and the acquisition of toxic properties.
While loss of function can be harmful, toxic gain of function is invariably pathogenic. Toxicity may be direct or indirect. For instance, an aberrant protein structure might bind to a specific receptor directly, causing an inappropriate activation of a cascade that initiates cellular changes, which in turn lead to compromise of cellular function. Alternatively, aberrant structures might acquire properties that allow them to interact with and destabilize cellular membranes or other proteins, thus causing a secondary toxicity. Moreover, accumulation of aberrant proteins may strain the normal mechanisms responsible for controlling protein folding and degradation, resulting in a generalized loss of protein homeostasis and consequent toxicity. In the cases where there are large repeat expansions within the non-coding region of the gene, these can act as templates for repeat associated non-ATG (RAN) translation, generating aberrant repeat peptides which also accumulate in the cell.
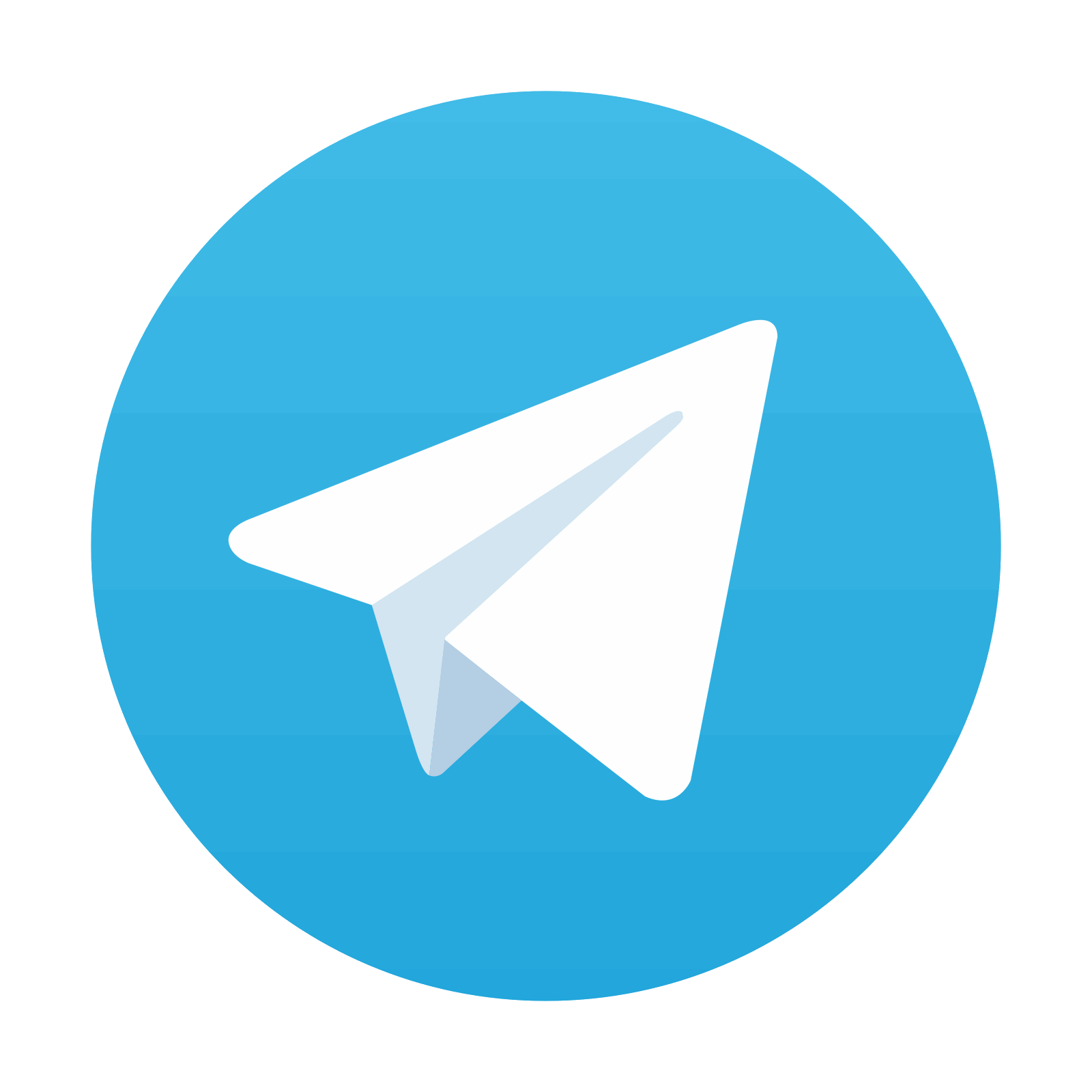
Stay updated, free articles. Join our Telegram channel

Full access? Get Clinical Tree
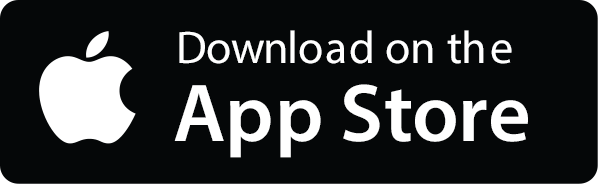
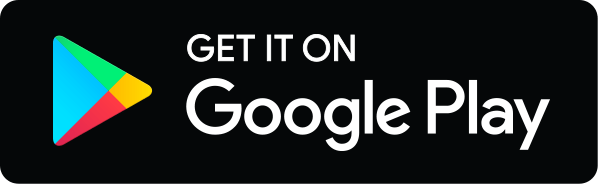